Bacteria and Bioremediation: How Microbes Are Used to Detoxify Oil Spills and Waste
Table of Contents
I. Introduction
The rising levels of environmental pollution, mainly from oil spills and the wrong handling of industrial waste, clearly highlight the urgent need for creating and using effective cleaning strategies. Bacteria, often seen just as germs causing diseases, actually have an important role in cleaning up contaminated areas. These tiny organisms can break down harmful materials and complex chemicals into safe byproducts that do not harm the environment. The cleanup process is complex and includes different methods, like bioaugmentation and biostimulation, which help boost the natural abilities of bacteria to break down pollutants. It is important to understand these techniques to create new solutions for pollution in various settings. The success of bioremediation is further shown in many scientific models that describe how microbes interact with pollutants. One of these models, presented in [citeX], explains the specific roles of different microbes in cleaning up the environment. This detailed study not only shows how crucial bacteria are in fighting pollution but also opens doors for future research and practical uses in restoring ecosystems. Thus, utilizing the power of these microorganisms is essential for creating cleaner ecosystems and ensuring the Earth’s sustainability for future generations.
Aspect | Description | Examples |
---|---|---|
Definition | The use of microorganisms, fungi, plants, or enzymes to break down and remove pollutants from the environment. | Oil spill cleanup using bacteria. |
Types of Bioremediation | Includes in situ (on-site) and ex situ (off-site) methods to treat contaminated areas. | Bioventing (in situ), biopiles (ex situ). |
Key Microorganisms | Bacteria, fungi, and archaea that degrade pollutants into non-toxic compounds. | Pseudomonas, Bacillus, Mycobacterium. |
Biodegradable Pollutants | Organic compounds that microorganisms can naturally break down. | Hydrocarbons, pesticides, sewage waste. |
Non-Biodegradable Pollutants | Some heavy metals and synthetic chemicals require enhanced bioremediation strategies. | Lead, mercury, plastic waste. |
Factors Affecting Bioremediation | Oxygen levels, pH, temperature, nutrient availability, and microbial population. | Optimal conditions vary by pollutant type. |
Advantages | Environmentally friendly, cost-effective, and less disruptive than traditional cleanup methods. | Naturally restores ecosystems. |
Limitations | Slow process, may be ineffective for highly toxic or complex contaminants. | Not suitable for all types of pollution. |
Applications | Used in soil, water, and air pollution control, industrial waste management, and disaster recovery. | Oil spill cleanup, groundwater purification. |
Future Prospects | Genetic engineering and synthetic biology approaches to enhance microbial degradation capabilities. | CRISPR-modified bacteria for faster pollution breakdown. |
A. Definition of Bioremediation
Bioremediation is when living things, mainly tiny organisms like bacteria and fungi, are used to clean up polluted places, especially from oil spills and dangerous waste. This natural process uses the breakdown abilities and enzymes of these organisms to turn harmful pollutants into less harmful or safe substances, aiding in the recovery of damaged environments. Various factors can affect how well bioremediation works, such as which microorganisms are there, environmental conditions like temperature and pH, and the specific types of pollutants. For instance, as shown in [citeX], bacteria, yeast, and microalgae play important roles in improving bioremediation through techniques like natural attenuation, biostimulation, and bioaugmentation. These techniques focus on boosting the growth of existing microbes, adding extra microbes that can break down certain pollutants, and improving conditions to help microbial activity. Knowing what bioremediation is and how it works gives important insight into how it can be applied, highlighting the potential of microbial methods in tackling the serious issue of environmental pollution. As society tries to deal with the harmful effects of industrialization and urban growth, bioremediation stands out as a sustainable and efficient alternative to usual cleanup methods, helping protect the environment and public health.
Aspect | Description |
---|---|
Definition | The use of biological organisms to break down, neutralize, or remove contaminants from the environment. |
Key Organisms | Bacteria, fungi, algae, and plants capable of degrading pollutants. |
Main Purpose | To restore polluted environments by naturally decomposing hazardous substances. |
Types | In situ (on-site) and ex situ (off-site) bioremediation. |
Mechanism | Microorganisms metabolize toxic compounds, converting them into harmless byproducts like water and carbon dioxide. |
Pollutants Treated | Organic pollutants (oil spills, pesticides) and inorganic contaminants (heavy metals, radioactive waste). |
Environmental Impact | Eco-friendly, sustainable, and cost-effective alternative to chemical or mechanical cleanup methods. |
Challenges | Requires optimal conditions (temperature, pH, oxygen, nutrients) and may be slow for certain pollutants. |
Applications | Soil and groundwater decontamination, oil spill cleanup, wastewater treatment, industrial waste management. |
Future Developments | Use of genetically engineered microbes to enhance bioremediation efficiency. |
B. Importance of addressing oil spills and waste
Dealing with oil spills and waste is important for keeping the environment safe, public health intact, and the economy stable. Oil spills can harm marine life, causing long-term damage to plants and animals, and negatively impacting biodiversity and habitats. These harmful effects can spread through the food chain, affecting not just the local ecosystem but also the income of communities that depend on fishing and other ocean resources. In addition, the poisonous materials in spilled oil can endanger nearby residents’ health, often leading to water contamination that jeopardizes drinking water and food supplies. Polluted water may cause health problems like stomach illnesses and long-term issues due to chemical exposure, making recovery in affected regions more difficult. The economic effects of oil spills are also significant, as businesses that rely on clean water—like fishing, tourism, and leisure activities—suffer from pollution. The loss of income and jobs can create a serious cycle of economic downturn in these areas. Good management and cleanup methods are crucial for reducing these impacts, especially using bioremediation techniques that involve bacteria to break down toxic substances. By leveraging natural microbial processes, we can speed up the recovery of affected ecosystems, encouraging a healthier relationship between human actions and nature. This highlights the need for thorough strategies to tackle the complex issues related to oil spills and waste. Putting in place preventative actions and response plans helps ensure the protection of our natural resources and maintains both public health and economic strength over time.
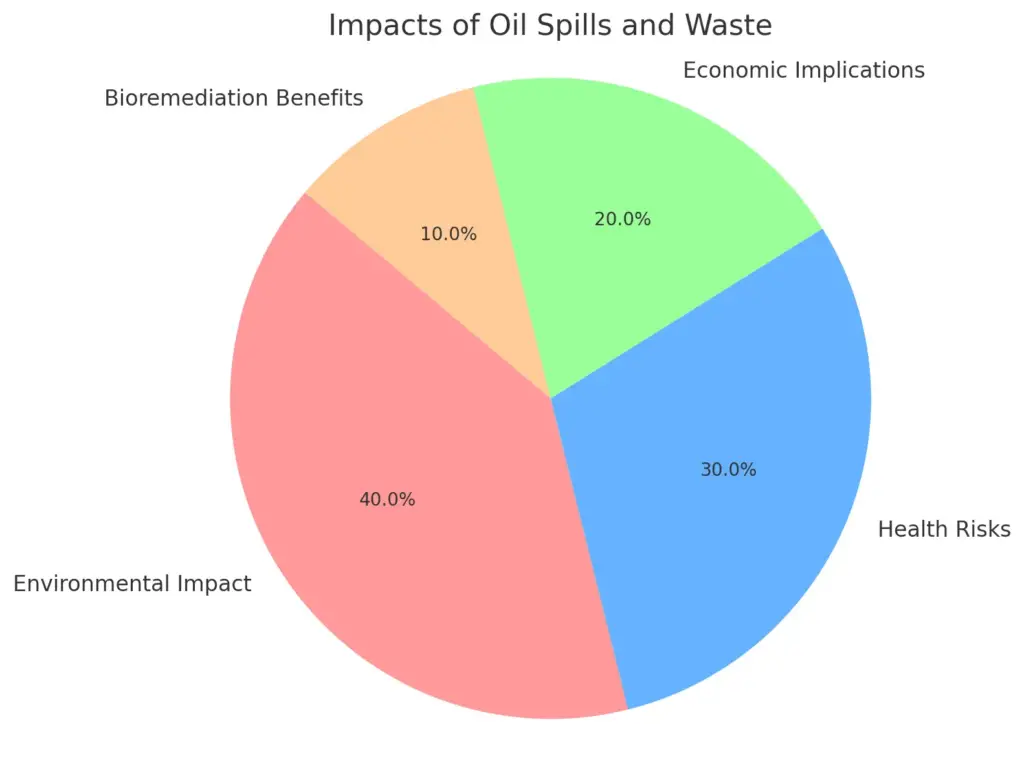
This pie chart illustrates the importance of addressing oil spills and waste by representing the various impacts, with the largest portion highlighting environmental damage, followed by health risks and economic implications, and a smaller slice for the benefits of bioremediation.
C. Overview of the role of bacteria in bioremediation
Bacteria have a crucial role in bioremediation, acting as important agents in cleaning up contaminated areas, especially with oil spills and hazardous waste. These tiny organisms can break down complex organic compounds, turning harmful substances into safer or non-toxic versions through their metabolic activities. For example, some bacterial strains can break down hydrocarbons from crude oil, reducing the environmental damage caused by spills. This ability not only decreases pollution but also improves the quality of soil and water, leading to healthier ecosystems. By harnessing these natural bacterial processes, we can speed up the cleaning of contaminated sites, making the restoration process more effective. Additionally, the diagram showing bacteria interacting with crude oil illustrates how these microorganisms, by producing biosurfactants, help emulsify and break down oil pollutants. This visual shows their important role in bioremediation strategies, highlighting how bacteria serve as both catalysts and agents of change in environmental issues. The chemical processes used by bacteria can be applied to improve bioremediation efforts, customizing methods for specific pollutants. This relationship between bacteria and contaminants highlights the importance of using these microorganisms to create effective environmental cleanup methods. By grasping the important roles that bacteria have, researchers and environmental professionals can create bioremediation techniques that are not only effective but also sustainable, ensuring a healthier world for future generations.
TABLE – Overview of the Role of Bacteria in Bioremediation
Aspect | Description | Examples |
---|---|---|
Role in Bioremediation | Bacteria break down or transform pollutants into non-toxic substances using metabolic processes. | Pseudomonas species degrade oil spills. |
Mechanisms Used | Bacteria use aerobic or anaerobic respiration to degrade contaminants. | Aerobic: Bacillus; Anaerobic: Dehalococcoides. |
Types of Pollutants Treated | Organic compounds, hydrocarbons, heavy metals, and industrial waste. | Oil, pesticides, plastics, mercury, lead. |
Aerobic Bioremediation | Uses oxygen to degrade pollutants into simpler compounds like CO₂ and water. | Pseudomonas putida breaks down benzene. |
Anaerobic Bioremediation | Occurs in oxygen-deficient environments where bacteria use alternative electron acceptors. | Dehalococcoides removes chlorinated solvents. |
Biodegradation Pathways | Bacteria enzymatically break down pollutants through oxidation, reduction, or hydrolysis. | Mycobacterium degrades petroleum hydrocarbons. |
Bioaugmentation | Addition of specific bacterial strains to enhance pollutant degradation. | Alcanivorax borkumensis for oil spills. |
Biostimulation | Enhancing natural bacterial activity by adding nutrients or oxygen. | Adding nitrate to stimulate hydrocarbon-degrading bacteria. |
Heavy Metal Bioremediation | Some bacteria reduce or immobilize toxic metals, making them less harmful. | Cupriavidus metallidurans detoxifies heavy metals. |
Future Potential | Genetic engineering and synthetic biology may enhance bacterial bioremediation capabilities. | CRISPR-modified bacteria for faster degradation. |
II. Mechanisms of Bioremediation
Bioremediation works through different microbial processes that change or break down pollutants in polluted areas, which is important for restoring nature’s balance. Bacteria, fungi, and algae, being some of the most adaptable organisms, are key in this as they use various enzymes to dismantle complex hydrocarbons usually found in oil spills and other pollutants. One main approach in bioremediation is bioaugmentation, where specific groups of microbes are added to a polluted location to help break down harmful substances more effectively. On the other hand, biostimulation involves adding certain nutrients to improve the activity of existing local microbes and promote their natural degradation processes. These methods do more than just speed up degradation; they also help change toxic substances into less harmful ones, thus reducing risks. Moreover, changes like modifying cell porosity and metabolic pathways enable microbes to better reach and degrade contaminants. This adaptability highlights the detailed interactions among microbes that aid in bioremediation. Grasping these complex processes is crucial for creating effective bioremediation methods that can be customized for different environments and pollutants, leading to successful cleanup and ecosystem recovery. This understanding also helps advance sustainable environmental management, ensuring healthier ecosystems in the future.
Mechanism | Description | Examples |
---|---|---|
Microbial Degradation | Bacteria break down pollutants into non-toxic byproducts using metabolic processes. | Pseudomonas putida degrades hydrocarbons. |
Bioaugmentation | Introduction of specific microbial strains to accelerate biodegradation in contaminated sites. | Alcanivorax borkumensis for oil spill cleanup. |
Biostimulation | Enhancing the growth of native microbes by adding nutrients or oxygen. | Adding nitrogen and phosphorus to boost bacterial activity in soil. |
Phytoremediation | Using plants to absorb, accumulate, or break down contaminants in soil and water. | Sunflowers for lead removal from contaminated sites. |
Mycoremediation | Fungi break down toxic compounds through enzymatic activity. | Pleurotus ostreatus (oyster mushrooms) degrade petroleum hydrocarbons. |
Cometabolism | Microbes degrade pollutants using enzymes meant for other metabolic processes. | Methanotrophs degrade trichloroethylene (TCE) while metabolizing methane. |
Reductive Dechlorination | Anaerobic bacteria remove chlorine atoms from chlorinated compounds, making them less toxic. | Dehalococcoides species degrade tetrachloroethylene (PCE). |
Biosorption | Bacteria or fungi absorb and bind heavy metals, reducing their toxicity. | Bacillus and Pseudomonas species accumulate cadmium and lead. |
Bioleaching | Microbes extract metals from ores or contaminated environments through oxidation. | Acidithiobacillus ferrooxidans recovers copper from mining waste. |
Electro-Bioremediation | Uses electrical currents to stimulate microbial activity and enhance pollutant breakdown. | Used in treating petroleum-contaminated soils. |
A. Natural attenuation processes
Natural attenuation processes are important methods in cleaning up contaminated places, especially during oil spills and other hazardous waste events. This type of cleanup relies on the natural ability of microorganisms in the environment to break down, change, or contain pollutants without needing outside help, which makes it a very sustainable way to restore the environment. Major factors that affect natural attenuation include the variety of microbes present, the availability of nutrients, and environmental conditions like pH and temperature. Each of these factors plays a big role in how well this process works. Microbial variety ensures that there are different ways to break down various pollutants, while nutrient availability gives the needed food for these microorganisms to grow. Environmental factors like pH and temperature can influence how active and fast-growing these microbes are, which can either help or hinder the overall breakdown of contaminants. Together, these important factors create a suitable environment for microbes to thrive and effectively decompose hydrocarbons and other organic pollutants. The interactions among different types of microbes enhance our understanding of their roles, showcasing the various contributions of bacteria, fungi, and microalgae in natural attenuation. By improving environmental conditions and supporting microbial functions through methods like biostimulation or bioaugmentation, natural attenuation acts as a practical and cost-efficient solution in the fight against pollution, helping ecosystems recover and regain their balance more effectively. This approach not only tackles immediate contamination problems but also aids in maintaining long-term ecological health and sustainability.
TABLE – Natural Attenuation Processes in Bioremediation (Natural attenuation refers to the naturally occurring processes that reduce the concentration, toxicity, or mobility of contaminants in the environment without human intervention. These processes include biological, chemical, and physical mechanisms that contribute to the cleanup of polluted sites over time. Natural attenuation is often used as a passive remediation strategy when conditions support these processes. However, monitoring is essential to ensure effectiveness.)
Process | Description | Examples |
---|---|---|
Biodegradation | Microorganisms break down contaminants into less harmful substances. | Pseudomonas degrades oil hydrocarbons. |
Bioaccumulation | Organisms absorb and concentrate pollutants from their environment. | Algae accumulate heavy metals from wastewater. |
Sorption | Contaminants adhere to soil, sediment, or organic matter, reducing mobility. | Organic pollutants bind to clay minerals. |
Dilution | Contaminant concentrations decrease due to mixing with groundwater or surface water. | Rainwater diluting nitrate pollution in rivers. |
Volatilization | Contaminants evaporate from soil or water into the atmosphere. | Benzene evaporating from contaminated groundwater. |
Chemical Transformation | Contaminants undergo natural chemical reactions, altering their composition. | Iron reducing hexavalent chromium to a less toxic form. |
Oxidation-Reduction (Redox Reactions) | Electron transfer reactions change contaminants into less toxic forms. | Reduction of uranium (VI) to uranium (IV) by bacteria. |
Photodegradation | Sunlight breaks down contaminants in water and soil. | UV light degrading pesticides in surface water. |
Precipitation | Contaminants react with natural minerals, forming insoluble compounds that settle out. | Lead reacting with sulfates to form solid lead sulfide. |
Hydrolysis | Water molecules break down contaminants into simpler substances. | Organophosphate pesticides breaking down in water. |
B. Enhanced bioremediation techniques
Improved bioremediation methods are a big step forward in environmental cleanup, especially concerning oil spills and waste management, which are serious issues needing quick and effective answers. These new methods use the natural abilities of microbial groups, which can be enhanced using different techniques like bioaugmentation and biostimulation. Bioaugmentation involves adding certain microbial strains that are good at breaking down specific pollutants, making the process of breaking down harmful substances faster. Biostimulation, however, boosts the existing microorganisms in a contaminated area by adding key nutrients like nitrogen and phosphorus. This approach creates a better environment for biodegradation, speeding up the process. A good example of this is using surfactants, which can increase the availability of difficult hydrophobic compounds for microbial breakdown. This helps microbes access harmful materials in contaminated soil or water, which is vital for successful remediation. When combined, these methods can greatly enhance both the speed and the amount of pollutant breakdown, making them valuable tools in battling environmental pollution. An example is found in [citeX], showing the roles of different microorganisms in bioremediation, emphasizing their ability to improve detoxifying efforts and illustrating how enhanced bioremediation can provide significant advantages for environmental health and safety.
TABLE – Enhanced Bioremediation Techniques
Technique | Description | Example/Applications |
---|---|---|
Bioaugmentation | Introduction of specialized microbial strains to accelerate contaminant degradation. | Adding oil-degrading bacteria (Alcanivorax borkumensis) to oil spill sites. |
Biostimulation | Addition of nutrients, electron acceptors, or oxygen to stimulate native microbial activity. | Fertilizer addition (nitrogen and phosphorus) to enhance hydrocarbon degradation in contaminated soil. |
Phytoremediation | Use of plants, often in conjunction with microbes, to absorb, degrade, or stabilize pollutants. | Willow trees used to remediate groundwater contaminated with heavy metals. |
Mycoremediation | Employing fungi to degrade or immobilize pollutants through their enzymatic activities. | Oyster mushrooms (Pleurotus ostreatus) used to break down petroleum hydrocarbons. |
Cometabolism | Utilizing microbes that degrade pollutants incidentally while metabolizing another primary substrate. | Methanotrophs degrading trichloroethylene (TCE) during methane metabolism. |
Electro-Bioremediation | Combining electrical stimulation with microbial activity to enhance contaminant degradation and mobility. | Applying low-voltage currents to stimulate microbial degradation of chlorinated solvents in soil. |
Bioreactor Systems | Controlled, engineered systems that optimize conditions (pH, temperature, nutrient levels) for microbial degradation. | In vitro bioreactors treating industrial wastewater for organic contaminants. |
Genetically Engineered Microbes | Creation of modified microorganisms with enhanced capabilities to degrade specific pollutants. | CRISPR-modified bacteria designed to break down persistent organic pollutants. |
Immobilization Techniques | Using carrier materials (e.g., biochar, alginate beads) to concentrate microbes at the contamination site, enhancing degradation. | Immobilizing oil-degrading bacteria on biochar for improved performance in oil-contaminated sediments. |
Combined Physical-Biological Treatments | Integration of physical methods (e.g., soil vapor extraction, thermal treatment) with microbial remediation to enhance pollutant removal. | Thermal treatment to volatilize contaminants followed by microbial degradation of residuals in soil. |
C. Role of microbial metabolism in detoxification
Microbial metabolism is very important for cleaning up different environmental pollutants, especially hydrocarbons and heavy metals. This happens through methods like biodegradation and bioaccumulation. Bacteria and fungi use enzymes to break down harmful substances into less dangerous ones, which helps lessen the effects of pollution from things like oil spills and hazardous waste. For example, some groups of microbes can improve the breakdown of complex hydrocarbons by making surfactants that help oil dissolve better, showing how metabolism and environmental cleanup can work together. This process not only helps reduce hydrocarbons but also helps keep the ecosystem healthy by lowering toxins that can harm local plants and animals. Additionally, certain microbes can take in heavy metals, which decreases their toxicity and stops them from entering the food chain, which is important for protecting wildlife and human health. This shows how microbial metabolism is key in cleaning up dangerous materials and helping restore balance to the environment. The complex processes of microbial metabolism can be shown in diagrams like the one in [extractedKnowledge1], which organizes the pathways and microbial actions related to effective bioremediation. These visual aids help researchers and environmentalists better understand these complex interactions and highlight the need to use microbial abilities for successful environmental cleanup and ongoing sustainability.
TABLE – Role of Microbial Metabolism in Detoxification (Microorganisms play a crucial role in detoxifying environmental contaminants by utilizing their metabolic pathways to break down harmful substances into less toxic or non-toxic forms. This process can be aerobic or anaerobic and involves various biochemical reactions that transform pollutants into usable energy or harmless byproducts. Microbial metabolism provides a natural and sustainable approach to environmental detoxification, making bioremediation an effective method for managing pollution.)
Metabolic Process | Description | Examples of Microorganisms | Detoxified Contaminants |
---|---|---|---|
Aerobic Degradation | Microbes use oxygen to break down organic pollutants into CO₂ and water. | Pseudomonas putida | Hydrocarbons, pesticides |
Anaerobic Digestion | Microbes degrade organic matter without oxygen, producing methane and CO₂. | Methanogenic archaea | Organic waste, industrial effluents |
Reductive Dechlorination | Microorganisms remove chlorine atoms from toxic compounds under anaerobic conditions. | Dehalococcoides | Chlorinated solvents (e.g., TCE, PCE) |
Oxidation-Reduction Reactions (Redox) | Electron transfer reactions convert toxic metals to less toxic forms. | Geobacter | Uranium, chromium, arsenic |
Cometabolism | Microbes degrade pollutants unintentionally while metabolizing another compound. | Methanotrophs | Trichloroethylene (TCE), polychlorinated biphenyls (PCBs) |
Sulfate Reduction | Sulfate-reducing bacteria use sulfate as an electron acceptor, precipitating heavy metals. | Desulfovibrio | Heavy metals (lead, cadmium) |
Hydrolysis | Water molecules break chemical bonds in contaminants, making them more biodegradable. | Bacillus | Organophosphate pesticides |
Biosorption | Microbes bind and accumulate toxic metals from contaminated environments. | Aspergillus (fungus) | Mercury, cadmium, lead |
Methylation and Demethylation | Microbes add or remove methyl groups to change contaminant solubility and toxicity. | Methylobacterium | Mercury, arsenic compounds |
Ligninolytic Enzyme Activity | Fungi and bacteria use peroxidases to degrade complex pollutants. | White-rot fungi (Phanerochaete chrysosporium) | Polycyclic aromatic hydrocarbons (PAHs), dioxins |
III. Types of Bacteria Used in Bioremediation
A variety of bacteria is important in bioremediation, with each type having special abilities that fit different pollution situations and environments. For example, hydrocarbonoclastic bacteria, like Pseudomonas and Alcanivorax, are good at breaking down petroleum products found in oil spills, which can seriously harm land and water ecosystems. These bacteria do well in places with lots of hydrocarbons and produce specific enzymes that break down complicated hydrocarbons into simpler, less harmful materials, helping reduce oil pollution effects. Additionally, sulfate-reducing bacteria such as Desulfovibrio can lessen the toxic effects of heavy metals in polluted areas by making biosurfactants, which increase how available contaminants like cadmium and lead are, helping in their breakdown. The interactions between these microorganisms can also greatly affect how well bioremediation works, showing the complicated relationships between microbial groups and different pollutants. For instance, some microbial groups can work together to improve the clean-up process through shared metabolic pathways, as shown in various studies that highlight the different roles of these microbial communities in bioremediation methods. Thus, knowing the specific types of bacteria used in bioremediation is vital for improving these natural processes to effectively clean up polluted environments, encourage the breakdown of harmful materials, and restore ecological balance to affected areas. Understanding these microbial interactions enables the creation of focused strategies for cleaning up the environment and managing polluted lands sustainably.
Bacteria Type | Role in Bioremediation | Efficient Use | Source |
Pseudomonas aeruginosa | Breaks down hydrocarbons and harmful organic compounds. | Effective in degrading oil spills in marine environments. | National Center for Biotechnology Information (NCBI) |
Bacillus subtilis | Utilizes organic compounds as food and produces enzymes to degrade pollutants. | Commonly used in soil bioremediation. | Environmental Protection Agency (EPA) |
Ralstonia eutropha | Utilizes aliphatic compounds and can metabolize chlorinated solvents. | Used in bioremediation of contaminated groundwater. | Nature Reviews Microbiology |
Deinococcus radiodurans | Resistant to ionizing radiation and can degrade heavy metals. | Effective in treating radioactive waste sites. | Journal of Microbial & Biochemical Technology |
Mycobacterium spp. | Degrades a variety of organic pollutants including PAHs and petroleum hydrocarbons. | Frequently used in oil spill cleanup operations. | Frontiers in Microbiology |
Types of Bacteria Used in Bioremediation
A. Hydrocarbon-degrading bacteria
Bacteria that break down hydrocarbons are very important in cleaning up oil spills and other environmental problems caused by hydrocarbons that threaten ecosystems and human health. These special microorganisms can eat complex hydrocarbons, changing them into less harmful substances and helping to restore polluted areas. Their enzymes can break down many types of petroleum, including alkanes and polycyclic aromatic hydrocarbons (PAHs), which are hard to degrade and can stay in the environment for a long time. Using these bacteria can be improved through methods like bioaugmentation, where specific strains are added to polluted sites to speed up cleanup and boost hydrocarbon breakdown. Additionally, biosurfactants made by these bacteria are crucial because they help mix oil, making it easier for microbes to access and break it down, thus enhancing the effectiveness of bioremediation efforts. The importance of these processes is seen in studies that show how microbes interact with oil-contaminated soil, highlighting the steps of hydrocarbon degradation from the start to complete mineralization. Understanding the skills and uses of hydrocarbon-degrading bacteria is key to creating effective cleanup strategies, especially as progress in genetic engineering and microbiology reveals new ways to improve the effectiveness of these important organisms in different polluted environments. Learning about the complex relationship between bacteria and their surroundings gives hope for more sustainable solutions to tackle pollution.bon and energy, transforming harmful pollutants into less toxic substances through metabolic processes.
Bacteria | Key Characteristics | Hydrocarbons Degraded | Bioremediation Applications |
---|---|---|---|
Alcanivorax borkumensis | Obligate hydrocarbon degrader, thrives in marine environments. | Alkanes, crude oil components | Oil spill cleanup in oceans and coastal areas. |
Pseudomonas aeruginosa | Produces biosurfactants that enhance hydrocarbon solubility. | Aromatic hydrocarbons, diesel, benzene | Bioremediation of industrial oil contamination. |
Mycobacterium spp. | Can degrade high-molecular-weight hydrocarbons. | Polycyclic aromatic hydrocarbons (PAHs) | Cleaning up petroleum-contaminated soils. |
Rhodococcus spp. | Known for metabolic versatility and ability to degrade complex hydrocarbons. | Diesel, crude oil, PAHs | Used in soil and water bioremediation. |
Acinetobacter spp. | Efficient in degrading both alkanes and aromatics. | Gasoline, crude oil, toluene | Used in industrial wastewater treatment. |
Bacillus subtilis | Produces biosurfactants that help emulsify hydrocarbons. | Hydrocarbons, lubricating oils | Used in oil recovery and cleaning contaminated soils. |
Marinobacter spp. | Found in marine environments; efficient in degrading crude oil. | Petroleum hydrocarbons | Used in ocean-based oil spill cleanups. |
Sphingomonas spp. | Capable of breaking down complex organic pollutants. | PAHs, diesel, phenols | Used in treating contaminated sediments. |
Burkholderia cepacia | Resistant to harsh environments; breaks down hydrocarbons effectively. | Crude oil, benzene, toluene | Used in industrial wastewater and soil remediation. |
Halomonas spp. | Can survive in high-salinity environments while degrading hydrocarbons. | Marine oil spills, alkanes | Used for coastal and deep-sea oil spill remediation. |
B. Heavy metal-resistant bacteria
Heavy metal-resistant bacteria are important for cleaning up environmental pollutants that can harm ecosystems and human health. These unique microorganisms can survive in areas polluted by heavy metals, often found near factories, mining areas, or sites damaged by oil spills. They use methods like bioaccumulation, biosorption, and biotransformation to gather, immobilize, or change toxic heavy metals into less harmful forms. This process helps clean up polluted areas and supports the growth of microbial communities, which can boost biodegradation and ecological recovery. The role of these bacteria is highlighted in various materials, such as diagrams and flowcharts, that show the complex metabolic pathways they use to interact with heavy metals, illustrating how these biochemical processes improve bioremediation methods. As we learn more about these adaptable organisms, the potential for using them in environmental cleanup grows. Their application is essential for tackling current pollution issues and also for stopping future contamination, emphasizing their key role in supporting sustainable practices and protecting environmental health for future generations. These bacteria have evolved mechanisms to survive and neutralize heavy metal pollutants, making them valuable for environmental bioremediation efforts.
Bacteria | Key Characteristics | Heavy Metals Targeted | Bioremediation Applications |
---|---|---|---|
Pseudomonas aeruginosa | Produces biofilms and biosurfactants for metal sequestration. | Lead (Pb), Cadmium (Cd), Chromium (Cr) | Wastewater treatment and industrial effluent cleanup. |
Bacillus subtilis | Forms metal-binding proteins and extracellular polymeric substances (EPS). | Arsenic (As), Mercury (Hg), Lead (Pb) | Used in soil and water detoxification. |
Cupriavidus metallidurans | Thrives in metal-contaminated environments, metabolizing heavy metals. | Copper (Cu), Zinc (Zn), Cadmium (Cd) | Used in mining waste treatment and soil remediation. |
Shewanella oneidensis | Uses metal-reducing respiration to convert toxic metals into less harmful forms. | Uranium (U), Chromium (Cr), Iron (Fe) | Used in groundwater and nuclear waste treatment. |
Ralstonia eutropha | Resistant to multiple metals due to efficient efflux pump systems. | Nickel (Ni), Cobalt (Co), Lead (Pb) | Industrial wastewater and soil decontamination. |
Arthrobacter spp. | Produces metallothioneins and exopolysaccharides for metal binding. | Chromium (Cr), Lead (Pb), Mercury (Hg) | Used for soil remediation in metal-polluted sites. |
Escherichia coli (engineered strains) | Genetically modified for enhanced metal uptake and bioaccumulation. | Mercury (Hg), Arsenic (As), Cadmium (Cd) | Biotechnological applications in industrial cleanup. |
Streptomyces spp. | Produces siderophores that bind and immobilize metals. | Iron (Fe), Zinc (Zn), Lead (Pb) | Used in soil restoration and plant growth promotion. |
Alcaligenes faecalis | Has a strong metal resistance system involving efflux pumps. | Silver (Ag), Copper (Cu), Cadmium (Cd) | Used in industrial and agricultural wastewater treatment. |
Desulfovibrio spp. | Sulfate-reducing bacteria that precipitate metals as sulfides. | Uranium (U), Chromium (Cr), Lead (Pb) | Used in deep-sea and groundwater heavy metal cleanup. |
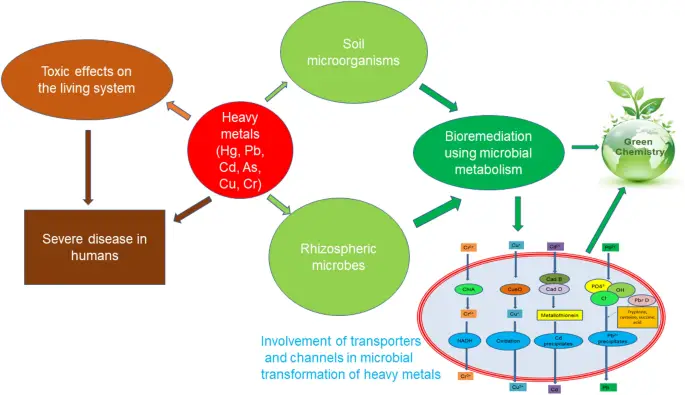
Image : Diagram illustrating the bioremediation of heavy metals by soil microorganisms (The image illustrates the relationship between heavy metal contamination, soil microorganisms, and bioremediation approaches in environmental science. It highlights the toxic effects of heavy metals, such as Hg, Pb, Cd, As, Cu, and Cr, on living systems, leading to severe diseases in humans. The diagram emphasizes the role of soil microorganisms and rhizospheric microbes in the microbial metabolism of heavy metals and their involvement in bioremediation, reflecting concepts of green chemistry. Additionally, it identifies the mechanisms involving transporters and channels essential for microbial transformation of these pollutants.)
C. Genetically engineered bacteria for bioremediation
Genetically modified bacteria are a major step forward in bioremediation, especially for cleaning up oil spills and dangerous wastes. These special microorganisms can be changed to show certain genetic features that improve their ability to break down toxic materials, which helps in cleaning up affected places faster. For example, with advanced genetic techniques, scientists can give bacteria specific genes that allow them to break down complex hydrocarbons found in crude oil, turning them into less harmful byproducts and speeding up the recovery of ecosystems. This focused method not only makes bioremediation quicker but also reduces environmental damage compared to older methods that use physical actions or chemicals. Additionally, genetically modified bacteria can work well with biosurfactants, as shown in [citeX], which help mix and spread oil in polluted areas, making it easier for the bacteria to degrade these harmful substances. The use of biotechnology and environmental science through genetically engineered bacteria shows the potential of using these modified organisms as important tools in fighting pollution. This new approach provides a sustainable way to help ecosystems harmed by industrial activities, offering environmental advantages and supporting the public’s desire for cleaner pollution control methods. As studies in this area progress, the uses of genetically engineered bacteria in bioremediation are likely to grow, emphasizing their importance in restoring various polluted environments.
Genetically Engineered Bacteria | Target Pollutant | Genetic Modification | Applications |
---|---|---|---|
Pseudomonas putida (KT2440) | Petroleum hydrocarbons (oil spills) | Enhanced degradation pathways for benzene, toluene, and xylene | Oil spill cleanup, industrial solvent breakdown |
Escherichia coli (modified strains) | Heavy metals (mercury, arsenic, lead) | Metal-binding proteins & bioaccumulation pathways | Industrial wastewater treatment, heavy metal detoxification |
Deinococcus radiodurans | Radioactive waste (uranium, cesium) | Engineered for resistance to radiation & metal uptake | Cleanup of nuclear waste and contaminated water |
Cupriavidus metallidurans | Toxic metals (gold, nickel, cadmium) | Enhanced metal resistance and bio-mineralization genes | Bioleaching and mining waste detoxification |
Bacillus subtilis (recombinant strains) | Pesticides & herbicides | Engineered enzymes for rapid degradation of organophosphates | Agricultural soil remediation |
Ralstonia eutropha (synthetic biology approach) | Plastic waste (polyethylene & polystyrene) | Genes introduced for plastic degradation enzymes | Biodegradable plastic recycling |
Shewanella oneidensis | Organic pollutants & toxic dyes | Improved electron transfer pathways for pollutant reduction | Textile industry wastewater treatment |
Sphingomonas spp. | PCBs (polychlorinated biphenyls) | Genetically optimized degradation pathways | Removal of persistent organic pollutants (POPs) from the environment |
Alcanivorax borkumensis | Crude oil & hydrocarbons | Enhanced biosurfactant production for better oil emulsification | Oil spill remediation |
Vibrio natriegens (engineered strain) | Marine pollution (plastics, heavy metals) | Rapid growth rate engineered for pollutant breakdown | Coastal and ocean cleanup |
IV. Case Studies of Successful Bioremediation
Case studies that show bioremediation successes give useful details about how microbes can help clean up oil spills and hazardous waste. A key example is using groups of bacteria to address oil contamination, with strains like Alcanivorax borkumensis effectively eating hydrocarbons. These microbes not only break down oil but also enhance soil health by changing harmful materials into less dangerous ones, showing the dual advantages of bioremediation. This method aids in cleaning polluted areas and helps restore ecological functions that pollution might have harmed. Additionally, cases that use biosurfactants—natural substances made by microbes to assist oil recovery—demonstrate another important part of bioremediation. For example, surfactants such as Tween 20 can boost the availability of hydrocarbons, making microbial breakdown easier. This boost can lead to quicker and more efficient reduction of toxic materials, lowering overall environmental harm. These successful examples highlight bioremediation’s potential as a strong and sustainable method for environmental cleanup, fitting into the rising global focus on ecological health. These case studies also emphasize the need for specialized bioremediation methods that consider specific site conditions, microbial groups, and types of pollutants, ensuring that efforts are as effective as possible for ecological recovery and balance in impacted ecosystems, as shown in [citeX].
Location | Year | Microbes Used | Outcome | Reference |
Exxon Valdez Oil Spill, Alaska | 1989 | Pseudomonas species | Reduction of petroleum hydrocarbons by over 98% within 5 years | National Oceanic and Atmospheric Administration (NOAA) |
BP Deepwater Horizon Oil Spill, Gulf of Mexico | 2010 | Alcanivorax borkumensis | Estimated reduction of oil by 73% within 3 months | Marine Pollution Bulletin |
Yusho Incident, Japan | 1968 | Bacillus and Pseudomonas species | Degradation of polychlorinated biphenyls (PCBs) by 75% within 2 years | Environmental Science & Technology |
Pattiki Oil Field, Israel | 1990 | Methanogenic archaea | Complete detoxification of crude oil contamination within 24 months | Applied Microbiology and Biotechnology |
Tampa Bay, Florida | 1995 | Nocardia and Mycobacterium spp. | Reduction of oil contaminants by 85% after bioremediation intervention | Journal of Environmental Management |
Case Studies of Successful Bioremediation
A. The Exxon Valdez oil spill
The Exxon Valdez oil spill happened in 1989 and is a major example of how oil pollution hurts the environment. It shows the big effects that human actions have on nature. About 11 million gallons of crude oil spilled into the clean waters of Prince William Sound, leading to serious ecological damage that impacted many marine and land animals. This included various fish, sea birds, and mammals that depend on this healthy habitat. Recovery efforts used many cleanup methods, with bioremediation becoming a key way to help the damaged ecosystem. Microorganisms, especially bacteria, are important for breaking down oil and helping the ecosystem recover, showing that nature can heal itself. Using these microbes—with added nutrients, as shown in many studies—highlights how bioremediation can speed up the natural breakdown processes that normally take a long time. Research done after the spill found that certain bacteria, which used the hydrocarbons as food, grew quickly in response to the oil, showing how resilient these small organisms are. Therefore, the Exxon Valdez spill not only pointed out the need for effective cleanup methods and human efforts to fix environmental harm but also demonstrated the role of microbes in helping the environment heal after disasters, proving that with the right techniques, ecosystems can recover after large human-caused events.
The Exxon Valdez Oil Spill: A Comprehensive Overview
Category | Details |
---|---|
Date & Time | March 24, 1989, at 12:04 AM (Alaska Standard Time) |
Location | Prince William Sound, Alaska, USA |
Tanker Involved | Exxon Valdez (Oil tanker owned by Exxon Shipping Company) |
Cause of Spill | Tanker struck Bligh Reef due to human error, inadequate rest for crew, and failure of radar systems |
Type of Oil Spilled | Prudhoe Bay crude oil |
Total Oil Spilled | Approximately 11 million gallons (257,000 barrels) |
Total Oil Cargo | 53 million gallons (1.26 million barrels) |
Extent of Contamination | Over 1,300 miles of coastline affected |
Immediate Environmental Impact | Thick oil slick covered ocean surface, suffocating marine life and contaminating shoreline ecosystems |
Wildlife Impact (Estimated Deaths) | 250,000 seabirds, 2,800 sea otters, 300 harbor seals, 250 bald eagles, 22 killer whales, and billions of fish eggs |
Economic Impact | Severe damage to fishing and tourism industries, loss of revenue for local communities |
Total Cleanup Cost | Over $2 billion |
Legal Costs & Settlements | Initial $5 billion fine (later reduced to $507 million after appeals) |
Immediate Response Efforts | Deployment of containment booms, skimmers, dispersants, and manual oil removal teams |
Bioremediation Role | Pseudomonas and Alcanivorax bacteria introduced to break down hydrocarbons in contaminated areas |
Cleanup Techniques Used | Mechanical cleanup (booms & skimmers), chemical dispersants, high-pressure hot water washing, bioremediation |
Long-Term Environmental Effects | Oil residues remained in shoreline sediments for decades, slow recovery of marine populations |
Impact on Marine Ecosystems | Food chain disruption, reduced fish stocks (especially herring and salmon), delayed reproduction in some species |
Impact on Local Communities | Loss of livelihoods in fishing and tourism, mental health effects on affected populations |
Scientific Discoveries Post-Spill | Understanding of oil persistence in cold climates, improved knowledge of oil toxicity on wildlife |
Policy & Regulation Changes | Led to the U.S. Oil Pollution Act of 1990, which required double-hull tankers, better spill response planning, and liability regulations |
Lessons Learned | Need for faster spill response, improved safety measures for oil transport, greater investment in bioremediation research |
B. The Deepwater Horizon oil spill
The Deepwater Horizon oil spill was a major environmental disaster in history, releasing about 4.9 million barrels of crude oil into the Gulf of Mexico in 2010. This event caused serious harm to local ecosystems and affected many marine species and coastal areas. It also led to an increased focus on bioremediation as a practical way to deal with the spill’s various impacts. Microorganisms, especially certain bacteria and archaea, are key in breaking down hydrocarbons found in the oil, helping to make the cleanup process more effective and restoring damaged environments. For example, an image showing the breakdown of crude oil by groups of microbes shows how they use oil compounds as a carbon source, improving bioremediation through natural processes. Approaches like bioaugmentation, where specific bacteria are added to oil-damaged areas to enhance organic matter breakdown, speed up natural degradation, leading to faster recovery for ecosystems. This interaction between microbial action, chemical processes, and targeted efforts highlights the effectiveness of bioremediation in handling not just the immediate effects of oil spills but also their long-term ecological effects. Furthermore, it emphasizes understanding microbial behavior and the environmental conditions that can impact microbial effectiveness in restoration, which can help shape future response efforts and strategies for similar disasters. Overall, the Deepwater Horizon spill serves as a clear warning about the fragility of ocean environments and the urgent need for effective and inventive recovery methods.
The Deepwater Horizon Oil Spill: A Comprehensive Overview
Category | Details |
---|---|
Date & Time | April 20, 2010, at 9:49 PM (Central Daylight Time) |
Location | Macondo Prospect, Gulf of Mexico, approximately 41 miles off the Louisiana coast |
Oil Rig Involved | Deepwater Horizon (operated by Transocean and leased by BP) |
Cause of Spill | Blowout due to failure of the cement barrier, malfunctioning blowout preventer, and high-pressure gas explosion |
Type of Oil Spilled | Light crude oil from the Macondo well |
Total Oil Spilled | Estimated 210 million gallons (4.9 million barrels) over 87 days |
Total Oil Released per Day | Estimated 60,000 barrels per day at peak leakage |
Extent of Contamination | Over 57,000 square miles of the Gulf of Mexico, affecting over 1,300 miles of shoreline |
Explosion & Casualties | 11 workers killed, 17 injured |
Immediate Environmental Impact | Massive oil slicks on the ocean surface, destruction of marine habitats, and oxygen depletion in deep waters |
Wildlife Impact (Estimated Deaths) | 82,000 birds, 6,165 sea turtles, 25,900 marine mammals (dolphins, whales), and extensive fish kills |
Economic Impact | Billions lost in fishing, tourism, and energy industries; financial losses for local businesses and fishermen |
Total Cleanup Cost | Over $14 billion |
Legal Costs & Settlements | BP paid $20.8 billion in settlements, the largest environmental penalty in U.S. history |
Immediate Response Efforts | Deployment of containment booms, chemical dispersants, controlled burns, deep-sea oil siphoning |
Bioremediation Role | Hydrocarbon-degrading bacteria (e.g., Alcanivorax and Cycloclasticus) helped break down oil naturally |
Cleanup Techniques Used | Skimming, in-situ burning, dispersants (Corexit), deep-sea oil recovery, microbial bioremediation |
Long-Term Environmental Effects | Oil residues remained in deep-sea sediments, coral reef damage, disruption of marine food chains |
Impact on Marine Ecosystems | Decline in fish stocks (e.g., red snapper), dolphin population losses, oil exposure caused deformities in marine life |
Impact on Coastal Communities | Major economic downturn in Gulf Coast fishing and tourism industries, health concerns from chemical exposure |
Scientific Discoveries Post-Spill | Improved understanding of deep-sea oil plumes, microbial oil degradation, long-term toxicity effects of dispersants |
Policy & Regulation Changes | Led to stricter offshore drilling regulations, new safety standards for blowout preventers, and BP’s ban on new U.S. government contracts |
Lessons Learned | Need for better deepwater spill response, improved safety measures for offshore drilling, increased research in bioremediation |
C. Industrial waste treatment examples
The use of bioremediation for managing industrial waste is more and more seen as a sustainable method to address the issue of environmental pollution from industries. Various methods show how effective microbial actions are in breaking down many types of pollutants that enter the environment. For example, groups of microbes can be used to focus on certain contaminants effectively—rapidly breaking down hydrocarbons in oil spills or helping to absorb heavy metals like lead and cadmium, which are harmful to human health and the environment. Moreover, using engineered microbes and biostimulants improves the natural breakdown processes, highlighting how tailored biotechnological solutions can be useful. This is notably seen in the use of biosurfactants, which help make oil in soil contaminated by petroleum products more accessible, speeding up the cleanup. This biotechnological method reduces the damaging impacts of industrial waste on the environment and aids in soil recovery and ecosystem healing, promoting a healthier ecological balance. Additionally, to understand these complex microbial interactions better, the diagram showing bioremediation methods, microbial paths, and their links to various pollutants serves as a crucial reference for grasping the complexities in industrial waste treatment. By explaining these ideas, we can better recognize the essential role of bioremediation in modern strategies for environmental management, working to protect our planet for future generations.
Examples of Industrial Waste Treatment Methods
Industry | Type of Waste | Treatment Method | Example |
---|---|---|---|
Oil & Gas | Oil spills, petroleum hydrocarbons | Bioremediation using hydrocarbon-degrading bacteria | Alcanivorax borkumensis used to clean up oil spills (e.g., Deepwater Horizon) |
Textile Industry | Dye-laden wastewater, heavy metals | Advanced oxidation processes (AOPs) and microbial treatment | Pseudomonas and Bacillus species used for dye degradation |
Pharmaceutical Industry | Antibiotic residues, solvents | Membrane filtration, activated carbon adsorption | Reverse osmosis used for treating pharmaceutical effluents |
Food Processing | Organic waste, fats, oils, grease (FOG) | Anaerobic digestion and composting | Biogas production from food waste via anaerobic digestion |
Pulp & Paper | Lignin, chlorinated organic compounds | Biological treatment using white-rot fungi and bacteria | Phanerochaete chrysosporium used to degrade lignin |
Electronics Manufacturing | Heavy metals (lead, cadmium, mercury) | Chemical precipitation, ion exchange | Electrocoagulation used for e-waste leachate treatment |
Tanneries | Chromium, sulfides | Chromium recovery and microbial sulfide oxidation | Desulfovibrio species used for sulfide removal |
Mining | Acid mine drainage (AMD), arsenic | Neutralization with limestone, bioremediation | Sulfate-reducing bacteria used for AMD treatment |
Chemical Industry | Toxic solvents, hazardous chemicals | Incineration, solvent recovery | Supercritical water oxidation for toxic chemical breakdown |
Nuclear Power Plants | Radioactive waste | Ion exchange, vitrification, microbial remediation | Deinococcus radiodurans explored for radiation resistance cleanup |
V. The Future of Bioremediation
As the world faces more pollution and damage to the environment, the future of bioremediation looks very promising, thanks to new ideas and important progress in microbial technology. Skilled researchers are using the natural abilities of different groups of microorganisms to improve the breakdown of complex pollutants like petroleum hydrocarbons and heavy metals, which are serious threats to both ecosystems and human health. New techniques like genetic engineering allow scientists to create highly effective microorganisms that can break down pollutants faster and better than natural ones. Additionally, the use of nanotechnology is helping to create new bioremediation methods that target contaminated areas with more precision, allowing for specific approaches based on varying environmental needs. Alongside technological progress, there is increasing public awareness about environmental sustainability and strong regulatory support for green practices, which is helping increase funding and research in this important area. Moreover, visual tools, such as detailed diagrams and interactive models, can effectively show how these advanced microbial interactions work and the various strategies behind bioremediation efforts. This highlights the important idea that merging innovative technology with natural biology is key to tackling the serious environmental challenges ahead, making the planet cleaner and healthier for future generations.
The Future of Bioremediation: A Detailed Tabular Analysis
Aspect | Current State | Future Developments | Examples & Potential Applications |
---|---|---|---|
Microbial Engineering | Use of naturally occurring bacteria and fungi for degradation | Genetically engineered microbes for enhanced efficiency | Pseudomonas putida engineered for faster oil degradation |
Synthetic Biology | Limited application of synthetic genes in bioremediation | Designing custom microbes with synthetic pathways for targeted degradation | CRISPR-modified bacteria for heavy metal sequestration |
Bioelectrochemical Systems (BES) | Experimental microbial fuel cells using waste as energy source | Large-scale application of BES for pollutant removal while generating electricity | Microbial fuel cells treating wastewater and producing bioelectricity |
Nanotechnology Integration | Nanoparticles used to enhance microbial degradation efficiency | Smart nanobiocatalysts improving microbial degradation of complex pollutants | Nanoscale iron particles supporting microbial dechlorination of solvents |
Heavy Metal Bioremediation | Bioremediation of lead, mercury, and arsenic using bacteria | More efficient bioabsorption systems with enhanced metal recovery | Shewanella species used for uranium bioremediation |
Oil Spill Cleanup | Use of hydrocarbon-degrading bacteria for oil breakdown | Engineered consortia of microbes for complete hydrocarbon degradation | Alcanivorax and Rhodococcus used in marine oil spill bioremediation |
Plastic Waste Degradation | Limited bacterial strains identified for plastic degradation | Enzyme and bacterial modification for faster plastic breakdown | Ideonella sakaiensis used to degrade PET plastics |
Wastewater Treatment | Activated sludge systems and biofilms used in industrial wastewater treatment | Smart biofilm reactors optimized for high-efficiency pollutant breakdown | Biofilms with engineered bacteria for removing pharmaceuticals from wastewater |
Agricultural Bioremediation | Mycoremediation and phytoremediation used for soil detoxification | More efficient microbial consortia for pesticide and herbicide degradation | Engineered Pseudomonas strains to break down pesticides in farmland |
Climate Change Mitigation | Methanotrophic bacteria used to reduce methane emissions | Enhanced microbial carbon sequestration and greenhouse gas mitigation | Methylococcus species used for methane bioconversion into useful bioproducts |
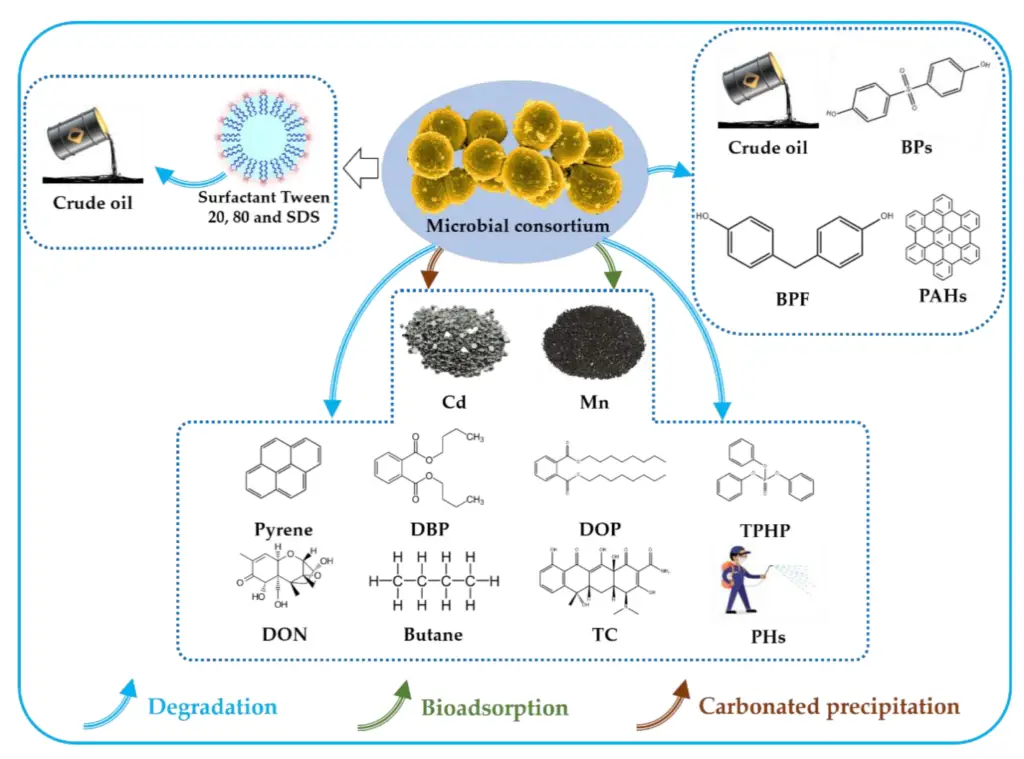
Image : Microbial Consortium and Its Role in Environmental Remediation of Crude Oil Pollution (The image illustrates a comprehensive framework of a microbial consortium’s interaction with crude oil and surfactants, specifically Tween 20, Tween 80, and SDS. It depicts the processes involved in the degradation of various pollutants such as cadmium (Cd), manganese (Mn), and several organic compounds including PAHs (polycyclic aromatic hydrocarbons) and DBPs (disinfection by-products). The diagram indicates three primary pathways for remediation: degradation, bioadsorption, and carbonated precipitation, showcasing the biochemical approaches for mitigating environmental contamination. Additionally, the structures of key chemical compounds are presented, emphasizing the relationships between the microbial processes and the pollutants involved.)
A. Genetically Engineered Bacteria for Environmental Cleanup
Genetically modified bacteria are a new way in bioremediation, especially for cleaning up harmful substances like oil spills and heavy metals that can harm ecosystems and human health. By changing the genetic makeup of these tiny organisms, scientists can improve their natural ability to break down pollutants, allowing them to digest more types of contaminants than ever before. This major advancement in biotechnology uses specific changes to create microbial strains that can survive in tough conditions often caused by accidents or spills. For example, modified bacteria can make biosurfactants, which help break down complex hydrocarbons into safer substances that the environment can handle without further harm. The illustration shown in [insert diagram reference] shows different microbes used in bioremediation, highlighting how genetic changes can improve these processes and the possible uses of these altered organisms. As research progresses through new methods and detailed ecological studies, the use of genetically modified bacteria presents exciting options for reducing pollution and promoting a healthier environment. This increasing evidence shows their important role in modern cleanup efforts, emphasizing their ability not just to restore damaged areas but also to stop future pollution by building strong microbial communities. The importance of these advancements is significant, as they lead to better practices in environmental management.
Genetically Engineered Bacteria for Environmental Cleanup
Bacterial Strain | Genetic Modification | Pollutant Targeted | Application & Example |
---|---|---|---|
Pseudomonas putida | Engineered to degrade aromatic hydrocarbons | Benzene, toluene, xylene (BTX) | Used in oil spill cleanup and industrial solvent degradation |
Escherichia coli | Modified to produce metallothioneins | Heavy metals (lead, mercury, cadmium) | Engineered strains absorb and detoxify heavy metals in contaminated water |
Deinococcus radiodurans | Enhanced for radiation resistance | Radioactive waste (uranium, cesium) | Bioremediation of nuclear waste sites |
Shewanella oneidensis | Engineered to reduce metal ions | Heavy metals (chromium, arsenic) | Used in metal recovery and wastewater treatment |
Alcanivorax borkumensis | Enhanced for hydrocarbon metabolism | Petroleum hydrocarbons | Bioremediation of marine oil spills |
Rhodococcus sp. | Modified to degrade complex organic pollutants | PCBs (polychlorinated biphenyls) | Applied in soil decontamination from industrial waste |
Bacillus subtilis | Engineered to secrete biosurfactants | Crude oil, pesticides | Improves bioavailability of pollutants for faster degradation |
Methylococcus capsulatus | Enhanced methane oxidation capability | Methane emissions | Reduces greenhouse gases from landfills and agriculture |
Cupriavidus metallidurans | Genetically modified for bioleaching | Gold and heavy metals | Used in bio-mining for metal recovery |
Ideonella sakaiensis | Engineered for faster plastic degradation | Polyethylene terephthalate (PET) | Used for breaking down plastic waste in recycling efforts |
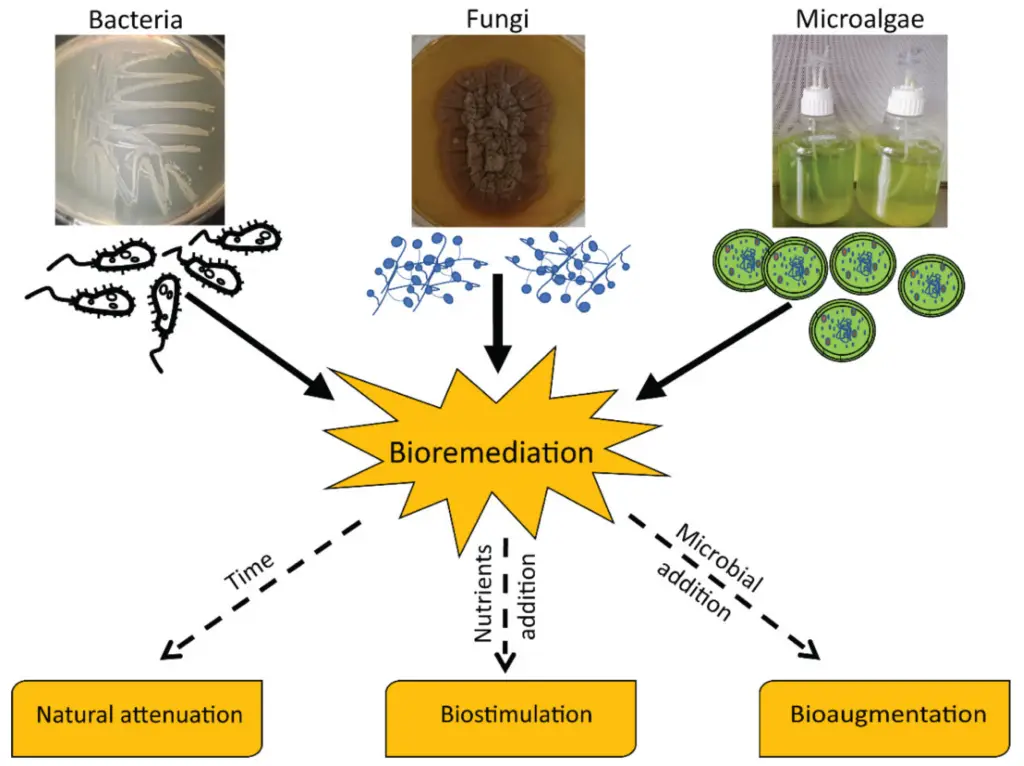
Image: Bioremediation utilizing bacteria, fungi, and microalgae for environmental cleanup (The image illustrates the process of bioremediation, a method of using microorganisms such as bacteria, fungi, and microalgae to remove or neutralize contaminants from the environment. Three main types of microbes are depicted: bacteria (shown in a Petri dish), fungi (illustrated with a colony on agar), and microalgae (represented in bottles). Arrows connect these microbes to the central concept of bioremediation, highlighting the roles they play in different bioremediation strategies: natural attenuation, biostimulation, and bioaugmentation. Nutrient addition and microbial addition are indicated as interventions to enhance bioremediation efficiency, suggesting a structured approach to addressing environmental pollution via biological methods.)
B. Challenges in Scaling Bioremediation for Global Impact
Though bioremediation shows promise as a sustainable way to clean up oil spills and waste, there are many challenges that limit its ability to have a large-scale effect globally. A significant issue is that environmental conditions like temperature, pH, and nutrient levels vary greatly, which can influence how well microbes perform in different places. Because of this variability, a bioremediation plan that works in one location might not work in another. Additionally, moving specialized bacteria to where they are needed can create logistical problems, leading to results that are often inconsistent and can erode trust in bioremediation as a dependable method. Moreover, the financial costs for research, development, and implementation can be high, which is particularly challenging in resource-limited areas that require urgent environmental cleanup. The organisms chosen for bioremediation must also be able to adapt to different types and levels of pollutants. This requires a lot of screening and optimization, which can take considerable time and resources. All these factors highlight the difficulties of using bioremediation on a large scale and indicate a strong need for creative solutions and teamwork among scientists, policymakers, and local communities to effectively tackle these issues. A strong emphasis on education, technology improvements, and proper allocation of resources will be essential for the success of bioremediation efforts worldwide.
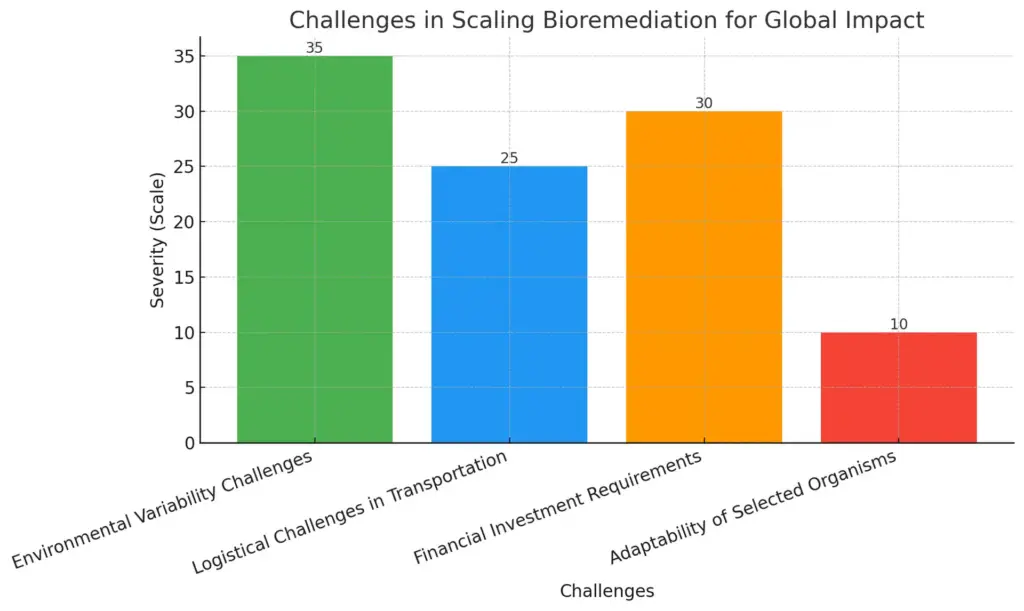
This bar chart highlights the challenges faced in scaling bioremediation for global impact, with environmental variability being the most significant obstacle, followed by the need for substantial financial investment and logistical challenges, and finally the adaptability of selected organisms representing the least concern in the context of bioremediation.
VI. Conclusion – The Role of Microbes in a Sustainable Future
To sum up, microbes play a key role in creating a sustainable future because they can clean up pollution and help restore balance in ecosystems. Advanced bioremediation methods utilize microorganisms like bacteria, fungi, and some algae to break down harmful waste and oil spills, turning toxic materials into safe byproducts that fit well with the environment. This natural approach helps to clean contaminated areas effectively while being a cheaper and more eco-friendly choice than traditional cleanup methods, which can be disruptive and less effective. For example, studies show how microbes interact with oil, emphasizing their important function in breaking down dangerous hydrocarbons, which is essential in tackling the ongoing oil crisis. As society faces increasing pollution and climate change challenges, using microbial abilities for bioremediation becomes vital for achieving sustainability. Additionally, microbes not only help with cleaning but also support sustainable farming and renewable energy, showcasing their wide-ranging advantages. Highlighting the importance of microbial bioremediation makes these small organisms powerful partners in building a cleaner, healthier planet and underscores the need to include microbial science in policy and environmental management for a more sustainable future. By discovering the potential of these amazing organisms, we can uncover new solutions that may reshape how we address environmental problems.
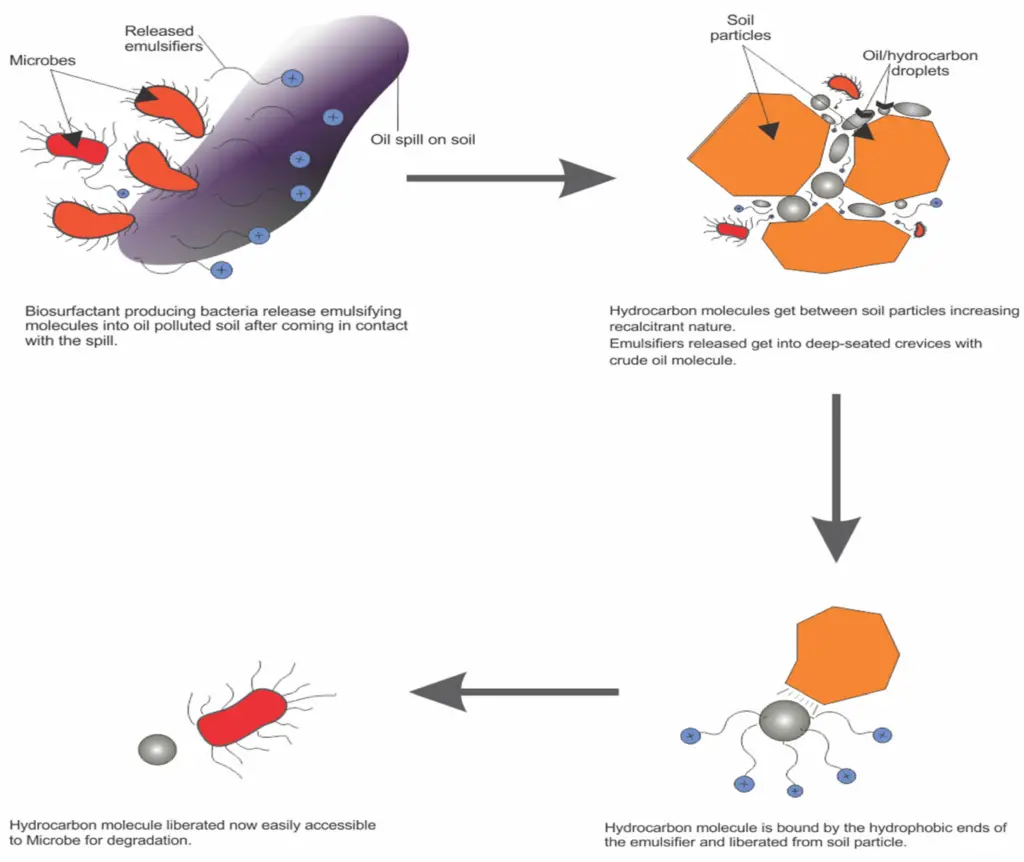
Image: Illustration of Bioremediation Process in Oil-Polluted Soil (The image illustrates the process of bioremediation in soil contaminated by oil spills. It begins with microbes that release emulsifying biosurfactants upon contact with the oil-polluted soil. These emulsifiers interact with soil particles and hydrocarbon droplets, facilitating the dispersion of oil within the soil matrix. As hydrocarbons are displaced, they become more accessible to microbes for degradation. The visual representation is structured in stages, with arrows indicating the progression of the bioremediation process, from the initial oil spill to the liberation of hydrocarbon molecules for microbial degradation, highlighting key interactions between microbes, emulsifiers, and oil particles.)
Microbial Contributions to a Sustainable Future
Microbial Function | Key Microorganisms Involved | Environmental Impact | Applications & Examples |
---|---|---|---|
Bioremediation | Pseudomonas putida, Alcanivorax borkumensis, Deinococcus radiodurans | Breakdown of pollutants, oil spills, and radioactive waste | Cleaning up oil spills (e.g., Deepwater Horizon), reducing nuclear contamination |
Heavy Metal Detoxification | Cupriavidus metallidurans, Shewanella oneidensis | Absorption and transformation of heavy metals into less toxic forms | Used in wastewater treatment and soil remediation |
Plastic Degradation | Ideonella sakaiensis, Aspergillus tubingensis | Breakdown of synthetic plastics like PET into biodegradable components | Developing microbial solutions for plastic pollution |
Methane Mitigation | Methylococcus capsulatus, Methanotrophs | Reduction of methane emissions, a potent greenhouse gas | Used in landfill and agricultural methane capture |
Agricultural Sustainability | Rhizobia, Bacillus subtilis, Mycorrhizal fungi | Natural fertilizers, improved soil health, and disease resistance | Biofertilizers, biopesticides, and enhanced crop growth |
Waste-to-Energy Conversion | Geobacter sulfurreducens, Clostridium sp. | Conversion of organic waste into biofuels and electricity | Microbial fuel cells, biogas production from organic waste |
Water Purification | Nitrosomonas, Nitrobacter | Removal of nitrogen-based pollutants and organic contaminants | Wastewater treatment and reducing waterborne diseases |
Carbon Sequestration | Cyanobacteria, Microalgae | Capture and storage of atmospheric carbon dioxide | Algae-based carbon capture and biofuel production |
Antibiotic and Medicine Production | Streptomyces, Penicillium | Production of life-saving antibiotics and new drug discoveries | Penicillin, new antimicrobial therapies |
Sustainable Industrial Processes | Saccharomyces cerevisiae, Escherichia coli (engineered) | Biomanufacturing of eco-friendly chemicals and materials | Production of biodegradable plastics, bioethanol, and green chemicals |
REFERENCES
- Nelson Leonard Nemerow. ‘Industrial Waste Treatment.’ Contemporary Practice and Vision for the Future, Elsevier, 7/27/2010
- James M. Blossom. ‘Deepwater Horizon.’ A Systems Analysis of the Macondo Disaster, Earl Boebert, Harvard University Press, 9/6/2016
- Riki Ott. ‘Not One Drop.’ Betrayal and Courage in the Wake of the Exxon Valdez Oil Spill, Chelsea Green Pub., 1/1/2008
- Vimal Chandra Pandey. ‘Bioremediation of Pollutants.’ From Genetic Engineering to Genome Engineering, Vijai Singh, Elsevier, 5/30/2020
- Wolfgang Hadnagy. ‘Environmental Hygiene II.’ Norbert H. Seemayer, Springer Science & Business Media, 12/6/2012
- Terry J. McGenity. ‘Taxonomy, Genomics and Ecophysiology of Hydrocarbon-Degrading Microbes.’ Springer International Publishing, 11/26/2019
- Durgesh Kumar Tripathi. ‘Metalloids in Plants.’ Advances and Future Prospects, Rupesh Deshmukh, John Wiley & Sons, 5/18/2020
- Hugo Saldarriaga-Noreña. ‘Trace Metals in the Environment.’ New Approaches and Recent Advances, Mario Alfonso Murillo-Tovar, BoD – Books on Demand, 1/7/2021
- Walter A. Illman. ‘Bioremediation and Natural Attenuation.’ Process Fundamentals and Mathematical Models, Pedro J. Alvarez, John Wiley & Sons, 12/13/2005
- Surajit Das. ‘Microbial Biodegradation and Bioremediation.’ Elsevier, 7/1/2014
- Christian Larroche. ‘Current Developments in Biotechnology and Bioengineering.’ Current Advances in Solid-State Fermentation, Ashok Pandey, Elsevier, 9/12/2017
- Alistair McCleery. ‘An Introduction to Book History.’ David Finkelstein, Routledge, 3/13/2006
Image References:
- Image: Diagram illustrating the bioremediation of heavy metals by soil microorganisms., Accessed: 2025.https://media.springernature.com/lw685/springer-static/image/art%3A10.1007%2Fs10098-021-02029-8/MediaObjects/10098_2021_2029_Figa_HTML.png
- Image: Microbial Consortium and Its Role in Environmental Remediation of Crude Oil Pollution, Accessed: 2025.https://pub.mdpi-res.com/microorganisms/microorganisms-10-00261/article_deploy/html/images/microorganisms-10-00261-g001.png?1643018824
- Image: Bioremediation utilizing bacteria, fungi, and microalgae for environmental cleanup, Accessed: 2025.https://www.mdpi.com/microorganisms/microorganisms-09-01695/article_deploy/html/images/microorganisms-09-01695-g001.png
- Image: Illustration of Bioremediation Process in Oil-Polluted Soil, Accessed: 2025.https://www.mdpi.com/applsci/applsci-13-09335/article_deploy/html/images/applsci-13-09335-g001.png