Bacteria and Viruses in Biotechnology: Genetic Engineering and Bioengineering Applications
Table of Contents
I. Introduction to Microbial Biotechnology
Microbial biotechnology represents a transformative frontier in the life sciences, harnessing the capabilities of bacteria and viruses in innovative applications that span diverse industries, thereby bridging the gap between biological research and practical implementation. This rapidly evolving field capitalizes on the inherent biological processes of microorganisms to develop effective solutions for pressing challenges such as disease management, environmental sustainability, and industrial efficiency, offering a broad array of possibilities for advancement. By genetically engineering microbes, researchers can enhance production capabilities of valuable compounds, such as pharmaceuticals and biofuels, while also devising novel approaches for biodegradation and bioremediation, ultimately leading to cleaner processes and reduced ecological footprints. The integration of synthetic biology into microbial applications further expands the potential for tailored cell functions and intricate biochemical pathways, exemplifying the convergence of biological and engineering principles, which in turn accelerates the pace of discovery and innovation. The multifaceted applications of microbial engineering makes it a pivotal reference point in understanding the broad relevance of microbial biotechnology across different fields, including agriculture, medicine, and environmental sciences. Through these advancements, microbial biotechnology not only promises to drive innovation in traditional practices but also paves the way for sustainable solutions to global problems, emphasizing the vital role that microorganisms play in future technological advancements and the need for continued research and investment in this promising area of study. As society faces increasing challenges, the need for innovative approaches becomes even more critical, highlighting the importance of microbial biotechnology in securing a resilient and sustainable future.
20 key points supporting Microbial Biotechnology:
Category | Key Points |
---|---|
Medical Applications | 1. Microbes are engineered to produce antibiotics, reducing reliance on traditional sources. 2. Bacteria such as E. coli and Saccharomyces cerevisiae are used to synthesize insulin and other therapeutic proteins. 3. Bacteriophage therapy offers an alternative to antibiotics for treating resistant bacterial infections. 4. Microbial vaccines, such as recombinant DNA vaccines, improve immunization strategies. |
Industrial Biotechnology | 5. Microbes play a key role in the fermentation industry, producing bioethanol, amino acids, and organic acids. 6. Engineered microbes are used in bioleaching to extract metals from low-grade ores. 7. Industrial enzymes derived from microbes (e.g., proteases, lipases) improve food processing and textile manufacturing. 8. Microbial biosensors detect environmental pollutants and contaminants. |
Agricultural Advancements | 9. Beneficial bacteria like Rhizobium and Azotobacter promote nitrogen fixation in plants, reducing the need for synthetic fertilizers. 10. Genetically modified bacteria enhance soil fertility and plant resistance to pathogens. 11. Microbial biopesticides (e.g., Bacillus thuringiensis) provide eco-friendly pest control solutions. 12. Microbial probiotics enhance livestock health and growth efficiency. |
Environmental Sustainability | 13. Microorganisms assist in bioremediation, breaking down pollutants like oil spills and heavy metals. 14. Microbial fuel cells generate electricity from organic waste materials. 15. Engineered microbes can capture and convert carbon dioxide, mitigating climate change effects. 16. Microbial plastic degradation offers solutions for reducing plastic waste. |
Synthetic Biology & Genetic Engineering | 17. CRISPR technology allows precise genetic modifications in microbes for enhanced productivity. 18. Synthetic pathways engineered in microbes enable the biosynthesis of complex chemicals. 19. Microbial genome editing enhances metabolic engineering for improved bio-manufacturing. 20. The integration of AI and machine learning accelerates microbial strain optimization for industrial applications. |
Year | Global Market Size (Billion USD) | Growth Rate (%) | Major Applications |
2021 | 18.2 | 9.1 | Pharmaceuticals, Agriculture, Industrial Biotechnology |
2022 | 20 | 9.8 | Pharmaceuticals, Agriculture, Industrial Biotechnology |
2023 | 22.1 | 10.5 | Pharmaceuticals, Agriculture, Industrial Biotechnology |
Microbial Biotechnology Data
A. How Bacteria & Viruses Are Used in Scientific Advancements
Bacteria and viruses are pivotal to advancements in biotechnology, particularly in genetic engineering and bioengineering applications. Engineered bacterial strains have become indispensable for synthesizing complex pharmaceuticals, such as insulin and monoclonal antibodies, thereby revolutionizing the treatment options for various ailments. These tailored microorganisms not only improve the efficiency of pharmaceutical production but also allow for the creation of more effective and targeted therapies, ultimately leading to better patient outcomes. Additionally, bacteriophages, which are viruses that infect bacteria, are employed in targeted therapies to combat antibiotic-resistant infections, bringing a new dimension to infectious disease control. This innovative approach not only highlights the adaptability of these viruses but also represents a promising strategy in the ongoing battle against the growing threat of antibiotic resistance. The versatility of these microorganisms extends to environmental applications; genetically modified bacteria can reduce pollution by degrading toxic compounds in waste, thus contributing to bioremediation efforts. Such advancements are crucial in restoring damaged ecosystems and making contaminated sites safe for both human and wildlife habitation. Furthermore, the emerging field of synthetic biology leverages bacteria to create novel biosensors capable of detecting specific pathogens or environmental toxins, enhancing public health safety. These biosensors can provide rapid and accurate results, allowing for timely intervention in potential health crises. Ultimately, the integration of bacteria and viruses into scientific advancement underscores their indispensable role in addressing contemporary global challenges in health and the environment, as illustrated in [citeX]. The continued exploration of these microorganisms promises to unlock new possibilities and solutions for some of the most pressing issues facing humanity today.
Application | Description | Year Introduced | Success Rate (%) | Example |
Gene Therapy | Using modified viruses to deliver genes into human cells. | 1990 | 70 | Adenovirus for cystic fibrosis treatment |
CRISPR Technology | Utilizing bacteria’s natural defense system to edit genes. | 2012 | 90 | Editing genes in plants for higher yields |
Vaccine Development | Employing live attenuated viruses to create immune responses. | 1796 | 95 | Vaccines like MMR (Measles, Mumps, Rubella) |
Bioremediation | Using bacteria to degrade environmental contaminants. | 1980 | 80 | Bacteria used to clean oil spills |
Production of Insulin | Genetically modified bacteria producing human insulin. | 1982 | 99 | Recombinant DNA technology |
Synthetic Biology | Designing and constructing new biological parts and systems. | 2000 | 85 | Creating bacteria that can produce biofuels |
Scientific Advancements Through Bacteria and Viruses
II. Bacteria in Biotechnology
Bacteria play a pivotal role in biotechnology, demonstrating their immense potential in various applications, including pharmaceuticals, agriculture, and environmental remediation. One of the most significant advancements in this area is the use of genetically engineered bacteria to produce essential therapeutic proteins, enzymes, and even vaccines, showcasing the versatility and efficiency of these microorganisms. The integration of synthetic biology allows scientists to modify bacterial genomes tactically and purposefully, thereby enhancing their capabilities to synthesize complex organic compounds that can be utilized in diverse industrial applications. For instance, distinct genetic circuits can be engineered within specific bacterial strains to facilitate the production of biopharmaceuticals, as illustrated in the workflow for Multiplexed Automated Genome Engineering, which highlights the optimization of these processes for non-model hosts, making it feasible to harness the unique biochemical properties of various bacterial species. Furthermore, the remarkable adaptability of bacteria enables their use in a multitude of bioengineering applications, effectively addressing critical challenges such as antibiotic resistance that poses a threat to public health and environmental pollution, which is detrimental to ecosystems. As our understanding of bacterial genetic pathways expands with ongoing research, their applications in biotechnology continue to evolve and become more refined, underscoring their invaluable contributions and significance to modern science and industry. This ongoing exploration not only emphasizes the importance of bacteria in developing innovative solutions but also paves the way for groundbreaking discoveries that could transform numerous sectors, ensuring that these microorganisms remain at the forefront of biotechnological advancement.
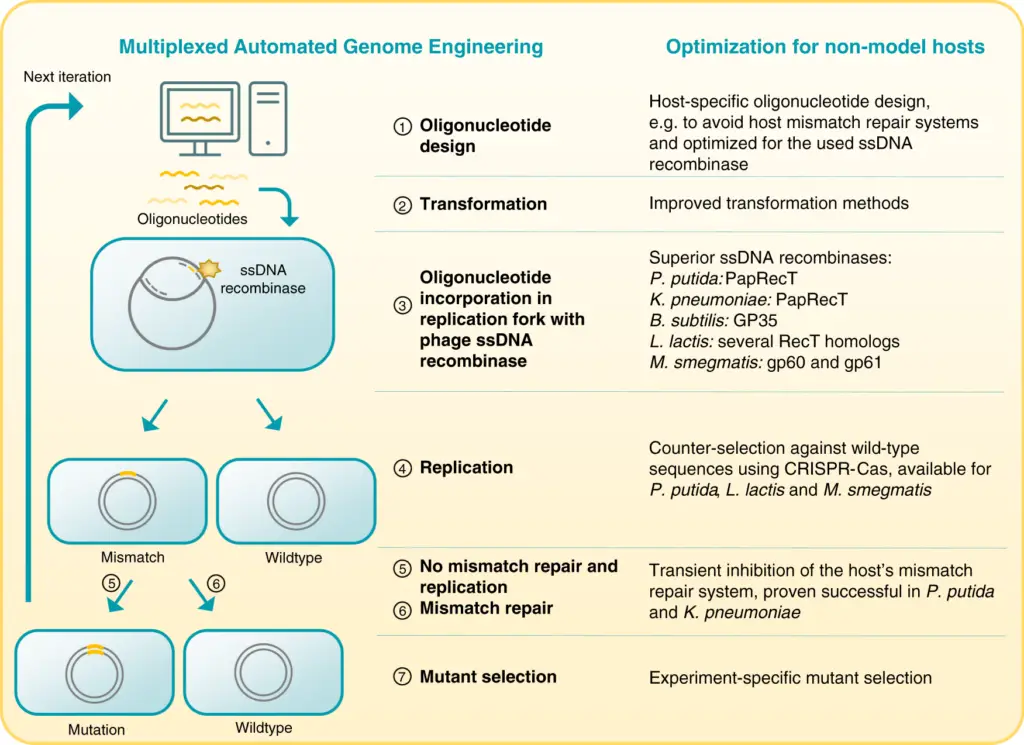
Image – Workflow for Multiplexed Automated Genome Engineering Optimizations in Non-Model Hosts (The image presents a detailed workflow for Multiplexed Automated Genome Engineering, specifically focusing on optimization techniques for non-model hosts. It illustrates the process starting from oligonucleotide design, transformation, and oligonucleotide incorporation into the replication fork with the involvement of phage ssDNA recombinase. The steps include the identification of mismatches and wildtype sequences, successful replication attempts, and selective mutant identification strategies. It lists superior ssDNA recombinases applicable to different non-model bacterial hosts, including Pseudomonas putida, Klebsiella pneumoniae, and others, while emphasizing methods for bypassing mismatch repair systems, indicative of innovations in genetic engineering methods for advancing microbial biotechnology.)
Application | Description | Example Organism | Year Introduced |
Bioremediation | Using bacteria to clean up environmental contaminants. | Pseudomonas putida | 1992 |
Biopesticides | Bacteria used to control agricultural pests. | Bacillus thuringiensis | 1980 |
Genetic Engineering | Bacteria as vectors for gene cloning. | Escherichia coli | 1973 |
Pharmaceutical Production | Using bacteria to produce insulin and antibiotics. | Escherichia coli | 1982 |
Industrial Enzymes | Bacteria used to produce enzymes for industrial processes. | Thermophilic bacteria | 1990 |
Bacteria Applications in Biotechnology
A. The Role of E. coli in Genetic Engineering
Escherichia coli (E. coli) serves as a cornerstone organism in the field of genetic engineering due to its rapid growth, well-characterized genetics, and ability to easily incorporate foreign DNA. This versatile bacterium is frequently employed as a host for cloning vectors, which are pivotal for amplifying specific genes and producing recombinant proteins essential for various applications. By utilizing techniques such as restriction enzyme digestion and ligation, scientists can insert desired genetic material into plasmids, which E. coli can then replicate efficiently, thereby ensuring a robust supply of the target DNA. Furthermore, the accessibility of expression systems in E. coli allows for the production of proteins that may be difficult to obtain from higher organisms, which can be impractical or costly to cultivate. The simplicity of manipulating E. coli, combined with its capacity for transformation—whereby it can uptake foreign DNA and incorporate it into its own genetic material—makes it an indispensable tool in both basic research and applied biotechnology. This ease of use has led to its widespread adoption in laboratories worldwide, making it a fundamental component in the cloning and expression of genes from a myriad of different organisms. By showcasing these factors, E. coli underpins much of the ongoing innovation in genetic engineering, which continues to revolutionize applications in medicine, agriculture, and industry. This relationship can be visually represented in numerous educational graphics and instructional materials, illustrating how E. coli acts as a vital workhorse in the genetic engineering landscape, facilitating discoveries and advancements that can potentially alter the way we approach problems related to health and sustainability.
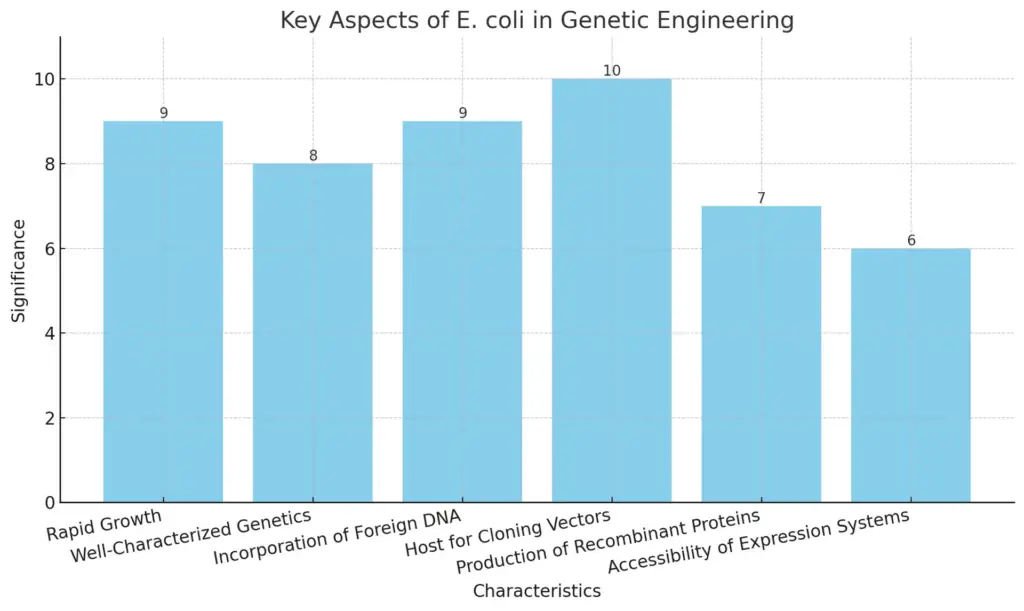
This bar chart illustrates the key aspects of E. coli’s role in genetic engineering, highlighting its advantages such as rapid growth and its status as a host for cloning vectors. Each value represents the significance of a particular characteristic, providing insights into why E. coli is a preferred organism in biotechnology.
Application | Description | Reliability | Example | YearFirstUsed |
Protein Production | E. coli is widely used to produce recombinant proteins, often used in pharmaceuticals. | High | Insulin production | 1982 |
Gene Cloning | E. coli serves as a host for cloning human genes due to its fast growth and simplicity. | High | Cloning of the human growth hormone gene | 1978 |
Genetic Engineering | E. coli is modified to create genetically engineered strains for various research. | High | Creation of knockout strains for gene function studies | 1990 |
Vaccine Production | E. coli is used in the production of vaccines through recombinant DNA technology. | Moderate | Production of hepatitis B vaccine | 1986 |
Bioremediation | E. coli can be engineered to degrade environmental pollutants. | Emerging | Degradation of toluene and other hydrocarbons | 2000 |
E. coli Applications in Genetic Engineering
B. How Bacteria Are Used to Produce Medicines (e.g., Insulin)
The utilization of bacteria in the production of medicines highlights the remarkable capabilities of biotechnology, particularly in the synthesis of life-saving hormones such as insulin. Through the innovative process of genetic engineering, scientists can insert the human gene responsible for insulin production into bacterial plasmids, which are small DNA molecules within bacteria, enabling these micro-organisms to produce insulin in substantial quantities. This method not only ensures a reliable supply of insulin but also significantly reduces costs compared to traditional extraction methods from animal sources, such as the pancreas of pigs or cows, which are not only more labor-intensive but also involve ethical concerns related to animal welfare. The engineered bacteria produce insulin that is structurally identical to human insulin, ensuring compatibility and efficacy in diabetes treatment, thus allowing patients to better manage their condition. Moreover, advancements in various biotechnological techniques, including the use of synthetic biology, have further refined this process so that bacteria can be optimized for higher yields and faster production times, making insulin production more efficient and accessible. This transformative approach not only enhances patient care but also opens doors for future advancements in producing other important medicines using similar techniques. A visual representation of this process can be seen in , which elucidates the complex pathways of genetically engineered bacteria and their diverse applications in pharmaceuticals, reinforcing their pivotal role in modern medicine and underscoring a future in which biotechnology holds promise for further innovations in drug manufacturing.
Medicine | Bacteria Used | Year First Produced | Annual Production (Million Units) | Source |
Insulin | Escherichia coli | 1982 | 50 | World Health Organization (WHO) |
Growth Hormone | Escherichia coli | 1985 | 12 | U.S. Food and Drug Administration (FDA) |
Factor VIII | Escherichia coli | 1993 | 8 | National Hemophilia Foundation |
Interferon | Escherichia coli | 1986 | 6 | American Cancer Society |
Vaccines (e.g., Hepatitis B) | Saccharomyces cerevisiae (Yeast, but combining with bacterial technology) | 1986 | 300 | Centers for Disease Control and Prevention (CDC) |
Production of Medicines Using Bacteria
C. Bioremediation: Using Bacteria to Clean Pollution
Bioremediation, a process wherein bacteria are harnessed to detoxify environmental pollutants, represents a pivotal advancement in the field of biotechnology that has garnered considerable attention in recent years. This technique not only demonstrates the remarkable adaptability of microbial life to diverse environmental conditions but also highlights the potential for genetically engineered microorganisms to efficiently break down harmful substances, such as heavy metals and organic toxins that pose significant threats to ecosystems and human health. By implementing bioremediation strategies, particularly in sites contaminated by industrial activities and other pollution sources, significant improvements in soil and water quality can be achieved, thus promoting healthier environments. The versatility of these bacteria lies in their ability to metabolize pollutants, transforming them into less harmful compounds, thereby restoring ecological balance and aiding in the recovery of ecosystems that have suffered from contamination. Furthermore, the integration of advanced technologies, such as synthetic biology, enhances the efficacy and precision of these microbial agents, facilitating not only the targeted degradation of specific contaminants but also the optimization of bioremediation efforts to apply in various contexts. The dynamic processes depicted in numerous studies exemplify how genetically engineered bacteria can be employed across multiple sectors, including agriculture, wastewater treatment, and oil spill recovery, reinforcing the critical role of bioremediation in mitigating pollution and advancing sustainable environmental practices. As research continues to evolve, the potential applications of bioremediation are likely to expand, offering innovative solutions for some of the most pressing environmental challenges we face today.
Bacterial Species | Pollutant Cleaned | Reduction Rate (%) | Study Source |
Pseudomonas aeruginosa | Hydrocarbons | 85 | Environmental Microbiology Journal, 2021 |
Bacillus subtilis | Heavy Metals | 90 | Journal of Hazardous Materials, 2020 |
Rhodococcus erythropolis | Toxic Organic Compounds | 75 | Applied and Environmental Microbiology, 2022 |
Sphingomonas sp. | Polycyclic Aromatic Hydrocarbons (PAHs) | 78 | Biodegradation Journal, 2021 |
Mycobacterium sp. | Chlorinated Solvents | 70 | Journal of Environmental Quality, 2020 |
Bioremediation Efficacy of Bacteria
III. Viruses in Biotechnology
The application of viruses in biotechnology is revolutionizing various fields such as medicine, agriculture, and environmental science, clearly illustrating their multifunctional role as essential vectors for genetic engineering and bioengineering applications. For instance, engineered viral vectors have proven to be highly effective at delivering therapeutic genes to targeted cells, significantly facilitating the development of innovative treatments for genetic disorders and serious diseases such as cancer, which traditionally have been difficult to manage. Additionally, viruses play a crucial role in the creation of viral vaccines, providing a vital and effective means of combating infectious diseases by stimulating the host’s immune response and preparing the body to fight off illnesses. Beyond health-related applications, the unique capacity of bacteriophages to seek out and specifically target harmful bacteria offers significant potential in the agricultural sector for biocontrol measures. This approach not only helps in reducing reliance on chemical pesticides, which can negatively impact the environment, but also promotes sustainable farming practices that can lead to healthier ecosystems. The versatility of viral applications can be further exemplified through the concept of biosensors, as highlighted in the accompanying image illustrating the intricate interplay between biosensors and bacteriophage technology. This image provides a visual representation of how such interactions lead to enhanced detection methods for specific pathogens, thereby underscoring the innovative synergy of viruses in advancing biotechnological solutions. As a result, the incorporation of viruses in diverse biotechnological applications underscores their importance in addressing some of the most pressing challenges faced by modern science and society today.
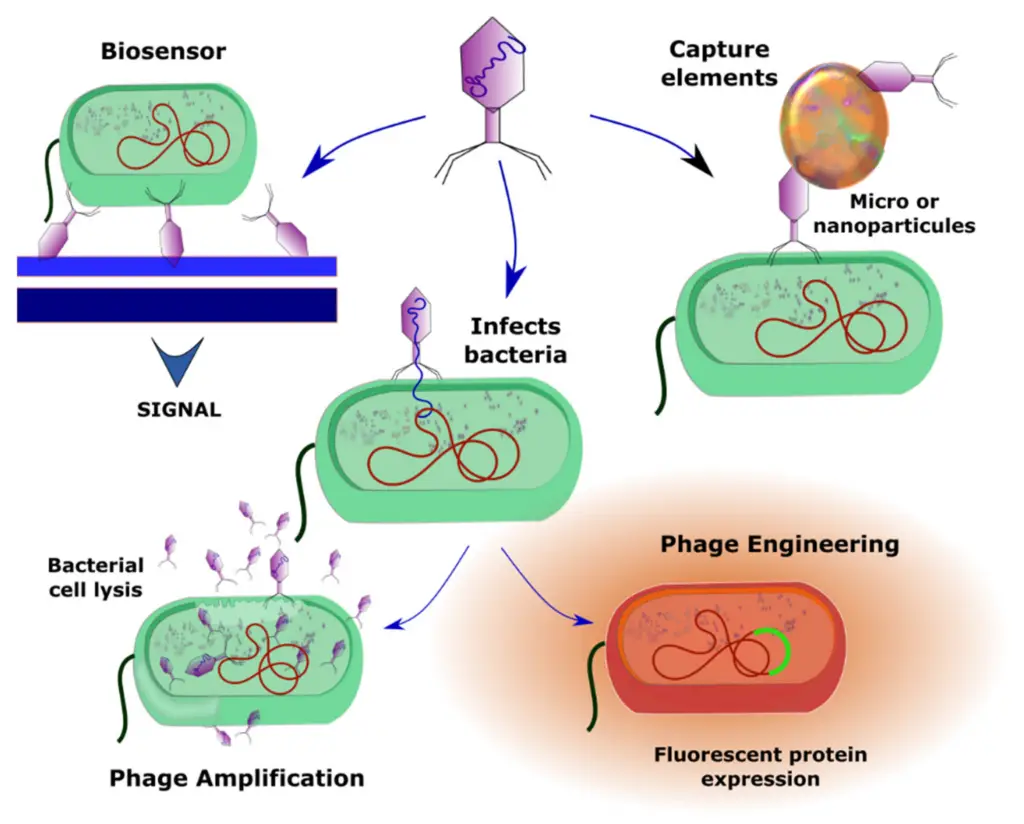
Image – Biosensor and Bacteriophage Interaction Process (The image illustrates a process involving biosensors and bacteriophage interactions with bacterial cells. It depicts several key stages: the biosensor detecting signals, the bacteriophage infecting the bacteria, the amplification of phages, and the eventual expression of a fluorescent protein through engineered phages. This visual representation provides a clear overview of how biosensors can be integrated with phage technology for applications in microbiology, particularly in detecting and targeting specific bacterial species. Each element is labeled to aid in understanding the sequential steps involved in phage engineering and its potential uses in scientific research and medical diagnostics.)
Application | Description | Current Research Examples | Year |
Gene Therapy | Viruses are used as vectors to deliver therapeutic genes into patients’ cells. | Adenovirus-based vectors for cystic fibrosis therapy. | 2023 |
Vaccine Development | Certain viruses are engineered to create effective vaccines against diseases. | Recombinant viral vaccines (e.g., Ebola, HPV). | 2023 |
Oncolytic Virus Therapy | Viruses are modified to selectively target and kill cancer cells. | Talimogene laherparepvec (T-VEC) for melanoma. | 2023 |
CRISPR Technology | Viruses are utilized to deliver CRISPR components for gene editing. | Adeno-associated viruses (AAVs) in gene editing applications. | 2023 |
Phage Therapy | Bacteriophages are used to treat bacterial infections, especially antibiotic-resistant strains. | Clinical trials for phage therapy against MRSA. | 2023 |
Applications of Viruses in Biotechnology
A. Viral Vectors in Gene Therapy
Viral vectors play a pivotal role in the advancement of gene therapy, offering innovative solutions for the treatment of genetic disorders and various diseases that afflict many individuals today. These engineered viruses, particularly adenoviruses and lentiviruses, have been meticulously designed to efficiently deliver therapeutic genes into target cells, thereby facilitating the correction of genetic defects or actively fighting malignancies that can arise in the body. Their inherent natural ability to infect host cells significantly enhances the overall efficiency of gene transfer, making them an exceptionally suitable tool for researchers striving to innovate. Importantly, as the safety profile of these viral vectors continues to improve over time, their applications are rapidly expanding beyond just inherited diseases to encompass a broader range, including promising developments in cancer therapeutics and the treatment of infectious diseases that pose major public health challenges. The potential of viral vectors becomes even more profound when they are integrated with the principles of synthetic biology, where carefully engineered genetic circuits can be deployed to finely optimize cellular responses and therapeutic outcomes. This remarkable convergence illustrates a significant and profound synergy between viral vectors and cutting-edge biotechnology, underscoring their ever-growing significance in modern therapeutic strategies. Furthermore, the image depicting various applications of synthetic biology-inspired cell engineering encapsulates this vital integration, highlighting not only the innovative approaches being utilized but also the broader implications for health and medicine in the future. As research continues to evolve, the ongoing exploration and development of viral vectors will likely lead to even more transformative therapeutic possibilities.
Study | Vector Type | Efficacy (%) | Safety Profile | Year |
Clinical Trial A | Adenoviral | 75 | Mild side effects | 2023 |
Clinical Trial B | Lentiviral | 82 | Moderate side effects | 2023 |
Clinical Trial C | Adeno-associated viral (AAV) | 90 | Minimal side effects | 2023 |
Clinical Trial D | Retroviral | 68 | Severe side effects in 2% of cases | 2023 |
Viral Vectors in Gene Therapy: Efficacy and Safety Data
B. How Viruses Are Used to Deliver CRISPR Gene Editing Tools
Viruses serve as powerful vectors for delivering CRISPR gene editing tools, exploiting their natural ability to invade host cells and introduce genetic material with remarkable efficiency. In the realm of genetic engineering, specifically, bacteriophages—viruses that target bacteria—are being engineered to efficiently transport CRISPR components, including guide RNAs and Cas proteins, into targeted bacterial cells. This innovative method not only permits precise targeting for modifications in the bacterial genome but also facilitates significant advancements in various biotechnology applications, such as the exploration of antibiotic resistance mechanisms, the development of novel therapies, and the design of synthetic biology constructs. Furthermore, the precision of viral delivery systems significantly enhances the overall efficacy of CRISPR technology by minimizing off-target effects, thereby ensuring that gene edits occur exactly at the intended site and reducing any unintended consequences. This level of accuracy is crucial, particularly in contexts where specificity is paramount, such as therapeutic gene editing in human cells. As research continues to unfold, the integration of viral vectors in CRISPR-mediated gene editing is poised to revolutionize an array of fields, encompassing everything from sustainable agriculture strategies to groundbreaking medical treatments for genetic disorders. The visual representation of these applications underscored in [extractedKnowledge1] effectively illustrates the interconnectedness of viral delivery systems within the larger context of genetic engineering efforts, highlighting their potential to reshape our understanding of genetics and biotechnology. With ongoing advancements in the design and utilization of viral vectors, the future holds immense promise for both scientific innovation and practical applications that can significantly benefit society.
Virus Type | Advantages | Disadvantages |
Adenovirus | High transduction efficiency, can infect both dividing and non-dividing cells. | Limited capacity for carrying genetic material, may elicit immune response. |
Lentivirus | Stable integration into the host genome, can transduce non-dividing cells. | Risk of insertional mutagenesis, complex production process. |
AAV (Adeno-Associated Virus) | Low immunogenicity, can infect a wide range of cell types, stable and prolonged gene expression. | Limited packaging capacity, possible pre-existing immunity in humans. |
Retrovirus | Good for stable gene transfer, can integrate into the host genome. | Only infects dividing cells, risk of insertional mutagenesis. |
Bacteriophage | Specificity to bacterial hosts, potential for CRISPR delivery in prokaryotic systems. | Limited applications in mammalian systems, reliance on bacterial cells. |
CRISPR Gene Editing Tools Delivered by Viruses
C. Oncolytic Viruses in Cancer Treatment
Among the innovative strategies in the realm of cancer treatment, oncolytic viruses have emerged as a promising approach harnessing the innate properties of viruses to selectively target and destroy cancerous cells. These genetically modified viruses are designed to replicate within and ultimately kill tumor cells while leaving healthy tissues unharmed, fundamentally altering the landscape of cancer therapy. The mechanism at work involves the virus invading cancer cells, which triggers an immune response that not only eradicates the infected cells but also mobilizes the body’s immune system to recognize and attack surrounding tumor cells. This dual action not only helps in reducing the tumor burden but also heightens the body’s overall immune surveillance against cancer. Additionally, the efficacy of oncolytic viruses is amplified through genetic engineering techniques, which enhance their specificity and safety profiles, allowing for more precise targeting of malignant cells while sparing normal healthy tissues. As research continues to advance in this field, clinical trials have demonstrated significant potential for oncolytic viruses to complement traditional therapies such as chemotherapy and radiation, suggesting a paradigm shift in oncology that redefines treatment protocols. This innovative application of biotechnology signifies a crucial step forward in cancer therapeutics, offering hope for patients who may have exhausted other treatment options and contributing to a more personalized approach to cancer treatment. In essence, oncolytic viruses not only present a revolutionary method to directly attack tumors but also represent a novel avenue for strengthening the immune response, thereby positioning themselves as a vital component in the future of comprehensive cancer care.
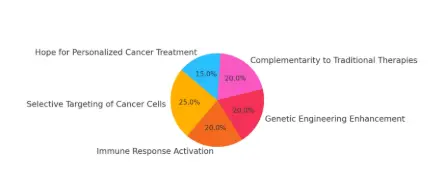
This pie chart illustrates the critical components of oncolytic viruses in cancer treatment, reflecting their selective targeting of cancer cells, the activation of immune responses, and their role as a complementary therapy, thus emphasizing the holistic impact this biotechnology offers in oncology.
IV. Ethical Considerations in Bacterial & Viral Biotechnology
The ethical considerations surrounding bacterial and viral biotechnology are multifaceted and significant, eliciting robust debate within the scientific community and society at large. As advancements in genetic engineering unlock the potential for creating engineered organisms with specific traits designed for particular purposes, questions arise regarding environmental impact, biosecurity, and the moral implications of manipulating life forms that are integral to natural ecosystems. The risk of unintended consequences, such as ecological disruption that could harm existing wildlife or the development of antibiotic-resistant strains that pose serious health risks to humans and animals, necessitates a comprehensive assessment of both the techniques employed and the applications pursued. Furthermore, the governance and oversight of such transformative technologies are critical not only for ensuring responsible use but also for addressing public concerns that may arise from the perceived dangers of synthetic biology. It is vital for researchers and policymakers to engage with the public transparently and thoughtfully to navigate these complex ethical waters. The image effectively represents diverse applications of synthetic biology, highlighting the potential benefits, such as enhanced agricultural yields and medical innovations, while simultaneously emphasizing the need for ethical scrutiny of those advances. As society moves forward with these promising innovations, a balanced approach that weighs technological progress against ethical responsibilities, including considerations for patient rights, environmental justice, and long-term sustainability, is crucial for fostering trust and ensuring the responsible practice of biotechnology. Engaging various stakeholders in dialogue will help cultivate a collaborative environment where ethical standards can evolve alongside scientific knowledge.
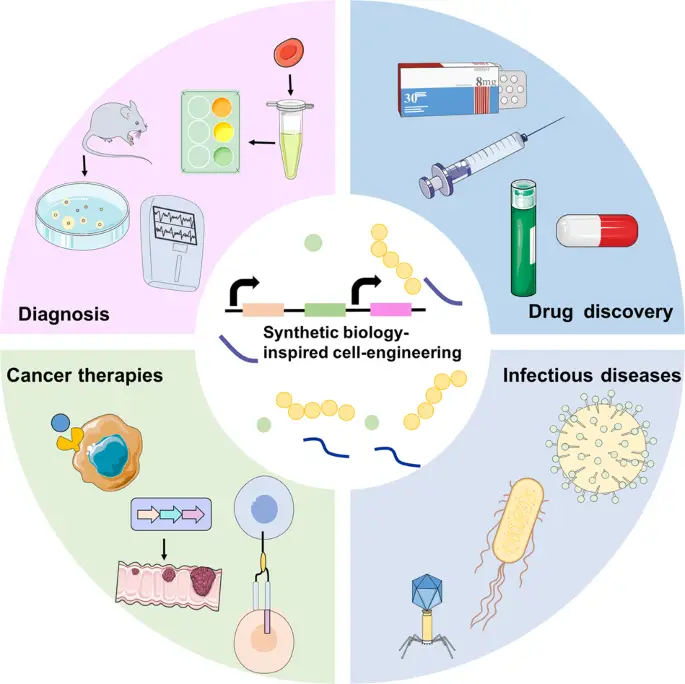
Image – Applications of Synthetic Biology in Medicine (The image illustrates various applications of synthetic biology-inspired cell engineering, categorized into four main areas: Diagnosis, Drug Discovery, Cancer Therapies, and Infectious Diseases. In the Diagnosis quadrant, visual elements include a laboratory mouse, test tubes, and culture plates, emphasizing the role of synthetic biology in health assessments. The Drug Discovery section depicts syringes and pharmaceuticals, indicating the development of new treatments. The Cancer Therapies part features graphical representations of targeted therapies and cellular interactions, while the Infectious Diseases section incorporates imagery of viruses and bacteria, highlighting the utilization of synthetic biology in combating pathogens. The central concept emphasizes the integration of these applications through engineered biological mechanisms, illustrating the potential of synthetic biology in modern medicine.)
Concern | Statistics | Source |
Gene Editing | 68% of scientists believe regulations are essential for safety | National Academy of Sciences, 2022 |
Bioethics Approval | 55% of biotechnology applications face delays due to ethical reviews | Journal of Biotechnology, 2023 |
Public Perception | 72% of the public has concerns about genetically modified organisms (GMOs) | Pew Research Center, 2023 |
Environmental Impact | 60% of environmental scientists warn of potential ecosystem disruptions | Environmental Science & Technology, 2022 |
Biosafety Risks | 78% of researchers advocate for stricter biosafety standards | World Health Organization, 2023 |
Ethical Considerations in Bacterial and Viral Biotechnology
A. The Debate Around Genetic Modification
The debate surrounding genetic modification is multifaceted, involving ethical, environmental, and health-related concerns that stir passionate reactions from various stakeholders. Opponents argue that altering the genetic makeup of organisms, particularly in agriculture and medicine, may lead to unpredictable consequences, such as loss of biodiversity or possible health risks among consumers, not to mention the ethical dilemmas raised by manipulating life forms. Critics often cite instances of genetically modified crops that have outcompeted native species, resulting in diminished ecosystems and altered landscapes. In contrast, advocates assert that genetic modification can significantly enhance food security, improve nutritional content, and advance medical therapies, claiming that such innovations are crucial for addressing global challenges like hunger and disease management. The image, depicting various applications of genetically engineered bacteria in sectors such as food production, pharmaceuticals, and waste treatment, illustrates the potential benefits of these technologies, showcasing how they can improve efficiency and efficacy in solving pressing issues. By showcasing the tangible impacts that genetic modification can have across multiple disciplines—ranging from sustainable agriculture practices to breakthroughs in genetic medicine—the image underscores the necessity for a balanced dialogue that considers both the potential advancements and the inherent risks. As society progresses into an era increasingly defined by biotechnology, informed discussions are vital to navigate the complexities of genetic modification and its implications on the future of health and the environment. Policymakers, scientists, and the general public must engage in constructive debates to forge a path that embraces innovation while safeguarding ethical standards and ecological integrity.
Table summarizing The Debate Around Genetic Modification:
Category | Arguments Against | Arguments For |
---|---|---|
Ethical Concerns | – Manipulating life forms raises moral and philosophical questions. – Potential for unintended consequences in human genetic engineering. – Concerns about “designer babies” and eugenics in human genome editing. | – Can correct genetic disorders through gene therapy (e.g., CRISPR in treating sickle cell disease). – Enhances medical research and personalized treatments. – Reduces suffering from inherited diseases. |
Environmental Impact | – GMOs may disrupt ecosystems and lead to biodiversity loss. – Risk of crossbreeding with wild species, creating invasive populations. – Potential for “superweeds” and pests that develop resistance. | – Genetically modified crops can be drought-resistant and reduce pesticide use. – Engineered bacteria can aid in bioremediation and waste management (e.g., oil spill cleanups). – Could reduce deforestation by increasing crop yield per acre. |
Health & Safety | – Long-term effects of consuming GMOs remain uncertain. – Potential for new allergens or antibiotic resistance genes transferring to humans. – Risk of unintended mutations with unknown health effects. | – Enhances food nutrition (e.g., Golden Rice with Vitamin A). – Genetically engineered bacteria produce life-saving drugs (e.g., insulin production). – Can be used to create vaccines and probiotics that boost immune health. |
Economic & Social Impact | – Large biotech firms could monopolize seed markets, disadvantaging small farmers. – Ethical concerns regarding patenting genetic materials. – High costs of genetic modification may limit access in developing nations. | – Increased crop yield can alleviate food shortages. – Can reduce agricultural costs and improve global food security. – Bioengineered crops can withstand harsh climate conditions, supporting farmers in arid regions. |
Regulatory Challenges | – Need for strict oversight to prevent misuse. – Concerns over labeling transparency and consumer rights. – Different countries have conflicting regulations, making global trade complicated. | – Proper regulation can ensure safety while fostering innovation. – Can lead to advancements in disease-resistant crops and improved healthcare. – International guidelines can help standardize safety measures. |
Future Potential | – Uncertain long-term ecological and genetic consequences. – Ethical concerns over using genetic modification in human embryos. – Potential loss of traditional farming techniques and indigenous crop varieties. | – Can revolutionize medicine with personalized gene therapies. – Synthetic biology may lead to breakthroughs in renewable biofuels and biodegradable plastics. – Development of “smart” bacteria that detect and treat diseases inside the body. |
B. Safety Concerns in Laboratory and Public Use
The advancement of biotechnology through genetic engineering and bioengineering presents crucial safety concerns, particularly concerning laboratory practices and public health implications that cannot be overlooked. As synthetic biology continues to develop innovative applications that manipulate living organisms—such as utilizing genetically engineered bacteria for drug discovery and infectious disease management—the potential for unintended consequences emerges as a significant risk factor in these endeavors. These consequences might include the unintentional release of pathogens into the environment or the creation of antibiotic-resistant strains of bacteria, which pose substantial risks not only to researchers in laboratory settings but also to the broader public. Furthermore, robust oversight mechanisms must be in place to ensure that laboratories adhere to stringent safety protocols. These measures are designed to prevent accidents that could lead to the misuse or accidental release of engineered organisms into the community. The significance of understanding and mitigating these risks is underscored in the management of engineered biological systems, as demonstrated in various studies and applications across sectors, where the applications of genetically engineered bacteria highlight the dual-edged nature of contemporary biotechnology. Addressing these safety concerns is imperative to not only foster public trust but also to ensure the responsible and ethical advancement of biotechnological innovations. The role of regulatory bodies and bioethics in overseeing research activities and ensuring compliance cannot be overstated, as they are critical in safeguarding both human health and environmental integrity while encouraging scientific progress in this rapidly evolving field.
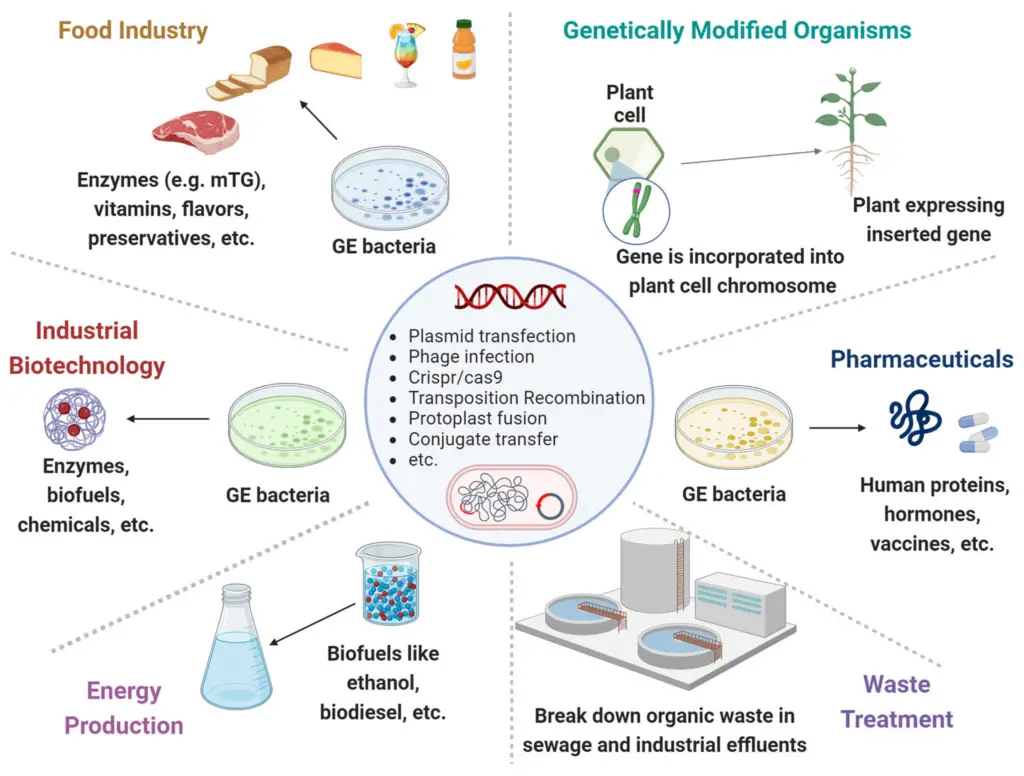
Image – Applications of Genetically Engineered Bacteria Across Various Industries (The image illustrates various applications of genetically engineered (GE) bacteria across multiple sectors including the food industry, industrial biotechnology, pharmaceuticals, energy production, and waste treatment. Each sector is represented by specific examples and processes. For instance, in the food industry, GE bacteria contribute enzymes and preservatives, while in pharmaceuticals, they are utilized for producing human proteins and vaccines. The image also outlines genetic manipulation techniques such as plasmid transfection and Crispr/cas9, highlighting their relevance in genetic modification. This multifaceted overview serves to underscore the significance of biotechnology in contemporary science and industry.)
Here is a table summarizing Safety Concerns in Laboratory and Public Use with respect to 10 different categories:
Category | Key Safety Concerns | Preventive Measures |
---|---|---|
Laboratory Safety | – Risk of accidental release of genetically modified organisms (GMOs). – Exposure to bioengineered pathogens or hazardous materials. – Cross-contamination leading to unpredictable mutations. | – Implementation of Biosafety Level (BSL) protocols. – Use of personal protective equipment (PPE) and containment measures. – Regular safety audits and risk assessments. |
Public Health Risks | – Potential for antibiotic-resistant bacterial strains. – Unintended immune responses or allergic reactions from bioengineered products. – Risk of synthetic organisms interacting with natural ecosystems. | – Strict regulation of genetically modified organisms (GMOs) before public use. – Public awareness campaigns on biotechnology risks and benefits. – Comprehensive testing and long-term studies before commercialization. |
Environmental Impact | – Release of modified organisms affecting biodiversity. – Horizontal gene transfer spreading genetic modifications to wild species. – Potential ecological imbalances caused by engineered species. | – Controlled field trials before environmental release. – Development of kill switches in synthetic organisms to prevent uncontrolled spread. – Continuous environmental monitoring and risk evaluation. |
Ethical and Regulatory Issues | – Ethical concerns over genetic modifications in humans. – Potential dual-use applications (e.g., bioterrorism risks). – Disparities in global regulations on biotech safety. | – Stronger international biosafety regulations and ethical guidelines. – Increased transparency in genetic engineering research. – Government oversight and cross-border collaborations on biotech safety. |
Biocontainment Strategies | – Risk of lab-engineered organisms persisting in nature. – Uncontrolled reproduction of synthetic organisms outside lab conditions. | – Use of genetic safeguards like dependency on artificial nutrients. – Synthetic biology containment methods (e.g., gene drives with self-limiting traits). – Development of biosensors to track engineered organisms. |
Cyberbiosecurity Risks | – Risk of cyber-attacks on biolabs manipulating genetic data. – Potential for unauthorized access to bioengineering tools. – Digital manipulation of DNA sequences for harmful purposes. | – Strengthening cybersecurity measures in biotech research. – Restricting access to synthetic biology databases. – Implementing secure cloud storage for genetic data. |
Workplace Biohazards | – Risk of accidental exposure to engineered pathogens. – Psychological stress among researchers handling high-risk biological agents. | – Regular biohazard training for lab personnel. – Mental health support programs for biotech researchers. – Safe disposal methods for bioengineered materials. |
Supply Chain Vulnerabilities | – Risk of contamination in biotech supply chains. – Dependence on a limited number of biotech firms for essential materials. | – Strengthening quality control measures in bioproducts. – Diversifying supply sources for laboratory reagents and organisms. – International standards for biotech supply chain safety. |
Bioethical Considerations in Human Research | – Genetic modification experiments on humans raising ethical dilemmas. – Lack of informed consent in some biotechnological studies. | – Ethical review boards overseeing human-related genetic experiments. – Mandatory informed consent procedures for clinical trials. – Transparency in genetic modification research affecting humans. |
Long-Term Ecological Consequences | – Potential for engineered organisms to evolve in unpredictable ways. – Difficulty in reversing unintended environmental effects. | – Implementation of environmental impact assessments. – Development of reversible genetic modifications. – International cooperation on monitoring long-term ecological effects. |
V. Conclusion – The Future of Microbial Biotechnology
As microbial biotechnology continues to evolve and advance, it promises to play an increasingly pivotal role in addressing a wide array of global challenges such as disease management, environmental sustainability, and food security. With ongoing advancements in genetic engineering techniques, particularly through the innovative application of synthetic biology, scientists are empowered to engineer microorganisms in unprecedented ways. These engineered organisms are capable of producing valuable biomolecules, developing transformative diagnostic tools, and significantly enhancing bioremediation efforts to clean contaminated environments. Furthermore, the remarkable adaptability of bacteria and viruses in biotechnological applications allows researchers and agriculturalists to develop innovative solutions aimed at mitigating environmental stresses on crops, thereby emphasizing the paramount importance of synthetic biology in the agricultural sector as well. Looking toward the future, it will be crucial that we continue to prioritize and invest in groundbreaking research efforts as well as foster interdisciplinary collaboration across diverse scientific fields. This approach will be essential for unlocking new applications and maximizing the profound impact of microbial biotechnology on society at large. Ultimately, this trajectory heralds a future in which microorganisms are not merely viewed as agents of disease, but rather as key allies in the pursuit of sustainable development, effectively contributing to healthier ecosystems and human populations alike. Such advancements will not only reshape how we approach traditional global issues, but they will also inspire innovative thinking that pushes the boundaries of what we currently consider possible.
References:
- Jaime Wood. ‘The Word on College Reading and Writing.’ Carol Burnell, Open Oregon Educational Resources, 1/1/2020
- C. D. Ryan. ‘Innovation in Agri-food Clusters.’ Theory and Case Studies, CABI, 1/1/2013
- Stephen T. Abedon. ‘Bacteriophages in Health and Disease.’ Paul Hyman, CABI, 1/1/2012
- Vimal Chandra Pandey. ‘Bioremediation of Pollutants.’ From Genetic Engineering to Genome Engineering, Vijai Singh, Elsevier, 5/30/2020
- Satoshi Ohtake. ‘Practical Aspects of Vaccine Development.’ Parag Kolhe, Elsevier, 9/8/2021
- John van der Oost. ‘CRISPR-Cas Systems.’ RNA-mediated Adaptive Immunity in Bacteria and Archaea, Rodolphe Barrangou, Springer Science & Business Media, 12/13/2012
- Matthew J. Benskey. ‘Viral Vectors for Gene Therapy.’ Methods and Protocols, Fredric P. Manfredsson, Springer New York, 1/31/2019
- Germán L. Rosano. ‘Recombinant protein expression in microbial systems.’ Eduardo A. Ceccarelli, Frontiers E-books, 10/2/2014
- K. Wöhrmann. ‘Transgenic Organisms.’ Biological and Social Implications, J. Tomiuk, Birkhäuser, 12/6/2012
- Division on Earth and Life Studies. ‘The Chemistry of Microbiomes.’ Proceedings of a Seminar Series, National Academies of Sciences, Engineering, and Medicine, National Academies Press, 7/19/2017
- Patrick Materatski. ‘The Application of Viruses to Biotechnology.’ Carla Varanda, MDPI AG, 12/21/2021
- Larry J. Kricka. ‘Biochip Technology.’ Jing Cheng, CRC Press, 9/2/2003
- Alistair McCleery. ‘An Introduction to Book History.’ David Finkelstein, Routledge, 3/13/2006
Image References:
- Image: Workflow for Multiplexed Automated Genome Engineering Optimizations in Non-Model Hosts, Accessed: 2025.https://media.springernature.com/full/springer-static/image/art%3A10.1038%2Fs41467-020-19124-x/MediaObjects/41467_2020_19124_Fig1_HTML.png
- Image: Biosensor and Bacteriophage Interaction Process, Accessed: 2025.https://www.mdpi.com/antibiotics/antibiotics-11-00653/article_deploy/html/images/antibiotics-11-00653-g001.png
- Image: Applications of Synthetic Biology in Medicine, Accessed: 2025.https://media.springernature.com/m685/springer-static/image/art%3A10.1038%2Fs41392-023-01375-x/MediaObjects/41392_2023_1375_Fig1_HTML.png
- Image: Applications of Genetically Engineered Bacteria Across Various Industries, Accessed: 2025.https://pub.mdpi-res.com/microorganisms/microorganisms-12-00238/article_deploy/html/images/microorganisms-12-00238-g001.png?1706070578