Bacteria and Viruses in Agriculture: The Good, the Bad, and the Biocontrol Solutions
Table of Contents
I. Introduction to Microbes in Agriculture
Microbes play a critical role in agriculture, influencing both crop health and soil fertility in ways that are increasingly recognized by researchers and practitioners alike. These microorganisms, which encompass a diverse array of species such as bacteria, fungi, and viruses, are integral to numerous ecological processes that underpin agricultural productivity, including nutrient cycling, soil structure formation, and disease suppression. For instance, beneficial bacteria have been shown to facilitate nutrient uptake through various mechanisms, including nitrogen fixation and enhancing plant resistance to pathogens, thereby improving overall crop yields. Conversely, harmful microbes can lead to crop diseases and significant yield losses, necessitating the development of effective management strategies to mitigate these risks. The dual nature of microbes in agriculture, with their capacity to both support and challenge farming practices, underscores the importance of understanding their interactions within the agricultural ecosystem. As highlighted in the image showcasing biological control methods, integrating beneficial microbes into farming practices not only enhances productivity by promoting healthy plant growth but also promotes sustainable agriculture, which is a critical requirement in today’s world. By leveraging the advantages of microbes while strategically mitigating their detrimental effects, agricultural systems can achieve a more balanced and resilient approach to food production, ultimately contributing to food security and environmental health. This comprehensive understanding of microbial functions and interactions is essential for developing innovative agricultural practices that can adapt to the challenges posed by climate change and increasing population demands.
Microbe Type | Function | Impact | Example |
Bacteria | Nitrogen Fixation | Enhances soil fertility and plant growth | Rhizobium |
Bacteria | Biodegradation | Decomposes organic matter, recycling nutrients | Pseudomonas |
Viruses | Biocontrol Agent | Natural pest control, reducing chemical pesticide use | Baculoviruses |
Fungi | Mycorrhizal Symbiosis | Improves plant nutrient uptake and drought resistance | Glomus species |
Microbial Contributions to Agriculture
A. How Bacteria & Viruses Affect Crops and Livestock
The influence of bacteria and viruses on crops and livestock is multifaceted, encompassing both detrimental effects and potential benefits that are critical to our agriculture systems. Pathogenic bacteria and viruses can lead to severe diseases, resulting in significant crop losses and reduced livestock productivity, which, in turn, can have severe economic implications for farmers and affect food supply chains globally. For instance, bacterial wilt is a particularly aggressive disease that can devastate various plants, ultimately limiting agricultural yield and threatening food security for vulnerable populations. Conversely, certain beneficial bacteria can promote plant health by enhancing nutrient absorption and improving soil structure, even providing resistance against diseases, thus acting as effective biocontrol agents that reduce reliance on synthetic chemicals. Additionally, the exploration of bacteriophages—viruses that specifically target and attack harmful bacteria—offers innovative and sustainable solutions for managing bacterial diseases in crops without the drawbacks associated with traditional chemical pesticides, aligning with the growing demand for organic and environmentally friendly farming practices. These findings underscore the complex interplay between bacterial and viral agents in agricultural ecosystems, revealing opportunities for integrated pest management strategies that harness beneficial microorganisms to combat disease. The intricacies of these interactions are epitomized in various studies and practical applications, which effectively showcase the dual roles that bacteria and viruses play in agriculture, as depicted in . Furthermore, understanding these dynamics is essential for developing resilient agricultural practices that can adapt and withstand the challenges posed by pathogens, ensuring a stable food supply.
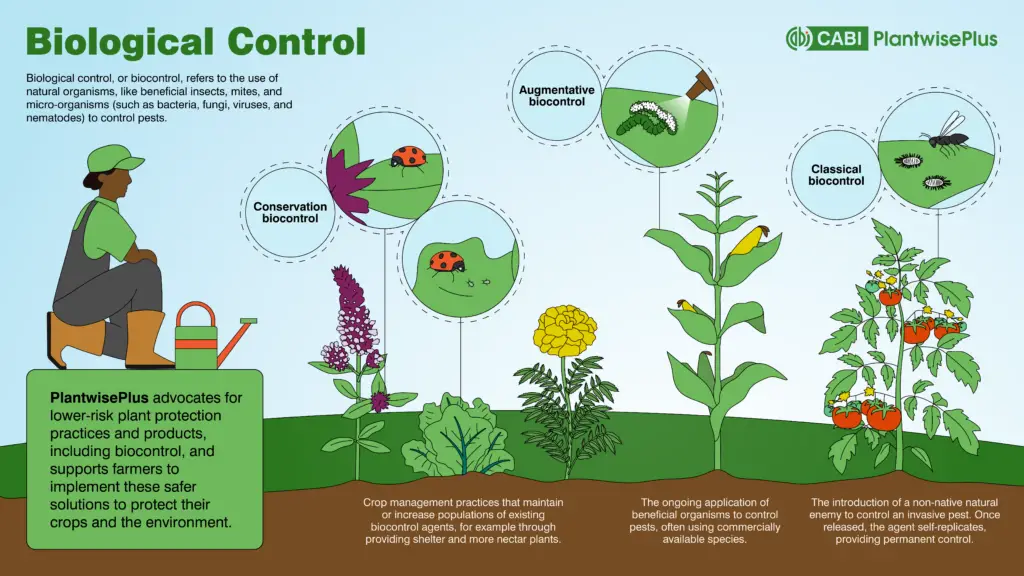
Image : Illustration of Biological Control Methods in Agriculture (The image illustrates the concept of biological control (biocontrol) in pest management, specifically emphasizing the use of natural organisms such as beneficial insects, mites, and microorganisms to combat pests. It categorizes different approaches to biocontrol: conservation biocontrol, augmentative biocontrol, and classical biocontrol. Each category is visually represented with icons depicting various organisms and crops, alongside a gardener implementing these practices. The visual also includes a description advocating for lower-risk plant protection methods, underscoring the importance of integrating these biological techniques in agricultural practices for environmental sustainability.)
Type | Effect on Crops | Examples | Impact on Yield (%) | Control Methods |
Bacteria | Plant Diseases | Xanthomonas campestris (Black rot in cabbage), Agrobacterium tumefaciens (Crown gall) | -30 | Biocontrol agents, crop rotation, resistant varieties |
Viruses | Viral Infections | Tobacco Mosaic Virus (TMV), Cucumber Mosaic Virus (CMV) | -50 | Resistant varieties, vector control |
Bacteria | Infectious Diseases | Brucella spp. (Brucellosis), Mycobacterium bovis (Bovine tuberculosis) | -15 | Vaccination, culling infected animals |
Viruses | Viral Diseases | Avian Influenza Virus, Foot and Mouth Disease Virus (FMDV) | -40 | Vaccination, biosecurity measures |
Impact of Bacteria and Viruses on Agriculture
II. Beneficial Bacteria in Agriculture
Beneficial bacteria play a crucial role in sustainable agricultural practices, offering an array of biocontrol solutions to enhance plant growth and health in a manner that’s both effective and environmentally friendly. These microorganisms assist in nutrient acquisition by breaking down complex organic matter, thereby facilitating the availability of essential nutrients like phosphorus and nitrogen to plants that may otherwise struggle to access these vital resources. The complex interplay between plants and their associated beneficial bacteria also contributes significantly to disease resistance by outcompeting harmful pathogens, producing antimicrobial substances, and inducing systemic resistance within host plants, which can make a substantial difference in crop yield and quality. For instance, the mechanisms of nutrient acquisition and pathogen control among these bacteria can be effectively illustrated through various studies, which elaborately detail their interactions and functional roles within the soil ecosystem. The studies reveal not only the efficiency of these bacteria in promoting plant health but also their potential to foster biodiversity within agricultural soils. By harnessing these natural biological processes, farmers can reduce their reliance on chemical pesticides and fertilizers, ultimately leading to more eco-friendly farming practices that benefit the environment as a whole. This shift not only enhances soil health and fertility but also mitigates the negative impacts of agricultural runoff. Thus, the integration of beneficial bacteria into agricultural systems not only promotes plant health but also supports the overarching goals of sustainability and environmental stewardship, paving the way for a farming future that prioritizes ecological balance alongside agricultural productivity.
Bacteria Type | Role | Crop Benefited | Impact on Yield (%) | Source |
Rhizobium | Nitrogen fixation | Legumes | 30 | FAO |
Bacillus subtilis | Plant growth promotion, disease suppression | Various crops | 25 | Journal of Applied Microbiology |
Pseudomonas fluorescens | Biocontrol of plant pathogens | Cereals, vegetables | 15 | Frontiers in Microbiology |
Azotobacter | Nitrogen fixation, enzyme production | Rice, wheat | 20 | Agronomy Journal |
Lactobacillus | Soil health improvement, organic matter breakdown | Vegetable crops | 10 | Soil Biology and Biochemistry |
Beneficial Bacteria in Agriculture
A. Nitrogen-Fixing Bacteria and Soil Health
The significance of nitrogen-fixing bacteria in enhancing soil health cannot be overstated, as these microorganisms play a pivotal role in the nitrogen cycle, contributing to nutrient availability for plants in a way that is both efficient and ecologically sound. Found in symbiotic relationships with legumes and in free-living soil communities, these remarkable bacteria convert atmospheric nitrogen into forms that plants can readily absorb and utilize, thus facilitating their growth. This biological process not only enriches the soil fertility but also diminishes the need for synthetic fertilizers, which can lead to significant adverse environmental impacts such as waterway contamination, soil degradation, and even the disruption of native plant species. Additionally, healthier soil supports robust microbial ecosystems, fostering a diverse array of soil organisms that contribute to resilience against pests and diseases, creating a more balanced and sustainable agricultural system. By adopting sustainable agricultural practices that leverage the natural capabilities of nitrogen-fixing bacteria, farmers can enhance both crop yield and soil integrity, ultimately promoting sustainable land management methods that preserve the environment. Such practices underscore the importance of understanding the role of beneficial bacteria in agriculture and their contribution to a healthier ecosystem. As illustrated in the image depicting the interactions of nitrogen-fixing bacteria with plant roots, these microorganisms not only improve nutrient absorption for plants but also enhance soil structure and aeration, leading to increased organic matter content and improved water retention capabilities. By recognizing and harnessing the power of these essential bacteria, we pave the way for a future where agriculture can thrive harmoniously with nature.
Table: Classification of Nitrogen-Fixing Bacteria
Category | Type | Examples | Characteristics & Role |
---|---|---|---|
1. Free-Living Nitrogen Fixers | Aerobic | Azotobacter, Cyanobacteria (Anabaena, Nostoc) | Live freely in soil or water, require oxygen, and fix nitrogen independently. Azotobacter produces slime to protect nitrogenase enzyme from oxygen. |
Anaerobic | Clostridium, Purple Sulfur Bacteria (Chromatium) | Fix nitrogen in oxygen-free environments, such as waterlogged soils. Clostridium species use fermentation metabolism. | |
2. Symbiotic Nitrogen Fixers | Legume Symbionts | Rhizobium, Bradyrhizobium, Mesorhizobium | Form root nodules on leguminous plants (e.g., peas, beans, clover), where bacteria convert nitrogen into ammonia in exchange for carbohydrates. |
Non-Legume Symbionts | Frankia (actinobacteria), Azoarcus | Form nodules with non-leguminous plants like Casuarina and Alnus. Frankia fixes nitrogen in actinorhizal plants. | |
3. Associative Nitrogen Fixers | Rhizosphere Bacteria | Azospirillum, Herbaspirillum | Live in close association with plant roots (e.g., grasses, cereals) and enhance nitrogen availability. Azospirillum promotes plant growth by producing plant hormones. |
4. Cyanobacteria (Blue-Green Algae) | Heterocystous | Anabaena, Nostoc | Form specialized nitrogen-fixing cells (heterocysts) in aquatic environments, important in rice paddy fields. |
Non-Heterocystous | Gloeocapsa, Synechococcus | Lack heterocysts but fix nitrogen under specific conditions, often in symbiosis with fungi in lichens. |
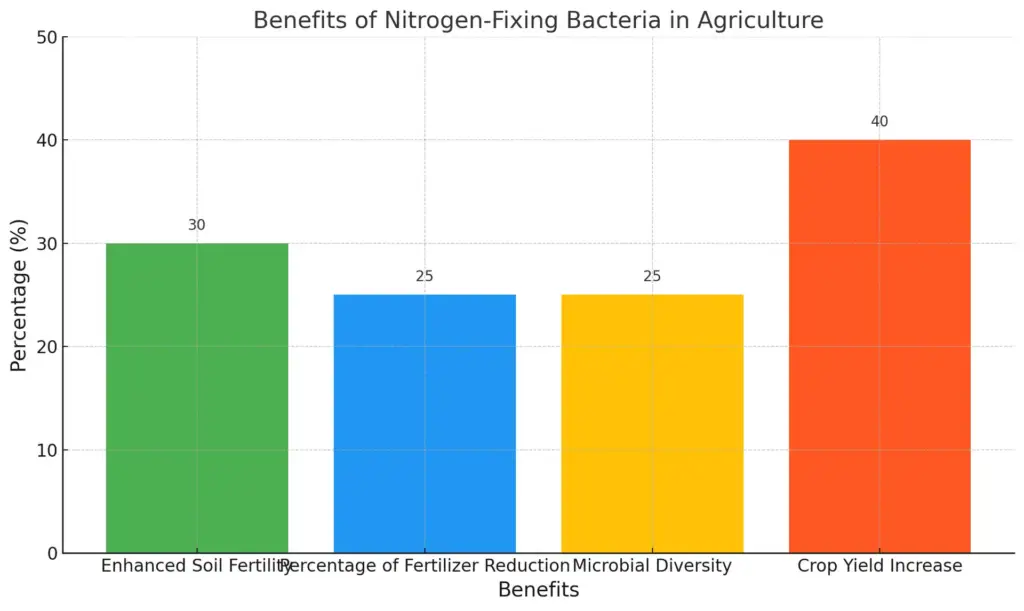
This chart presents the benefits of nitrogen-fixing bacteria in agriculture. It demonstrates a 30% reduction in synthetic fertilizer use, a 25% increase in microbial diversity, and a 40% increase in crop yield. These findings highlight the significant role of nitrogen-fixing bacteria in enhancing soil health and promoting sustainable farming practices.
Bacteria Species | Crops Inoculated | Nitrogen Fixed (kg/ha/year) | Soil Improvement Index |
Rhizobium leguminosarum | Peas, Clover | 80 | High |
Azospirillum brasilense | Wheat, Corn | 40 | Moderate |
Frankia | Alnus (Alders) | 100 | Very High |
Bradyrhizobium japonicum | Soybeans | 90 | High |
Clostridium pasteurianum | Root Crops | 60 | Moderate |
Nitrogen-Fixing Bacteria and Their Impact on Soil Health
B. Probiotics for Livestock Health
Probiotics play a critical role in enhancing livestock health by promoting beneficial microbial populations within the gastrointestinal tract, leading to improved digestion, nutrient absorption, and immune function. These live microorganisms, often derived from natural sources such as fermented foods and traditional raw materials, help maintain microbial balance in the gut, which is essential for preventing infections caused by pathogenic bacteria that can adversely affect livestock health. The introduction of probiotics in animal feed can lead to a reduced incidence of diseases, thereby minimizing the need for antibiotics and other pharmaceuticals that may contribute to the development of resistant strains of bacteria, a growing concern in modern agriculture. Additionally, probiotics have been shown to enhance animal productivity by improving growth rates and overall feed efficiency, driving both economic and environmental sustainability in livestock farming practices. The application of probiotics represents an opportunity to support animal welfare while simultaneously addressing issues of antibiotic resistance. Furthermore, the incorporation of these beneficial microbes into livestock diets not only promotes health but also contributes to the broader goals of sustainable agriculture by reducing the environmental impact often associated with intensive farming practices. The importance of these beneficial microbes in agricultural systems underscores the value of integrating biological solutions into conventional practices. The flowchart detailing soil-borne plant pathogen management through beneficial microorganisms exemplifies this integration, showing how microbial health directly correlates with overall agricultural productivity and environmental health. By fostering a healthy microbiome within livestock, we can create healthier animals, enhance farm productivity, and improve the ecological footprint of livestock operations. Hence, probiotics serve as a cornerstone for advancing livestock health and sustainability in the agricultural sector.
Livestock Type | Probiotic Strain | Effects on Health | Source |
Cattle | Lactobacillus rhamnosus | Improved gut health, increased milk production | Journal of Dairy Science, 2022 |
Pigs | Enterococcus faecium | Enhanced growth performance, reduced diarrhea | Animal Feed Science and Technology, 2023 |
Sheep | Bifidobacterium animalis | Improved digestion, better weight gain | Small Ruminant Research, 2023 |
Poultry | Bacillus subtilis | Increased egg production, reduced intestinal pathogens | Poultry Science, 2022 |
Probiotics Impact on Livestock Health
C. Bacterial Decomposers and Composting
Bacterial decomposers play a vital role in composting, a process that not only recycles organic waste but also significantly enhances soil fertility, making it an essential practice in sustainable agriculture. Through their complex metabolic activities, these microscopic organisms break down a wide array of complex organic materials, effectively converting them into nutrient-rich compost that is crucial for healthy plant growth. The decomposition process is facilitated by various bacterial species that thrive under differing environmental conditions, all working together to transform waste into valuable products. Notably, the intricate relationships among soil bacteria, the root systems of plants, and the decaying organic matter exemplify a fundamental aspect of sustainable agriculture by promoting the continuous cycling of essential nutrients within the ecosystem. Furthermore, the act of composting, driven by the natural process of bacterial decomposition, helps mitigate greenhouse gas emissions by reducing the volume of organic waste that would otherwise be sent to landfills. It also improves soil structure and aeration, thereby fostering healthier agricultural ecosystems that can better withstand the challenges posed by climate change. The understanding of these complex ecological dynamics highlights the importance of microbiome-assisted strategies in agricultural disease control, offering valuable insights into how farmers can leverage beneficial microorganisms effectively to promote optimal plant health and yield. This synergistic relationship between bacterial decomposers and other members of the ecosystem underscores the significance of their role in sustainable agricultural practices, ultimately contributing to food security and environmental health. By harnessing the power of these microorganisms, we can pave the way for more resilient agricultural systems that are capable of sustaining future generations.
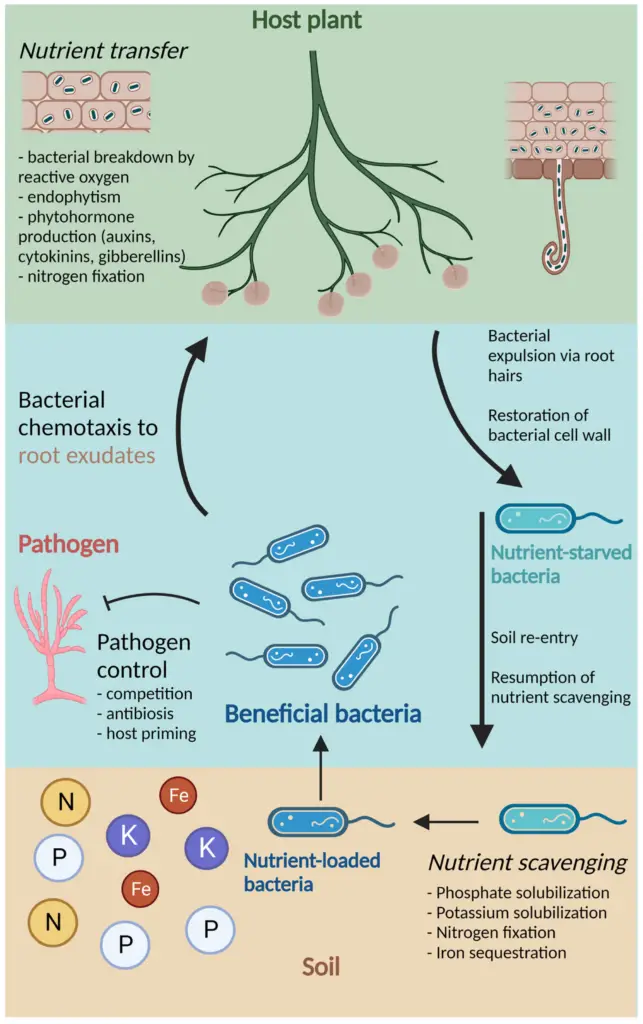
Image : Interactions between Host Plants and Beneficial Bacteria in Nutrient Transfer (The image provides a detailed diagram illustrating the interactions between host plants and beneficial bacteria in the context of nutrient transfer. It outlines processes such as bacterial breakdown by reactive oxygen, endophytism, and nitrogen fixation within the host plant. Additionally, it depicts the role of beneficial bacteria in soil nutrient scavenging, including phosphate solubilization, potassium solubilization, nitrogen fixation, and iron sequestration. Furthermore, the graphic illustrates how bacterial chemotaxis is directed towards root exudates and the mechanisms through which beneficial bacteria control pathogens, employing competition, antibiosis, and host priming. The relationships among these elements highlight the complex dynamics between plants and soil microorganisms that facilitate nutrient acquisition and disease management.)
Type of Bacteria | Role in Composting | Optimal Temperature (°F) | Optimal pH Level |
Actinobacteria | Decomposes complex organic matter, helps in breaking down cellulose. | 113-140 | 6.5-7.0 |
Pseudomonas | Degrades organic compounds, inhibits pathogens. | 68-104 | 6.0-8.0 |
Bacillus | Produces enzymes to decompose organic material, enhances nutrient availability. | 113-158 | 6.0-8.5 |
Bacterial Decomposers in Composting
III. Harmful Bacteria & Viruses in Agriculture
The presence of harmful bacteria and viruses in agriculture poses significant threats to crop health and food security, prompting the urgent need for effective management strategies that can mitigate such risks. Among these troublesome pathogens, bacterial blights, wilts, and viral infections can result in devastating crop losses, affecting not only the livelihoods of farmers but also creating ripple effects throughout global food supply chains. The economic impact of these diseases is considerable, with estimates indicating that billions of dollars are lost annually due to disease-related yield reductions, which can destabilize markets and lead to increased food costs for consumers. Furthermore, the reliance on conventional agrochemical solutions raises critical concerns surrounding the potential for harmful residue on produce, as well as the broader implications for ecological balance and biodiversity. To combat these multifaceted challenges, researchers are increasingly turning to innovative biocontrol methods that leverage natural antagonists to effectively manage pathogenic threats in agricultural systems. For instance, beneficial microorganisms, such as specific strains of bacteria and fungi, can suppress harmful pathogens through various mechanisms including competition for resources and the production of antimicrobial compounds that inhibit pathogen growth. This biocontrol approach not only promises a sustainable alternative to chemical treatments but also aligns agricultural practices with the principles of environmental stewardship and the creation of resilient ecosystems. As illustrated in various studies, understanding the intricate balance between harmful and beneficial microorganisms is key to advancing agricultural health and ensuring food security in the face of rising global population demands and changing climate conditions.
Pathogen | Disease | Affected Crops | Estimated Yield Loss (%) | Control Methods |
Xanthomonas campestris | Black rot in crucifers | Cabbage, Broccoli, Cauliflower | 20 | Crop rotation, resistant varieties |
Pseudomonas syringae | Bacterial halo blight | Beans | 30 | Sanitation, resistant varieties |
Potyvirus | Potato virus Y (PVY) | Potatoes, Tomatoes, Peppers | 50 | Use of virus-free seeds, insect control |
Fusarium oxysporum | Fusarium wilt | Tomatoes, Eggplants | 60 | Soil solarization, resistant varieties |
Tobacco mosaic virus (TMV) | Tobacco mosaic disease | Tobacco, Tomatoes, Peppers | 40 | Sanitation, resistant varieties |
Harmful Bacteria and Viruses in Agriculture
A. Bacterial Crop Diseases (e.g., Fire Blight, Bacterial Wilt)
Bacterial crop diseases, such as Fire Blight and Bacterial Wilt, pose significant challenges to agriculture, adversely affecting both plant health and crop yields on a wide scale. Fire Blight, which is caused by the bacterium Erwinia amylovora, primarily afflicts apple and pear trees, leading to devastating economic losses and frustrating many growers if left unchecked. The symptoms often result in blossom blight, shoot dieback, and canker formation, ultimately diminishing the viability of orchards. Similarly, Bacterial Wilt, caused by Ralstonia solanacearum, targets a wide range of crops, including tomatoes, potatoes, eggplants, and peppers, disrupting vascular systems which leads to wilting and potentially complete plant death. These diseases can spread rapidly under suitable environmental conditions, making them especially challenging to manage. The management of these diseases often involves the application of various chemical treatments; however, these methods carry significant risks of environmental degradation and the development of resistance among the bacterial populations. Consequently, the integration of biological control solutions has emerged as a promising and more environmentally friendly strategy for sustainable agriculture. Effective biocontrol agents, including certain beneficial bacteria and fungi, are being thoroughly investigated for their potential to suppress the growth of these harmful pathogens while simultaneously enhancing plant resilience and overall health. This multifaceted approach highlights the necessity of advancing sustainable practices to safeguard food systems against bacterial threats while minimizing ecological impact. The relevance of biological control methods is visually represented in research studies, which emphasizes the importance of utilizing natural organisms for effective pest management in agriculture, thereby promoting a healthier and more robust agricultural ecosystem.
Disease | Causative Agent | Affected Crops | Annual Economic Loss (USD) | Symptoms |
Fire Blight | Erwinia amylovora | Apples, Pears | 100000000 | Wilting and browning of blossoms and shoots |
Bacterial Wilt | Ralstonia solanacearum | Tomatoes, Potatoes, Eggplants | 450000000 | Wilting, stunted growth, and plant collapse |
Bacterial Blight | Pseudomonas syringae | Beans, Peas | 70000000 | Water-soaked lesions on leaves |
Bacterial Crop Diseases and Their Impact
B. Viral Crop Infections (e.g., Tobacco Mosaic Virus)
Viral crop infections represent a significant challenge in agriculture, with the Tobacco Mosaic Virus (TMV) serving as a prominent example of the detrimental impacts caused by such pathogens. TMV, notorious for its ability to infect a wide array of host plants, particularly targets tobacco and tomatoes, which results in considerable yield losses, along with a marked reduction in the overall quality of affected crops. The spread of this virus primarily occurs through mechanical means, such as contact from handling infected plants or through the use of contaminated tools, and it can retain its viability on surfaces for extended periods, which complicates management efforts and increases the potential for outbreaks. The symptoms associated with TMV infection, which include mottled leaves and diminished photosynthetic efficiency, signify an urgent need for effective and timely control strategies to mitigate the impact of this virus on agricultural productivity. In response to these formidable challenges, researchers and agricultural scientists are increasingly exploring biocontrol solutions, including the development and use of resistant cultivars and the application of biological pesticides, which may help to alleviate the adverse effects of viral infections without the heavy reliance on synthetic chemicals that can be harmful to the environment. The adoption of such innovative methods not only promotes sustainable agricultural practices but also holds significant potential for improving crop resilience in the face of future viral threats. This is further evidenced by the observed variations in TMV resistance across different strains of tobacco plants, highlighting the importance of biodiversity and resistance breeding in the ongoing fight against viral crop infections. Ultimately, these efforts will be crucial in creating a more robust agricultural system capable of withstanding the challenges posed by plant viruses like TMV.
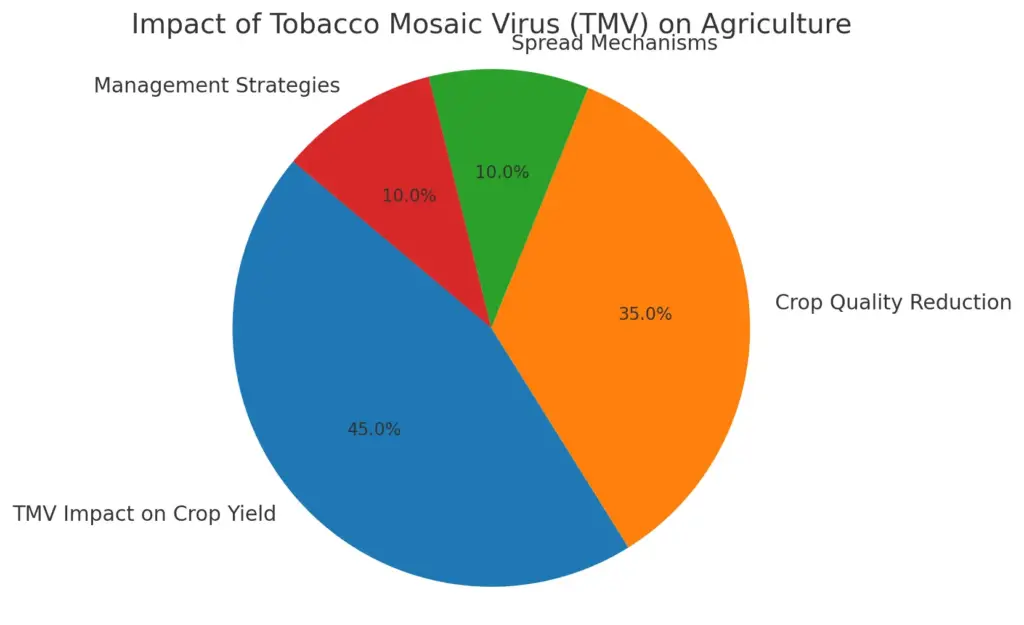
This pie chart illustrates the impacts of Tobacco Mosaic Virus (TMV) on agriculture, with 45% of crop yield losses attributed to TMV infections, 35% reflecting reduced crop quality, 10% identifying the spread mechanisms, and 10% outlining the management strategies being explored.
Virus | Host Plants | Symptoms | Transmission | Impact on Yield (%) | Control Methods |
Tobacco Mosaic Virus (TMV) | Tobacco, Tomatoes, Peppers | Mosaic pattern on leaves, Stunted growth, Leaf curling | Mechanical means, Insect vectors | 10-30% | Resistant varieties, Crop rotation, Insect control |
Cucumber Mosaic Virus (CMV) | Cucumbers, Lettuce, Beans | Mosaic and mottling on leaves, Yellowing, Stunted growth | Aphids, Seedborne | 20-50% | Aphid control, Resistant varieties, Removing infected plants |
Potato Virus Y (PVY) | Potatoes, Tomatoes, Peppers | Leaf rolling, Mottling, Wilting | Aphids, Potato tubers | 30-60% | Use of certified seed, Insect management |
Viral Crop Infections Data
C. Zoonotic Viruses and the Threat to Livestock
The emergence of zoonotic viruses presents a significant and multifaceted threat to livestock, posing risks not only to animal health but also to food security and public health on a global scale. Diseases such as avian influenza and porcine reproductive and respiratory syndrome have compellingly demonstrated that viruses can rapidly decimate livestock populations across vast regions, leading to severe economic repercussions that can destabilize local and international markets. The economic implications extend beyond the immediate losses in animal health; they can also affect farmers’ livelihoods, food supplies, and pricing. These zoonotic agents often originate in wildlife, exploiting agricultural environments as critical pathways to spread into domestic animal populations. Poor biosecurity measures, coupled with intensive farming practices that may overlook preventive precautions, further exacerbate these risks by facilitating the transmission of pathogens among different animal populations. Furthermore, the complex interactions between livestock and their microbial ecosystems can create conditions that favor the emergence of new strains capable of crossing species barriers, thereby intensifying the urgency and necessity for vigilant monitoring and control strategies. Additionally, government regulations and global trade policies often impact the ways we manage and respond to these threats. Visual representations of these dynamics can enhance understanding, particularly images that depict these interactions, such as , which illustrate the biological interactions underlying pathogen spread and resistance in agricultural settings. These visual aids serve to emphasize the critical need for integrated management approaches that are proactive rather than reactive, ensuring the health of livestock and safeguarding food systems against future zoonotic threats.
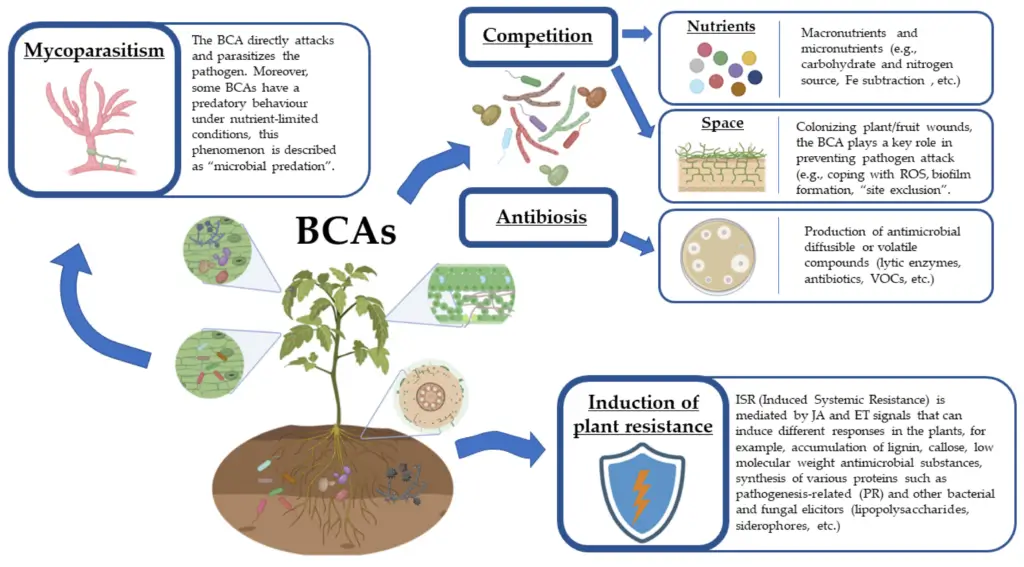
Image : Mechanisms of Action of Biological Control Agents in Plant Systems (The image illustrates the mechanisms by which biological control agents (BCAs) interact with plant systems to mitigate pathogen effects. It categorizes these mechanisms into mycoparasitism, competition, antibiosis, nutrient acquisition, space colonization, and induction of plant resistance. Each category is visually represented with relevant icons and concise descriptions emphasizing their roles in enhancing plant health and resistance against pathogens. The visual aids support the understanding of complex biological interactions involved in agricultural practices, particularly in the field of plant pathology and microbiology.)
Virus | Transmission | Species Affected | Impact | Year of Latest Outbreak |
African Swine Fever Virus (ASFV) | Direct contact with infected animals, contaminated feed, and equipment | Domestic pigs and wild boars | High mortality rates; detrimental economic impact on pig farming | 2023 |
Avian Influenza (H5N1) | Droplets, contaminated surfaces, and materials | Domestic and wild birds, can infect mammals | High mortality rates in birds; potential for zoonotic transmission | 2022 |
Bovine Viral Diarrhea Virus (BVDV) | Direct contact, bodily fluids, and contaminated environment | Cattle | Reproductive failures, respiratory issues; economic losses in beef and dairy sectors | 2023 |
Blue Tongue Virus (BTV) | Insect vectors (biting midges) | Cattle, sheep, goats, and deer | Causes fever, swelling, and can result in death; impacts sheep farming | 2022 |
Foot-and-Mouth Disease Virus (FMDV) | Direct contact with infected animals, respiratory secretions | Cattle, pigs, sheep, and goats | Highly contagious; severe economic impact due to livestock loss and trade restrictions | 2023 |
Zoonotic Viruses Affecting Livestock
IV. Biocontrol: Using Microbes to Protect Crops
The utilization of biocontrol through microbes has emerged as a crucial strategy in sustainable agriculture, offering an eco-friendly alternative to chemical pesticides that can harm the environment and human health. Beneficial microorganisms, such as bacteria and fungi, play an essential role in the suppression of plant pathogens through a variety of effective mechanisms. These include competition for essential resources, the production of inhibitory compounds that disrupt pathogen growth, and the induction of plant resistance mechanisms that prompt a more robust defensive response from the crops themselves. For instance, the beneficial presence of these microbes can significantly enhance the crops’ ability to withstand a range of diseases, thereby boosting overall yield and overall plant health. This innovative method not only minimizes the ecological footprint of agricultural practices by reducing reliance on synthetic chemicals, but it also fosters the development of a resilient agricultural ecosystem that can adapt to changing conditions. Furthermore, as illustrated in [citeX], biocontrol strategies incorporate a diverse array of approaches, ensuring a comprehensive defense against pests while simultaneously preserving beneficial species that contribute to soil health and biodiversity. By aligning with the principles of integrated pest management, which combines various strategies for pest control, microbial biocontrol solutions exemplify the promise of sustainable agriculture in effectively addressing the complex challenges faced by modern farming, such as climate change and pest resistance. In this way, microbes not only serve as a powerful tool for protecting crops but also contribute to the long-term viability and sustainability of agricultural systems worldwide.
Agent | Target Pest | Effectiveness (%) | Application Volume (liters/ha) | Year |
Bacillus thuringiensis (Bt) | Helicoverpa zea (corn earworm) | 90 | 50 | 2022 |
Trichoderma spp. | Fusarium wilt | 75 | 30 | 2022 |
Pseudomonas fluorescens | Pythium root rot | 85 | 25 | 2022 |
Beauveria bassiana | Aphids | 70 | 40 | 2022 |
Mycobacterium spp. | Whiteflies | 80 | 35 | 2022 |
Biocontrol Agents in Agriculture
A. Bacteriophages as Alternatives to Antibiotics
Bacteriophages, or phages, represent a promising and innovative alternative to conventional antibiotics, particularly within the field of agriculture, where bacterial diseases pose a significant threat to both crop yield and quality. These specialized viruses have the remarkable ability to specifically target bacterial pathogens, utilizing a unique lytic cycle that effectively destroys harmful bacteria while sparing the beneficial microflora essential for healthy soil and plant development. The application of bacteriophages can significantly reduce the reliance on chemical antibiotics, which have contributed to the concerning rise of antibiotic resistance and various forms of ecological disruption. Unlike traditional antimicrobial agents, phages exhibit a high degree of specificity, which minimizes non-target effects and promotes a more balanced microbial ecosystem, allowing beneficial microbes to thrive while effectively combating pathogenic strains. Furthermore, phages can be seamlessly integrated into biocontrol strategies, thus enhancing overall plant health and resilience against disease outbreaks. Given the clear and pressing global issue of antibiotic resistance, the employment of phages as a biocontrol solution addresses critical challenges in disease management and aligns harmoniously with sustainable agricultural practices. This innovative approach serves as a crucial component in the transformation of agricultural pest control and disease management strategies, where the potential of biological control methods is increasingly recognized and embraced. As illustrated in the concept of biocontrol approaches in various agricultural studies, the ongoing research into phage therapy offers valuable insights and practical applications, paving the way for a future in which sustainable farming practices can effectively coexist with the necessary fight against bacterial pathogens, ensuring food security and environmental health.
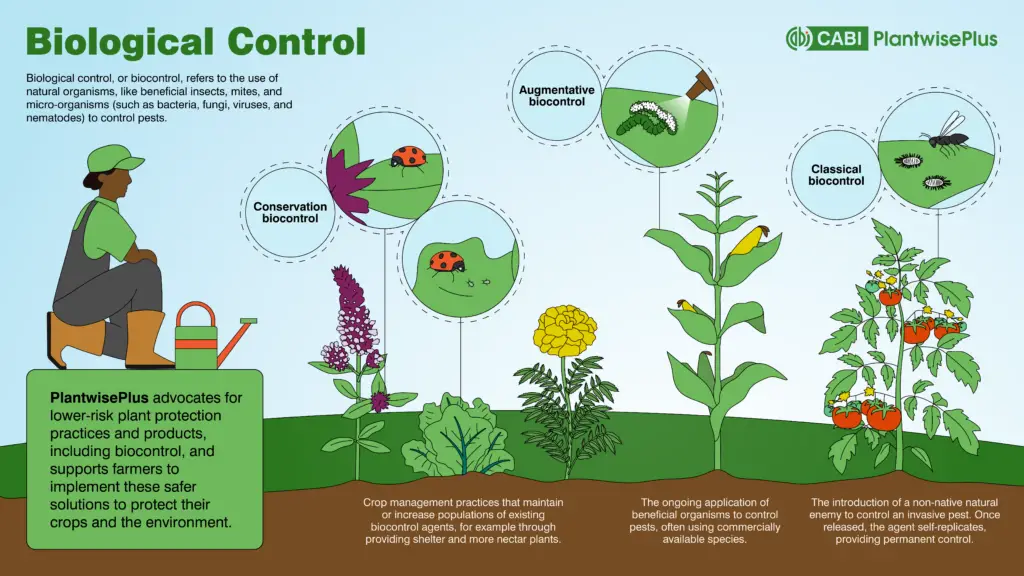
Image : Illustration of Biological Control Methods in Agriculture (The image illustrates the concept of biological control (biocontrol) in pest management, specifically emphasizing the use of natural organisms such as beneficial insects, mites, and microorganisms to combat pests. It categorizes different approaches to biocontrol: conservation biocontrol, augmentative biocontrol, and classical biocontrol. Each category is visually represented with icons depicting various organisms and crops, alongside a gardener implementing these practices. The visual also includes a description advocating for lower-risk plant protection methods, underscoring the importance of integrating these biological techniques in agricultural practices for environmental sustainability.)
Study | Organism Targeted | Bacteriophage Strain | Efficacy Rate (%) | Application Method |
Rosenberg et al. (2020) | Escherichia coli | ΦX174 | 85 | Soil treatment |
Katsuda et al. (2021) | Salmonella enterica | Salmonella phage A3 | 75 | Irrigation water |
Merril et al. (2019) | Pseudomonas syringae | ΦPS18 | 90 | Foliar spray |
Latif et al. (2022) | Xanthomonas campestris | φX174G | 80 | Seed treatment |
Dastjerdi et al. (2018) | Listeria monocytogenes | ListShield | 95 | Post-harvest treatment |
Bacteriophage Efficacy in Agricultural Applications
B. How Beneficial Microbes Outcompete Harmful Ones
Beneficial microbes play a crucial role in outcompeting harmful ones through various mechanisms that enhance plant health and resilience in several significant ways. These microorganisms effectively establish themselves in the rhizosphere, where they form complex, symbiotic interactions with plants that can inhibit the growth of pathogenic species by utilizing the available nutrients and space that would otherwise support harmful organisms. For instance, beneficial bacteria can produce antagonistic compounds that directly suppress pathogens, preventing their establishment and proliferation, or they can induce systemic resistance within the plants they colonize, effectively priming the plant’s immune system for future threats. Additionally, certain microbial communities can enhance nutrient acquisition through the solubilization of essential minerals, such as phosphorus and potassium, which are critical for plant development, thereby promoting overall plant growth and vigor. This competitive advantage is multifaceted; it not only aids in plant nutrition and development but also strengthens the plant’s defenses against adversarial microbes, creating a more robust ecosystem. The significance of these beneficial interactions is visually encapsulated in the flowchart depicting the intricate interplay between soil-borne plant pathogens and their management strategies. This representation effectively illustrates the vital role that beneficial microbes play in establishing a natural defense system for plants, demonstrating how they are essential for sustainable agricultural practices and integrated pest management solutions. By fostering the growth of these helpful microorganisms, we can promote healthier crops, reduce the reliance on chemical pesticides, and enhance the overall sustainability of agricultural systems.
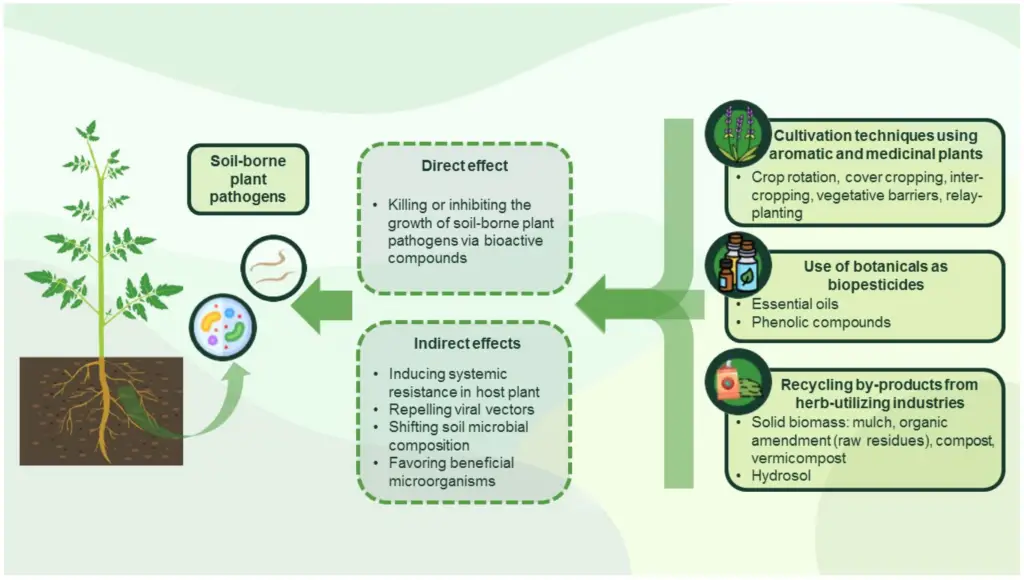
Image : Strategies for Managing Soil-borne Plant Pathogens in Agriculture (The image presents a flowchart detailing the effects of soil-borne plant pathogens and their management through various techniques. At the center, it highlights soil-borne plant pathogens and categorizes their management into direct and indirect effects. The direct effects are described as killing or inhibiting pathogen growth through bioactive compounds, while the indirect effects include strategies such as inducing systemic resistance in host plants, repelling viral vectors, shifting soil microbial composition, and favoring beneficial microorganisms. The right side of the flowchart showcases three approaches: ‘Cultivation techniques using aromatic and medicinal plants,’ which includes practices like crop rotation and cover cropping; ‘Use of botanicals as biopesticides,’ featuring essential oils and phenolic compounds; and ‘Recycling by-products from herb-utilizing industries,’ which highlights methods for utilizing solid biomass and hydrosol. Overall, the flowchart serves as a comprehensive overview of sustainable agricultural practices aimed at mitigating the impact of soil-borne plant pathogens.)
Microbe Name | Type | Beneficial Effect | Effectiveness (%) | Crops Impacted |
Rhizobium | Bacteria | Nitrogen Fixation | 75 | Legumes |
Bacillus thuringiensis | Bacteria | Pest Control | 85 | Various |
Trichoderma | Fungi | Soil Health Improvement | 70 | Vegetables |
Mycorrhizae | Fungi | Enhanced Nutrient Uptake | 80 | Perennials and Trees |
Lactobacillus | Bacteria | Promotion of Plant Growth | 60 | Fruits and Vegetables |
Beneficial Microbes in Agriculture
C. Genetic Engineering for Disease-Resistant Crops
The advent of genetic engineering has ushered in a transformative era for agriculture, particularly in the development of disease-resistant crops. By utilizing advanced techniques that modify the genetic makeup of plants at a molecular level, researchers can enhance natural resistance mechanisms against a myriad of pathogens that threaten crop health. These genetically engineered crops not only effectively reduce reliance on chemical pesticides, which minimizes the environmental impact associated with their use, but they also bolster food security significantly by increasing yields and reducing crop losses that occur due to devastating disease outbreaks. Furthermore, the integration of beneficial microbes within these engineered systems can create synergistic effects that promote plant health and resilience, thus enhancing the efficacy of biological control methods that are deemed more environmentally friendly. For instance, the image depicting phytomicrobiome-based strategies for plant disease control accentuates the importance of both genetic modifications and microbial interactions in establishing a harmonious relationship between crops and their associated microbiomes. This symbiosis is crucial as it can lead to improved nutrient uptake, better stress responses, and overall plant vigor. By fostering these innovative developments, agricultural practices can shift towards more sustainable and resilient methods, aligning with the broader goals of environmental stewardship and economic viability, ensuring that farming systems can withstand the challenges posed by climate change and an ever-growing global population. As we advance, the continual exploration of genetic engineering promises to yield even greater insights and advancements, equipping farmers with the tools they need to thrive in an increasingly uncertain agricultural landscape.
Crop | Trait | Year Introduced | Increase in Yield (%) | Reduction in Pesticide Use (%) |
Corn | Bt (Bacillus thuringiensis) Resistance | 1996 | 15 | 32 |
Soybean | Herbicide Tolerance | 1996 | 10 | 20 |
Cotton | Bt (Bacillus thuringiensis) Resistance | 1997 | 13 | 40 |
Papaya | Rainbow Variety for Ringspot Virus | 1998 | 50 | 60 |
Potato | Late Blight Resistance | 2022 | 20 | 70 |
Genetic Engineering Impact on Crop Disease Resistance
V. Conclusion – The Future of Microbial Agriculture
As agriculture faces mounting challenges from climate change, pest resistance, and food security, the future of microbial agriculture emerges as a beacon of hope for farmers and consumers alike. Harnessing bacteria and viruses for biocontrol offers sustainable methods to enhance crop resilience while minimizing reliance on chemical pesticides that can harm the environment and human health. This essential transition towards microbial solutions not only benefits the environment by reducing chemical runoff and pollution but also improves agricultural productivity by promoting soil health and biodiversity, which are critical for long-term sustainability. Future research must prioritize the development and application of beneficial microorganisms, focusing on their ability to optimize nutrient uptake and enhance pathogen resistance in crops, which can ultimately lead to increased yields and reduced input costs for farmers. Moreover, collaboration among scientists, farmers, and policymakers will play a pivotal role in facilitating the integration of microbial technologies into conventional farming practices. This collaboration will ensure that these innovative solutions are widely accessible to all farmers, particularly smallholders, thereby enhancing food systems sustainability on a global scale. The importance of visualizing these strategies can be effectively illustrated through advanced research, which highlights phytomicrobiome-based approaches to plant disease control, showcasing the intricate interconnectedness of microorganisms in fostering plant health and resilience. Thus, a commitment to microbial agriculture signifies a pivotal advancement in the quest for sustainable and resilient food production systems, offering a promising pathway to address the urgent challenges of our time while paving the way for a greener, more secure future in agriculture.
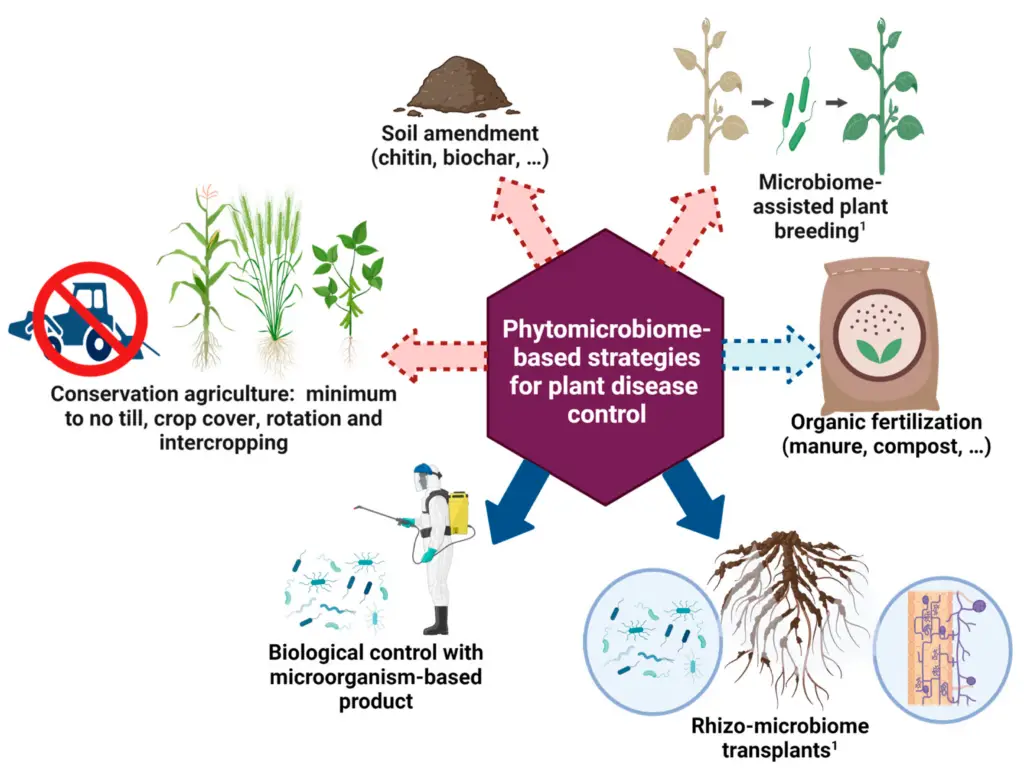
Image : Diagram of Phytomicrobiome-Based Strategies for Plant Disease Control (The image presents a diagram illustrating various phytomicrobiome-based strategies for plant disease control, organized around a central hexagon labeled “Phytomicrobiome-based strategies for plant disease control.” It features multiple approaches that promote sustainable agriculture, including conservation agriculture practices (e.g., no tillage, crop cover, rotation, intercropping), soil amendments (like chitin and biochar), microbiome-assisted breeding techniques, organic fertilization methods (such as manure and compost), and biological control utilizing microorganism-based products. Additionally, it highlights the concept of rhizo-microbiome transplants, emphasizing the role of beneficial microorganisms in enhancing plant health. The inclusion of icons and annotations aids in visual comprehension of these interconnected strategies.)
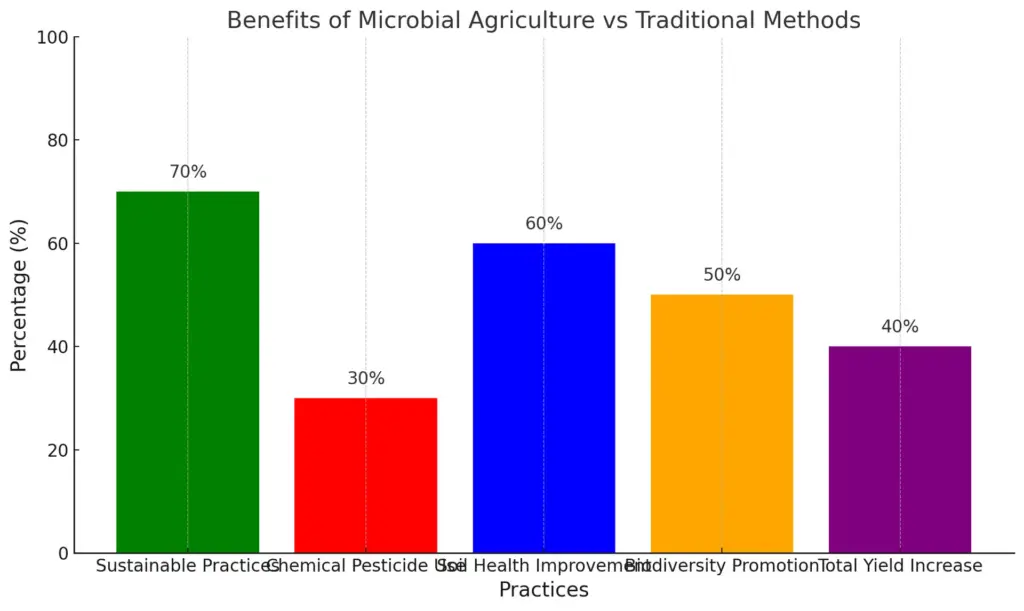
This bar chart illustrates the benefits of microbial agriculture compared to traditional methods. It highlights the significant emphasis on sustainable practices (70%) versus chemical pesticide use (30%). Additionally, it showcases improvements in soil health (60%) and biodiversity promotion (50%), which contribute to total yield increases (40%). This aligns with the advantages of biocontrol solutions in agriculture and their potential for sustainable food production.
REFERENCES
- William D. Hutchison. ‘Integrated Pest Management.’ Concepts, Tactics, Strategies and Case Studies, Edward B. Radcliffe, Cambridge University Press, 1/1/2009
- Peter G. Mason. ‘Biological Control.’ Global Impacts, Challenges and Future Directions of Pest Management, Csiro Publishing, 10/1/2021
- Nicholas J. Mills. ‘Biological Control.’ George E. Heimpel, Cambridge University Press, 4/3/2017
- Sudisha Jogaiah. ‘Biocontrol Agents and Secondary Metabolites.’ Applications and Immunization for Plant Growth and Protection, Woodhead Publishing, 11/13/2020
- Corné M.J. Pieterse. ‘Harnessing Useful Rhizosphere Microorganisms for Pathogen and Pest Biocontrol.’ Aurelio Ciancio, Frontiers Media SA, 1/10/2017
- Board on Global Health. ‘Improving Food Safety Through a One Health Approach.’ Workshop Summary, Institute of Medicine, National Academies Press, 9/10/2012
- Health and Medicine Division. ‘Global Health Impacts of Vector-Borne Diseases.’ Workshop Summary, National Academies of Sciences, Engineering, and Medicine, National Academies Press, 10/21/2016
- Richa Salwan. ‘Molecular Aspects of Plant Beneficial Microbes in Agriculture.’ Vivek Sharma, Elsevier, 3/12/2020
- Pankaj Kumar. ‘Rhizosphere Engineering.’ Ramesh Chandra Dubey, Academic Press, 2/15/2022
- Robert May. ‘Population Biology of Infectious Diseases.’ Report of the Dahlem Workshop on Population Biology of Infectious Disease Agents Berlin 1982, March 14 – 19, R.M. Anderson, Springer Berlin Heidelberg, 12/8/2011
- Pankaj Kumar Mishra. ‘Agriculturally Important Microbes for Sustainable Agriculture.’ Volume 2: Applications in Crop Production and Protection, Vijay Singh Meena, Springer, 9/20/2017
- Jessica Snyder Sachs. ‘Good Germs, Bad Germs.’ Health and Survival in a Bacterial World, Macmillan + ORM, 9/30/2008
- Abel Lajtha. ‘Handbook of Neurochemistry and Molecular Neurobiology.’ Neural Protein Metabolism and Function, Springer Science & Business Media, 3/26/2007
- Calvin O. Qualset. ‘Biodiversity in Agroecosystems.’ Wanda W. Collins, CRC Press, 8/14/1998
- Alistair McCleery. ‘An Introduction to Book History.’ David Finkelstein, Routledge, 3/13/2006
Image References:
- Image: Illustration of Biological Control Methods in Agriculture, Accessed: 2025.https://blog.plantwise.org/wp-content/uploads/sites/7/2024/07/CABI_BIOCONTROL_INFOGRAPHIC_08.07.2024-1.png
- Image: Interactions between Host Plants and Beneficial Bacteria in Nutrient Transfer, Accessed: 2025.https://www.mdpi.com/plants/plants-12-00400/article_deploy/html/images/plants-12-00400-g001.png
- Image: Mechanisms of Action of Biological Control Agents in Plant Systems, Accessed: 2025.https://www.mdpi.com/horticulturae/horticulturae-08-00577/article_deploy/html/images/horticulturae-08-00577-g001.png
- Image: Overview of Biological Control Methods in Pest Management, Accessed: 2025.https://bioprotectionportal.com/wp-content/uploads/2024/07/biological-control-infog-1024×576.png?x76044
- Image: Strategies for Managing Soil-borne Plant Pathogens in Agriculture, Accessed: 2025.https://www.mdpi.com/plants/plants-12-00706/article_deploy/html/images/plants-12-00706-g001.png
- Image: Diagram of Phytomicrobiome-Based Strategies for Plant Disease Control, Accessed: 2025.https://www.mdpi.com/plants/plants-12-02736/article_deploy/html/images/plants-12-02736-g001.png