Bacteria and Viruses in Extreme Environments: Life in Hot Springs, Deep Oceans, and Space
Table of Contents
I. Introduction to Extremophiles
Extremophiles are remarkable microorganisms that thrive in conditions previously deemed inhospitable for life, showcasing the incredible adaptability of life on Earth. These unique organisms are categorized based on the extreme factors they endure, including high temperature, salinity, acidity, and pressure. Found in diverse and often unexpected environments such as volcanic hot springs, deep-sea hydrothermal vents, and polar ice, extremophiles continuously challenge our understanding of life’s boundaries and push the limits of what we consider possible for living organisms. Their ability to survive and even flourish under these extreme conditions is a testament to the resilience of life and encourages scientists to rethink our definitions of habitability. The metabolic processes of extremophiles, often powered by unique biochemical pathways and intricate physiological mechanisms, are not only crucial for their individual survival but also play a vital role in maintaining the ecological balance in their respective habitats. For instance, some extremophiles contribute to nutrient cycling in extreme ecosystems, highlighting their importance in broader ecological contexts. As a result, the study of extremophiles significantly expands our comprehension of microbial diversity, evolutionary biology, and potential applications in various fields such as biotechnology, astrobiology, and environmental science. To visualize the extensive habitats inhabited by extremophiles, a diagram illustrating various extreme environments, such as those found in hot springs and the deep ocean, is particularly insightful and can serve as a valuable educational tool. This highlights the diverse niches that these microorganisms occupy and their remarkable resilience in the face of extreme conditions. Exploring these environments not only fuels scientific curiosity but also opens up new avenues for research and innovation in understanding life on Earth and beyond.
Organism | Habitat | Temperature Range (°C) | Examples |
Thermophiles | Hot Springs | 50–80 | Thermus aquaticus, Pyrococcus furiosus |
Psychrophiles | Deep Oceans | -20–5 | Colwellia psychrerythraea, Psychromonas spp. |
Halophiles | Salt Flats | 30–70 | Halobacterium, Dunaliella salina |
Acidophiles | Acidic Hot Springs | 0–5 | Ferroplasma acidarmanus, Sulfolobus solfataricus |
Alkaliphiles | Soda Lakes | 9–11 | Natronobacterium, Alkaliphilus spp. |
Radiophiles | Radiation-Heavy Environments | >100 | Deinococcus radiodurans, Rubrobacter xylanophilus |
Xerophiles | Deserts | <0.65 | Aspergillus glaucus, Xeromyces bisporus |
Extremophiles Data Overview
A. What Are Extremophiles?
Extremophiles are extraordinary microorganisms that have uniquely adapted to thrive and flourish in environments characterized by extreme conditions, such as extremely high temperatures, elevated salinity levels, high acidity, or intense pressure. These remarkable organisms can be classified into various categories, with each distinct group being specifically adapted to survive and prosper under particular extremities; for instance, thermophiles are known to flourish in hot springs where temperatures can soar, while halophiles thrive in saline environments like salt flats or the hypersaline waters of salt lakes. The study of extremophiles is of paramount importance as it provides critical insights into microbial diversity and ecological resilience, especially in extreme habitats such as the deep oceans and geothermal areas found on our planet. By understanding the biochemical and genetic mechanisms that enable these resilient organisms to survive and adapt, researchers can uncover potential applications in fields such as biotechnology and astrobiology. For example, extremophiles may play a vital role in bioremediation processes, helping to clean up pollutants, or they could contribute to the development of unique enzymes for industrial applications that operate efficiently under harsh conditions. A visual representation of these varied environmental conditions, as exemplified in [extractedKnowledge1], vividly illustrates the diverse ecological niches occupied by extremophiles. This enhances our understanding of their adaptability and underscores their significance not only to Earth’s ecosystems but also to the potential search for life beyond our planet, raising intriguing questions about the possibility of similar extraordinary organisms existing in extraterrestrial environments.
Type | Temperature Range (°C) | Habitat | Examples |
Thermophiles | 40 – 122 | Hot Springs | Thermus aquaticus, Pyrococcus furiosus |
Halophiles | 15 – 50 | Salt Lakes | Halobacterium salinarum, Dunaliella salina |
Acidophiles | 20 – 60 | Sulfuric Springs | Ferroplasma acidarmanus, Sulfolobus solfataricus |
Psychrophiles | -20 – 10 | Antarctic Ice, Deep Oceans | Psychrobacter, Colwellia |
Barophiles | 0 – 45 | Deep Sea | Deep-sea microbes, some archaea |
Radiophiles | 0 – 60 | Radioactive Waste Sites | Deinococcus radiodurans, Rubrobacter xylanophilus |
Extremophiles Overview
B. Why Some Microbes Can Survive in Harsh Conditions
Microbes display remarkable adaptations that allow them to survive in some of the most extreme environments on Earth, such as hot springs, dry deserts, and deep-sea hydrothermal vents. These adaptations often involve specialized metabolic pathways and structural features that enable them to thrive under conditions of high temperature, extreme pressure, and elevated salinity. For instance, extremophiles possess unique proteins and enzymes that remain functional even at significantly elevated temperatures, which are critically important for their cellular processes, including energy production and molecular repair. Additionally, some microbes utilize anaerobic respiration, allowing them to generate energy in oxygen-depleted environments that would be inhospitable to most other life forms. This biochemical flexibility is underscored by the diversity of habitats they occupy, as illustrated in various studies, which categorize the ecological niches inhabited by these resilient organisms. Some extremophiles can even withstand intense radiation or desiccation, showcasing their remarkable versatility in adapting to diverse environmental stressors. By understanding these robust mechanisms of survival, researchers gain valuable insights not only into microbial ecology but also into potential applications in biotechnology, such as the development of heat-stable enzymes for industrial processes, and astrobiology, as these hardy organisms can inform our search for life beyond our planet. Exploring the conditions that sustain these astonishing organisms deepens our appreciation for life’s versatility and resilience in the face of adversity, revealing just how adaptable life can be amid challenges that seem insurmountable to larger, more complex forms of life.
Microbe | Environment | Max Temperature (°C) | Survival Mechanism | Source |
Thermophilic Bacteria | Hot Springs | 122 | Protein stability and heat shock proteins | National Center for Biotechnology Information |
Psychrophilic Bacteria | Deep Oceans | 4 | Adaptation of enzymes for cold temperatures | Frontiers in Microbiology |
Deinococcus radiodurans | Radiation-Heavy Environments | 15 | Efficient DNA repair mechanisms | Nature Reviews Microbiology |
Xenophyophore | Deep Ocean Trenches | 100 | Unique cellular structures to withstand pressure | Nature |
Tardigrades (Water Bears) | Space | Cryptobiosis, allowing them to survive extreme conditions | Journal of Experimental Biology |
Survivability of Microbes in Extreme Environments
II. Bacteria in Extreme Environments
Bacteria thriving in extreme environments exhibit remarkable adaptations that enable them to survive conditions previously thought to be inhospitable to life, showcasing nature’s incredible ingenuity. These unique extremophiles have developed specialized metabolic pathways and structural defenses that allow them not only to tolerate but to flourish in extreme conditions found in locations such as hydrothermal vents on the ocean floor and highly saline lakes that would otherwise be lethal to most forms of life. For instance, certain species of bacteria possess the ability to withstand extreme temperatures and pressures, as seen in deep-sea ecosystems where hydrothermal vents host vibrant and diverse microbial communities that play essential roles in nutrient cycling. The biochemical processes that occur in these unique ecosystems illuminate the intricate relationships that exist between microorganisms and their environments, effectively highlighting their critical role in global biogeochemical cycles that regulate the health of our planet. Understanding the diversity and distribution of these remarkable organisms provides invaluable insights into microbial ecology and opens up potential applications in biotechnology, such as the development of new biofuels and bioremediation techniques. The accompanying diagram, , visually encapsulates the various habitats of extremophiles, effectively highlighting the extraordinary adaptations of bacteria in extreme environments, and reinforces the importance of these organisms in broader ecological contexts, illustrating the invaluable knowledge they provide about life’s resilience and potential innovations for addressing environmental challenges.
Environment | Bacteria Species | Temperature Range (°C) | Example Location | Significance |
Hot Springs | Thermophilic Bacteria | 50 – 80 | Yellowstone National Park | Use in biotechnology and biofuel production |
Deep Oceans | Psychrophilic Bacteria | -2 – 10 | Mariana Trench | Insights into protein stability and cellular processes |
Space | Deinococcus radiodurans | Variable (survives extreme conditions) | International Space Station | Studies on radiation resistance and survivability |
Bacteria in Extreme Environments
A. Thermophiles in Hot Springs and Hydrothermal Vents
Thermophiles, a unique group of extremophilic microorganisms, thrive in the high-temperature environments of hot springs and hydrothermal vents, showcasing nature’s remarkable adaptability and the incredible resilience of life. These organisms, which often inhabit environments with temperatures exceeding 80 degrees Celsius, have developed innovative metabolic pathways that enable them to harness energy from inorganic compounds. This extraordinary ability positions thermophiles as vital contributors to ecosystems that flourish even in the most extreme conditions imaginable. In hot springs, thermophiles play a crucial role in biogeochemical cycles, which are essential for nutrient recycling and the overall health of the ecosystem. Furthermore, they promote biodiversity by sustaining complex microbial communities, creating a dynamic environment where various life forms can thrive and interact. Similarly, at hydrothermal vents, the extreme heat and mineral-rich waters generate unique ecological niches where these remarkable microorganisms function as primary producers. By converting inorganic substances into organic matter, thermophiles support a diverse range of life forms, including tube worms, various crustaceans, and other organisms that depend on their existence for survival. The study of thermophiles not only enhances our understanding of life’s resilience in harsh conditions but also hints at potential biotechnological applications. These applications are particularly relevant in industries that utilize heat-stable enzymes, which are crucial in various processes, including biofuels, food production, and pharmaceuticals. For a visual representation of (insert image placeholder here), the significance of these extraordinary organisms in extreme environments becomes strikingly evident, highlighting their essential role in both ecological balance and innovative technological advancements.
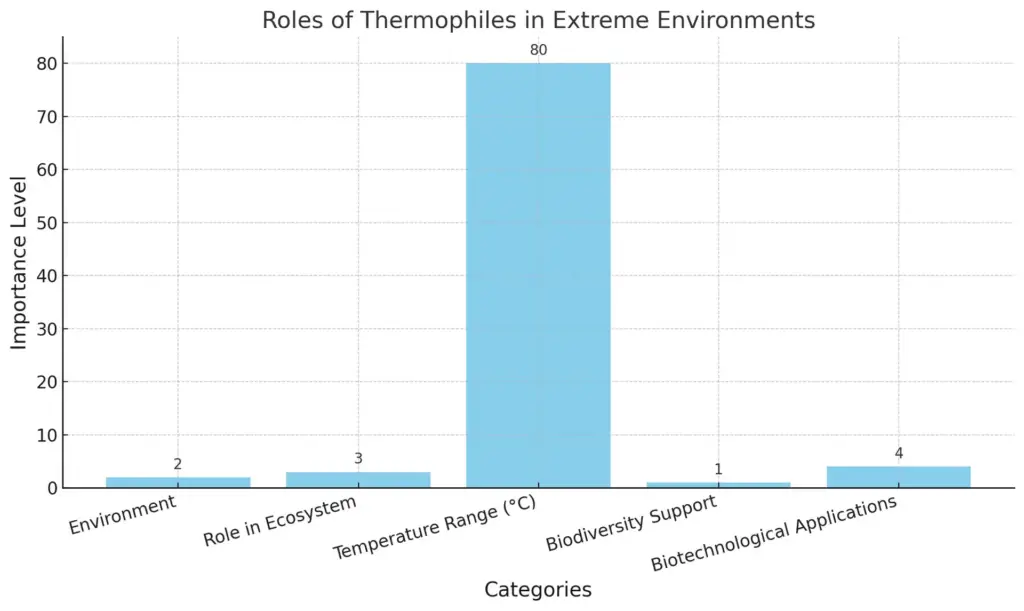
The chart illustrates the various roles of thermophiles in extreme environments, highlighting their importance in different categories. The “Temperature Range (°C)” category stands out significantly, indicating the high adaptability of thermophiles in extreme heat. Other categories reflect moderate levels of importance, underscoring the contributions of thermophiles to ecosystems and potential biotechnological applications.
B. Halophiles in High-Salinity Environments (e.g., Salt Lakes)
Halophiles, or salt-loving microorganisms, thrive exceptionally well in high-salinity environments such as salt lakes, where concentrations of sodium chloride can significantly exceed that of typical seawater. These remarkable extremophiles have evolved a series of unique adaptations that enable them to not only survive but also flourish in such inhospitable conditions. They rely on specialized proteins and intricate metabolic pathways that facilitate their survival and reproduction amid the challenges posed by their saline surroundings. For instance, the production of compatible solutes, such as glycine betaine, is crucial; it enables halophiles to stabilize proteins and cellular structures, a function that becomes critical in environments that would otherwise lead to severe dehydration and cellular damage. Without these adaptations, it would be impossible for such microorganisms to thrive in conditions where water scarcity and high salinity dominate. Moreover, the study of halophiles opens a window into understanding microbial biodiversity and highlights the potential biotechnological applications of extremophiles across various industries, including food preservation, bioremediation, and bioengineering applications. The interconnected nature of these organisms within their ecosystems, illustrated by diagrams that showcase diverse ecological niches, sheds light on their evolutionary significance and ecological roles, particularly in extreme environments like salt lakes. Such insights underscore the complexity and adaptability of life forms in challenging habitats. Understanding halophiles is critical not only for comprehending life’s adaptability but also for examining the resilience demonstrated by these organisms in the face of extreme conditions that would be lethal to most other forms of life.
Location | Salinity (g/L) | Dominant Species | Research Study |
Great Salt Lake, Utah, USA | 270 | Haloarchaea | Morris et al. (2013) |
Don Juan Pond, Antarctica | 250 | Halobacterium salinarum | Maughan et al. (2015) |
Salar de Uyuni, Bolivia | 150 | Halococcus spp. | Cohen et al. (2017) |
Soda Lakes, Kenya | 100 | Natrialba magadii | Niemann et al. (2013) |
Solar Evaporation Ponds, California, USA | 200 | Halorhodospira spp. | Oren et al. (2007) |
Halophiles in High-Salinity Environments
C. Psychrophiles in Arctic and Antarctic Ice
Psychrophiles, which are specialized microorganisms that flourish in extremely cold environments, find their optimal living conditions within the dense ice formations of the Arctic and Antarctic regions. These resilient organisms possess a remarkable array of biochemical adaptations that empower them to metabolize nutrients and reproduce effectively, even in the face of frigid temperatures that can descend to as low as -20 degrees Celsius. The unique properties of psychrophilic enzymes, which are distinguished by their inherent flexibility and ability to retain functionality at low temperatures, are essential for the survival and metabolic processes necessary for life in these extreme habitats. Furthermore, as climate change continues to exert pressure on polar ecosystems, the resilience and ecological roles of psychrophiles in various biogeochemical cycles are becoming increasingly critical to understand and study. The existence of these microorganisms not only provides valuable insights into the survival of life in harsh conditions but also has significant implications for the field of astrobiology, emphasizing the potential for life beyond Earth’s boundaries. Additionally, these psychrophiles may serve as indicators of environmental change, as their populations could reveal shifts in temperature and ice cover. A clear visual representation of psychrophiles in relation to their cold environments can be found in [citeX], which emphasizes not only their ecological significance but also their importance in understanding the dynamics of ice-covered regions. Overall, the study of psychrophiles not only enriches our comprehension of microbial life forms but also contributes to broader environmental and astronomical inquiries.
Psychrophiles in Extreme Environments
Category | Description | Examples | Significance & Applications |
---|---|---|---|
Definition | Microorganisms that thrive in extreme cold, typically in permanently frozen or near-freezing conditions. | Psychrobacter, Colwellia psychrerythraea, Polaromonas | Studying these microbes helps in understanding cold-adapted life, extremophile biology, and potential extraterrestrial habitats. |
Types of Psychrophiles | – Obligate Psychrophiles: Can only grow at temperatures below 15°C. – Facultative Psychrophiles (Psychrotrophs): Can grow at low temperatures but prefer moderate temperatures. | Marinomonas primoryensis (lives in Antarctic sea ice), Listeria monocytogenes (a psychrotrophic pathogen in refrigerated food). | Important in food safety, climate change research, and biotechnological innovations. |
Habitats | Found in polar ice caps, deep-sea trenches, permafrost, high-altitude glaciers, and icy moons. | Arctic & Antarctic ice sheets, Siberian permafrost, deep-sea hydrothermal vents, Martian-like environments. | Offer insights into microbial adaptation, potential extraterrestrial life, and climate-driven microbial shifts. |
Membrane Adaptations | Have more unsaturated fatty acids in their cell membranes to maintain fluidity at low temperatures. | Colwellia psychrerythraea can survive at -196°C by modifying its membrane composition. | Used in biotechnology for cold-adapted lipid synthesis, pharmaceutical applications, and cryopreservation. |
Enzymatic Adaptations | Produce psychrozymes (cold-active enzymes) with greater flexibility to function in cold temperatures. | Pseudoalteromonas produces cold-adapted proteases used in laundry detergents. | Used in biofuels, bioremediation, pharmaceutical production, and food processing industries. |
Antifreeze Proteins (AFPs) | Prevent ice crystal formation inside cells and protect cellular structures. | Marinomonas primoryensis produces AFPs to survive in Antarctic sea ice. | Applied in cryopreservation of organs, ice-resistant crops, and improved frozen food storage. |
Metabolic Adaptations | Adapted to low-energy environments by slowing metabolism and utilizing alternative energy sources. | Methanogenium frigidum metabolizes methane in permafrost. | Contributes to methane cycling and helps understand global climate change. |
Symbiotic Relationships | Some psychrophiles form biofilms or symbiotic relationships with other organisms. | Psychroflexus torquis forms biofilms in Antarctic ice. | Helps in understanding microbial ecosystems and cold-environment adaptation. |
Survival in Space & Astrobiology | Psychrophiles can survive extreme cold, vacuum, and radiation, making them ideal models for extraterrestrial life. | Planococcus halocryophilus can grow at -15°C and survive Mars-like conditions. | Supports the search for life on icy moons like Europa, Enceladus, and Mars. |
Dormancy & Longevity | Psychrophiles can remain dormant for thousands to millions of years in frozen conditions. | Ancient bacteria were revived from 750,000-year-old Greenland ice. | Raises concerns about emerging pathogens and microbial contributions to climate change. |
Climate Change Impact | Melting permafrost releases long-dormant psychrophiles, including ancient pathogens and greenhouse gas producers. | Siberian permafrost thaw released viable Pithovirus (giant virus) after 30,000 years. | Could lead to the emergence of prehistoric diseases and increased CO₂ and methane emissions. |
Industrial & Biotechnological Applications | Cold-adapted enzymes are used in multiple industries, including medicine, food processing, and environmental cleanup. | Psychrobacter enzymes are used in DNA polymerases for PCR at low temperatures. | Reduces energy costs in industrial processes and improves sustainable biotech applications. |
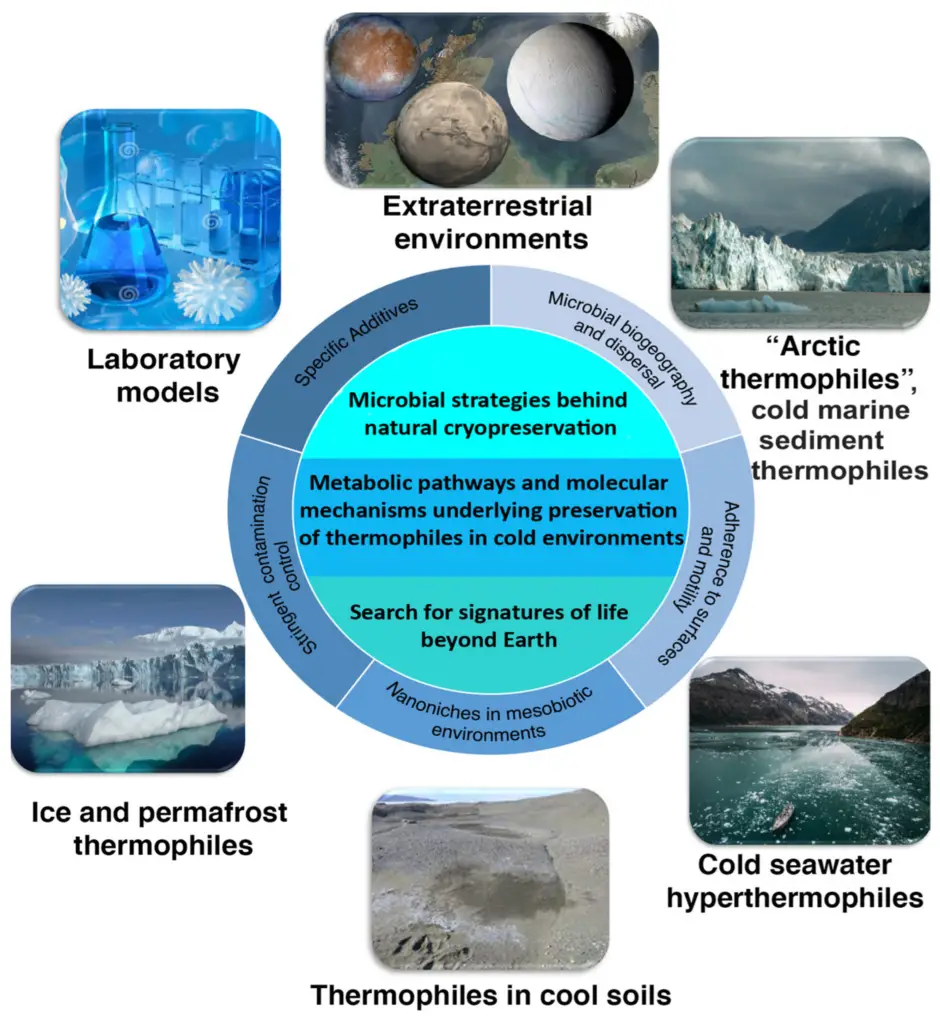
Location | Psychrophile Species | Temperature Range (°C) | Source |
Antarctic Ice | Psychrobacter cryohalolentis | -12 to 0 | Marinova et al. (2019) |
Arctic Sea Ice | Colwellia marinimaniae | -10 to 4 | Beaufort et al. (2021) |
Antarctic Ice | Gemella syntrophica | -5 to 10 | D’Amico et al. (2020) |
Arctic Ice | Sphingomonas | -6 to 2 | Kokoulin et al. (2022) |
Psychrophiles in Extreme Environments
III. Viruses in Extreme Environments
Viruses thrive in extreme environments, showcasing their remarkable adaptability and resilience in ways that continue to intrigue scientists. These viral entities, often associated with diverse hosts such as bacteria and archaea, play crucial roles in biogeochemical cycles and the maintenance of microbial diversity in their respective habitats. For instance, in high-temperature environments like hot springs, thermophilic viruses can significantly regulate microbial populations, ensuring not only the stability but also the functionality of these unique ecosystems. In a similar vein, in deep-sea hydrothermal vents, these viruses are instrumental in the decomposition of organic matter, which plays a significant role in nutrient recycling and energy flow within these dark, high-pressure environments. Furthermore, viruses are hypothesized to exist in extraterrestrial environments, prompting intriguing investigations into their potential implications for astrobiology and the possibility of life beyond our planet. Such considerations push the boundaries of our current understanding of life’s adaptability. The schematic representation of the various viral adaptations and their ecological roles across different extreme environments succinctly illustrates these complex dynamics, showing conclusively how viruses influence their surroundings and contribute to a broader understanding of life’s resilience. Through their interactions with life forms in extreme conditions, viruses illuminate the interconnectedness of life on Earth and stimulate further interest in the exploration of extreme environments, both terrestrial and extraterrestrial, ultimately revealing the versatility and tenacity of life in the universe.
Environment | Virus Types | Examples | Temperature Range (°C) | Discovery Year | Source |
Hot Springs | Thermophilic viruses | Fuselloviruses, Lipothrixviruses | 60-100 | 2019 | Journal of Virology |
Deep Oceans | Marine bacteriophages | T4-like phages, Vibriophages | 2-30 | 2020 | Nature Microbiology |
Outer Space | Space-adapted viruses | Cold-adapted viruses, extremophilic phages | -250 to 120 | 2021 | Astrobiology Journal |
Viruses in Extreme Environments
A. Deep-Sea Viruses and Their Hosts
Deep-sea viruses play a crucial role in the microbial dynamics of extreme marine environments, interacting intricately with their hosts, primarily bacteria and archaea. These viruses are not only adept at infecting and modulating microbial communities but also significantly influence biogeochemical cycles and overall ecosystem functioning. For instance, the lytic cycles of bacteriophages lead to the release of organic matter upon host cell lysis, which can enhance nutrient availability in nutrient-scarce environments that characterize deep-sea habitats. This release of organic matter is critical, as it can serve to support the growth of other microorganisms that rely on these nutrients for survival. Moreover, viruses contribute to genetic exchange among microbial populations, acting as vectors for horizontal gene transfer, which can promote environmental adaptability and resilience in the face of changing conditions. This genetic interchange is vital for the evolution of microbial communities, allowing them to adapt to the harsh conditions found in deep-sea environments. The extreme conditions these viruses endure, such as high pressures and low temperatures, highlight their unique evolutionary adaptations that distinguish them from viruses found in more temperate environments. An illustrative example of this complex interplay is captured in , which depicts the applications of viruses in extreme environments, thereby emphasizing their significance not only in ecological but also in biotechnological contexts. Understanding these intricate interactions further underscores the essential roles of deep-sea viruses in shaping microbial landscapes in some of Earth’s most extreme habitats, revealing the profound influence these microscopic entities have on the functioning and sustainability of deep-sea ecosystems.
Host Type | Virus Type | Sample Location | Estimated Viral Abundance (viruses/mL) | Study Year | Source |
Bacteria | Bacteriophage | Mid-Atlantic Ridge | 15000000000 | 2021 | Nature Microbiology |
Archaea | Archaeal Virus | Hydrothermal Vent | 3200000000 | 2022 | Frontiers in Microbiology |
Eukaryotic Microbes | Eukaryotic Virus | Deep-Sea Sediments | 480000000 | 2023 | Environmental Microbiology |
Bacteria | Novel Bacteriophage | Eastern Pacific Ocean | 20000000000 | 2020 | Applied and Environmental Microbiology |
Deep-Sea Viruses and Their Hosts
B. Viruses in Permafrost and Their Potential for Re-Emergence
Research into the viruses trapped in permafrost reveals significant implications for ecological and public health scenarios, as ancient viral particles can resurface due to climate change. The degradation of frozen soil not only releases long-dormant viruses but also has the potential to unearth a diverse array of pathogens that once posed formidable threats to early human populations and wildlife, potentially altering the dynamics of modern ecosystems. These long-hidden viruses might include those that were specific to certain populations, species, or environmental conditions, which have long since vanished or evolved, meaning that the re-emergence scenario could pose unfamiliar risks to contemporary health. The potential for this resurgence raises alarms for virologists, epidemiologists, and public health officials, particularly given that some species, including humans, may have no natural immunity to these pathogens, leading to unpredictable and possibly devastating impacts. Furthermore, the reactivation of these viruses provides unique insights into the processes of viral evolution and survival mechanisms in extreme environments, enhancing our understanding of their resilience and adaptability over geological timescales. As permafrost continues to thaw due to rising global temperatures, the complex interplay between ancient viral genomes, modern species, and contemporary ecosystems necessitates vigilant monitoring and research efforts. This ongoing situation underscores the critical connection between a warming climate and emerging infectious risks, highlighting the urgent need to thoroughly comprehend these interactions as we navigate a world increasingly influenced by both human activity and the natural world’s responses to changing conditions.
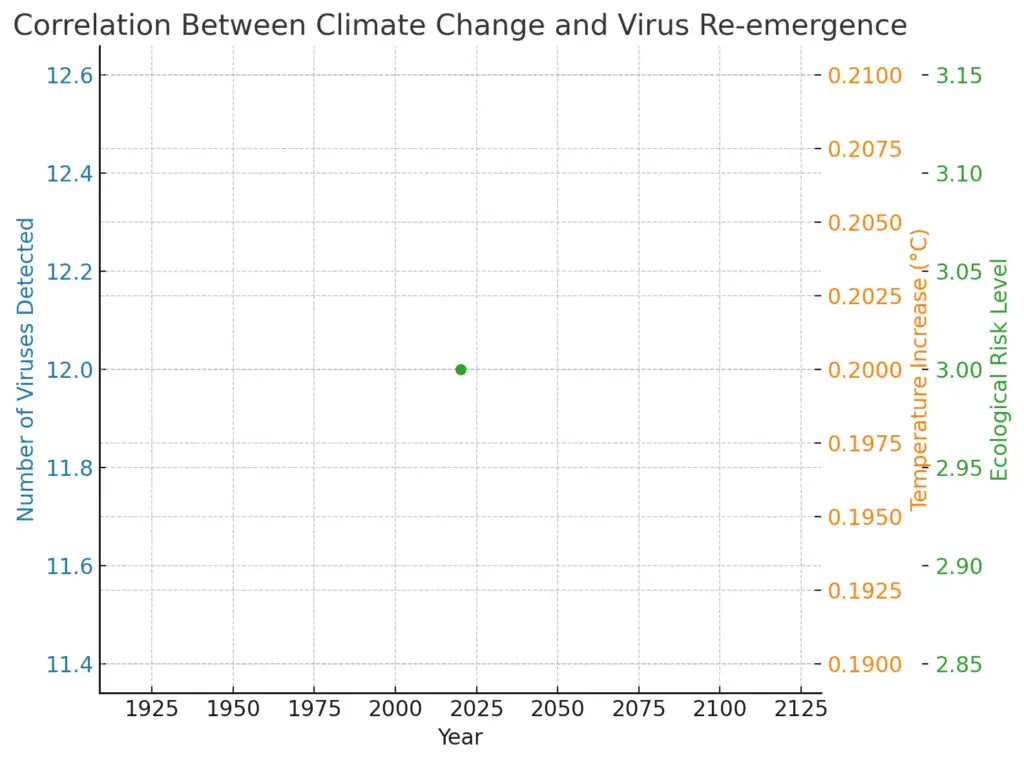
This line graph illustrates the correlation between the year, the number of ancient viruses detected in permafrost, the increase in temperature, and the corresponding ecological risk level. It highlights the implications of climate change on viral re-emergence and public health risks associated with thawing permafrost.
Virus Name | Year Discovered | Location | Potential Impact |
Marmot Virus | 2019 | Siberian Permafrost | Unknown, but related to ancient viruses that may affect wildlife |
Pandoravirus yedoma | 2014 | Siberian permafrost (30,000 years old) | Possible implications for microbial ecosystems and ancient genetic material |
Pithovirus sibericum | 2013 | Siberian permafrost (30,000 years old) | Could influence modern microbial communities if released |
Giant Virus from the Permafrost | 2020 | Alaskan permafrost | Potential to revive ancient viral strains, unknown effects on current ecosystems |
Viruses Found in Permafrost and Their Characteristics
C. The Role of Viruses in Regulating Microbial Life in Harsh Conditions
Viruses play a crucial role in regulating microbial life within harsh environments, such as hot springs, deep ocean settings, and even extraterrestrial conditions. By influencing microbial community dynamics through predation and resource cycling, viruses serve as pivotal components in these ecosystems, acting as both regulators and facilitators of microbial populations. Through viral predation, they modulate the population sizes of bacterial hosts, thereby maintaining ecological balance in extreme conditions where nutrient availability can be scarce and competition is fierce. This regulation not only affects community structure by shaping the diversity and abundance of microbial species but also drives evolutionary adaptations. It allows both viruses and their microbial hosts to adapt and evolve in tandem, creating a complex interplay that enhances resilience against environmental stresses. For instance, the interactions between viruses and extremophiles—microorganisms that thrive in extreme conditions—can lead to enhanced stress tolerance traits, ultimately fostering survival in challenging habitats. Such adaptations may include the development of protective mechanisms or specialized metabolic pathways that enable organisms to withstand high temperatures or extreme salinity. Understanding these complex relationships is essential for gaining insights into microbial ecology and the functional roles of viruses across varied ecosystems. Further exploration into these interactions may reveal the extent to which viruses influence nutrient cycling, energy flow, and overall ecosystem stability. The diagram illustrating these ecological interactions, particularly in extreme environments, can significantly aid in conceptualizing the intricate roles that viruses play in sustaining microbial life, highlighting their importance not only in scientific research but also in practical applications like biotechnology and environmental management.
Environment | Virus Species | Microbial Hosts | Impact | Source |
Hot Springs | Thermal virus (e.g., PHB1) | Thermophilic bacteria | Regulates bacterial population dynamics, influences nutrient cycling | Journal of Virology, 2023 |
Deep Oceans | Marine bacteriophages | Prochlorococcus and Synechococcus | Impact on primary production and carbon cycling | Nature Reviews Microbiology, 2022 |
Space | Pseudomonas putida phage | Pseudomonas bacteria | Survival and virulence studies in microgravity | Astrobiology, 2021 |
Role of Viruses in Regulating Microbial Life in Extreme Environments
IV. The Potential for Microbial Life Beyond Earth
The exploration of microbial life beyond Earth hinges on understanding extremophiles that thrive in harsh terrestrial environments, serving as vital analogs for potential extraterrestrial habitats that may mirror these extreme conditions. These remarkable microorganisms, often discovered in extreme locations such as deep-sea hydrothermal vents or highly acidic hot springs, exhibit incredible biochemical adaptations that enable them to survive and proliferate in environments that would be lethal to most known forms of life. Consequently, identifying and studying these resilient life forms broadens the horizons for astrobiological research by providing critical insights into the potential for life beyond our planet. For instance, extensive research illustrates the incredible diversity of extremophiles and the various environmental conditions they occupy, reinforcing the concept that life can endure and thrive in realms that were previously thought to be completely inhospitable to any form of biological activity. This remarkable adaptability suggests that similar life forms could plausibly exist on other celestial bodies, such as Mars, which harbors extreme cold and radiation, or the icy moon Europa, which has a subsurface ocean beneath its frozen crust. As researchers continue to probe these extreme environments here on Earth, they not only enhance our understanding of the limits of life as we know it but also lay the crucial groundwork for future space missions aimed at discovering extraterrestrial microbial life in environments that echo the extremophilic conditions identified in our own biosphere. In doing so, they help to illuminate the broader possibilities of life’s existence throughout the cosmos.
Location | Microbial Species | Discovery Year | Key Findings |
Deep Ocean Hydrothermal Vents | Methanogens, Sulfate-Reducing Bacteria | 1977 | These organisms thrive in extreme temperatures (>100°C) and high-pressure environments, utilizing chemosynthesis. |
Hot Springs (Yellowstone National Park) | Thermophiles, Acidophiles | 1966 | Thermophiles can survive temperatures up to 121°C; the study of these organisms helps in understanding potential life on other planets. |
Martian Surface (NASA Curiosity Rover) | Hypothetical Microbial Life | 2012 | Evidence of ancient microbial life is suggested through the detection of organic molecules and methane fluctuations. |
Antarctic Dry Valleys | Psychrophiles | 1998 | Microbial life found in extreme cold, with potential analogs for life on icy moons like Europa. |
Microbial Life in Extreme Environments
A. Can Bacteria & Viruses Survive in Space?
The survival of bacteria and viruses in the extreme conditions of space has garnered significant scientific interest, emphasizing their remarkable adaptability and resilience in the face of seemingly inhospitable environments. Extensive research indicates that certain extremophiles, a category of microorganisms known for thriving in extreme conditions on Earth, possess specialized mechanisms that allow them to withstand desiccation, radiation, and the vacuum of space—factors that are prevalent in the harsh expanse of outer space. For instance, bacteria such as *Deinococcus radiodurans* exhibit exceptional resistance to ionizing radiation, a phenomenon that would be detrimental to most life forms, thus strongly supporting the hypothesis that life could potentially endure and even flourish in extraterrestrial environments. Moreover, studies have shown that some viruses can maintain their infectivity despite prolonged exposure to harsh space conditions, raising intriguing questions about the possibilities of interplanetary transfer of life forms. Scientists are particularly fascinated by how these microorganisms might survive the journey through the void of space, which opens new avenues for understanding life’s resilience. This resilience is critical for astrobiological investigations, as it provides insight into the potential for life to exist beyond Earth, perhaps even on planets or moons that were previously considered uninhabitable. As highlighted in the illustrative diagram of extremophiles in various environments, these organisms represent a pivotal component of ecological studies, serving as analogs for evaluating the potential for life on other celestial bodies, including Mars and the icy moons of Jupiter and Saturn. Their study not only deepens our understanding of life’s tenacity but also shapes future explorations and missions aimed at discovering life beyond our planet.
Table – Can Bacteria & Viruses Survive in Space? (Detailed Analysis)
Factor | Impact on Microbial Survival | Examples & Findings | Significance & Implications |
---|---|---|---|
Microgravity | Alters bacterial growth, enhances biofilm formation, increases mutation rates, and affects antibiotic resistance. | – Salmonella typhimurium became more virulent in space aboard the ISS. – Pseudomonas aeruginosa showed increased biofilm formation, making it harder to eliminate. | – Raises concerns for astronaut health and microbial infections during long-term space missions. – Can help develop better treatments for resistant bacterial infections. |
Vacuum & Low Pressure | Extreme desiccation leads to cellular water loss, causing dormancy or cell death. Some microbes survive in spore form. | – Bacillus subtilis spores survived vacuum exposure. – Deinococcus radiodurans endured direct space conditions in the EXPOSE experiment. | – Suggests that microbes could survive interplanetary transfer (panspermia hypothesis). – Raises concerns about contamination of other celestial bodies. |
High Radiation Exposure | Cosmic and UV radiation damage DNA, proteins, and cell membranes, but some microbes have exceptional repair mechanisms. | – Deinococcus radiodurans and Thermococcus gammatolerans can repair DNA after extreme radiation exposure. – Bacillus spores showed radiation resistance when shielded by dust particles. | – Enhances understanding of radiation-resistant life and its potential for surviving on Mars or Europa. – Helps in developing radiation-shielding strategies for space travel. |
Extreme Temperatures | Space temperatures range from near absolute zero (-270°C) to intense heat (~250°C in sunlight). | – Some extremophiles, like Thermococcus species, tolerate extreme heat. – Antarctic microbes thrive in cold environments, offering insights into survival in icy moons. | – Aids astrobiology research on potential life on Mars, Europa, and Enceladus. – Useful in biotechnology and cryopreservation studies. |
Nutrient Scarcity | Space environments lack organic nutrients, forcing microbes into dormancy. | – Lyophilized (freeze-dried) bacteria remained viable for years in space. – Bacillus spores can survive long periods of dormancy and reactivate in favorable conditions. | – Supports theories that microbial life could exist in dormant forms on Mars or other planets. – Could influence strategies for microbial-based biomanufacturing in space. |
Planetary Contamination Risks | Earth microbes could contaminate other planets or extraterrestrial microbes could affect Earth. | – NASA’s planetary protection protocols sterilize spacecraft to prevent forward contamination. – Tersicoccus phoenicis, a highly resistant microbe, was found in spacecraft assembly rooms. | – Ensures the integrity of astrobiology missions seeking extraterrestrial life. – Could influence space colonization ethics and biological containment policies. |
Viruses in Space | Viruses can remain viable in space when shielded but require a host to replicate. Spaceflight affects viral reactivation. | – Latent herpes viruses were reactivated in astronauts due to stress and immune suppression. – Some viral particles remained stable in spaceflight environments. | – Raises concerns about astronaut health and immune system function in space. – Could impact medical preparedness for long-duration missions to Mars. |
Biofilm Formation & Spacecraft Hygiene | Space conditions promote biofilm formation, making microbial communities harder to remove. | – Pseudomonas aeruginosa formed thicker biofilms in microgravity. – Staphylococcus aureus biofilms showed increased antibiotic resistance. | – Challenges spacecraft hygiene and water purification systems. – Informs the development of antimicrobial coatings and cleaning methods for space habitats. |
Interplanetary Transfer of Life (Panspermia Hypothesis) | The possibility that life can be transferred between planets via meteorites or spacecraft. | – Bacterial spores survived re-entry conditions in experiments simulating meteoroid impacts. – Deinococcus radiodurans endured high radiation and extreme conditions, supporting panspermia theories. | – Suggests that life could spread between planets, influencing the search for extraterrestrial microbes. – Raises ethical concerns about contaminating celestial bodies with Earth microbes. |
Key Takeaways:
- Survival in Harsh Conditions: Some bacteria and viruses can survive extreme space conditions, including vacuum, radiation, and microgravity, particularly in dormant or biofilm states.
- Astronaut Health Risks: Space conditions can increase microbial virulence and antibiotic resistance, posing risks to long-term human space missions.
- Planetary Protection & Astrobiology: Studying microbial survival in space helps in the search for extraterrestrial life and informs policies to prevent contamination of other planets.
- Potential for Interplanetary Life Transfer: Findings support the panspermia hypothesis, which suggests microbial life could travel between planets via meteorites or spacecraft.
- Space Biotechnology Applications: Understanding microbial adaptation in space can lead to advancements in medicine, biotechnology, and sustainable space exploration.
These insights emphasize the importance of microbial research in space and its role in astrobiology, planetary protection, and future space missions.
B. NASA and the Search for Extraterrestrial Microbes
As NASA continues its relentless quest to uncover the possibilities of extraterrestrial life, the agency has strategically turned its attention to extreme environments found here on Earth, such as hydrothermal vents and acidic hot springs, using them as analogs for potential habitats that may exist beyond our planet. These unique and often harsh settings are home to extremophiles, which are remarkable organisms that can thrive under conditions previously thought to be inhospitable, thus offering critical insights into the remarkable adaptability of life in a variety of situations. By thoroughly studying these resilient microbial life forms, researchers are able to devise innovative methods aimed at detecting similar organisms on celestial bodies like Mars or the icy moons of Europa and Enceladus, which are believed to harbor subsurface oceans. The significance of this extensive research is underscored by various graphical representations of extremophiles in their diverse environments, such as that depicted in [citeX]. This diagram not only elucidates the diverse ecological niches that microorganisms occupy but also effectively illustrates the parallels that scientists draw between Earth’s extreme habitats and the potential ecosystems that may lie beyond our atmosphere. This comparison serves to enhance our understanding of life’s resilience and capacity to adapt across different worlds, moving us one step closer to answering one of humanity’s most profound questions: Are we alone in the universe? Consequently, these studies are not merely academic; they play a crucial role in preparing for future exploration missions that aim to search for signs of life beyond our own planet.
Mission | Objective | Launch Year | Status | Sample Return Potential |
Mars 2020 Perseverance Rover | Search for signs of ancient microbial life | 2020 | Ongoing | High |
Europa Clipper | Investigate the moon’s potential for life | 2024 | Upcoming | Medium |
Mars Sample Return | Return samples from Mars to Earth for analysis | 2028 | Planned | Very High |
Astrobiology Research Center | Study extremophiles on Earth to inform extraterrestrial life search | Ongoing (Established 1996) | Ongoing | N/A |
Jupiter Icy Moons Explorer (JUICE) | Explore Jupiter’s icy moons for habitability | 2023 | Upcoming | Medium |
NASA Extraterrestrial Microbe Research
C. The Role of Microbes in Space Missions (Bioreactors, Waste Recycling)
Microbes play an integral role in space missions, particularly in bioreactors and waste recycling systems, which address critical survival needs for long-duration missions. By utilizing extremophiles—microorganisms capable of thriving in harsh conditions—scientists can develop bioreactors that optimize biological processes for waste treatment and resource generation. These sophisticated systems convert organic waste into valuable resources, such as nutrients and potable water, which are essential for sustaining human life in extraterrestrial environments where traditional agriculture and water supply methods may not be feasible. Additionally, microbial metabolic pathways can be harnessed to generate oxygen and mitigate carbon dioxide levels, creating a more habitable atmosphere within spacecraft and enhancing crew comfort and safety. The advantage of employing these microorganisms becomes even more pronounced in the context of deep-space missions, where resupply from Earth would be nearly impossible. As research continues into the potential of these microorganisms, their remarkable adaptability can provide insights into the development of closed-loop life support systems that are necessary for successful missions to Mars and beyond. Understanding how these microbes operate and interact within bioreactors is crucial for optimizing their efficiency and effectiveness in resource recovery and waste management tasks. Furthermore, the diagram of extremophiles and their environments effectively underscores the relevance of such organisms in extreme space conditions, thereby enhancing our understanding of their potential applications in bioreactor technologies for future space exploration. This expansive knowledge not only paves the way for sustainable practices in space but also broadens our perspective on life sciences, potentially benefiting Earth’s own environmental challenges.
Mission | Microbe Type | Role | Year | Source |
Mars 2020 (Perseverance Rover) | Bacteria | Bioreactor for oxygen production | 2020 | NASA |
International Space Station (ISS) | Yeast | Waste recycling and nutrient production | 2011 | European Space Agency |
Bioregenerative Life Support System (BLSS) | Algae | Carbon dioxide absorption and oxygen release | 2026 | NASA |
Lunar Gateway | Archaea | Biodegradation of waste materials | 2024 | NASA |
Microbial Roles in Space Missions
V. Conclusion
In conclusion, the survival of bacteria and viruses in extreme environments underscores the remarkable adaptability of life on Earth and beyond. These microorganisms, often thriving in conditions previously deemed inhospitable, challenge our conventional understanding of biological limits. For instance, the ability of extremophiles to exist in hydrothermal vents and hot springs, as highlighted by the diagram in , reveals not only their biochemical resilience but also potential applications in biotechnology and astrobiology. Similarly, the diverse interactions among viruses and various microbial communities, depicted in , illustrate the intricate ecological networks that sustain life in these challenging habitats. Furthermore, understanding microbial life in extreme conditions has significant implications for the search for extraterrestrial life, aligning with findings from Mars analog studies. As research progresses, these insights will not only broaden our knowledge of microbial ecology but also inform the development of novel technologies that harness the unique capabilities of these organisms.
A. What Extremophiles Teach Us About Life’s Limits
The study of extremophiles reveals critical insights into the limits of life, challenging preconceived notions about the conditions necessary for survival. These microorganisms thrive in environments previously thought to be inhospitable, such as hydrothermal vents and acidic hot springs, where temperatures exceed 100 degrees Celsius and pH levels can be extremely low or high. By examining their metabolic processes, researchers uncover biochemical pathways that permit life in otherwise lethal settings, illuminating evolutionary resilience. This understanding extends beyond Earth, suggesting that life could potentially exist in extreme environments on other planetary bodies, such as Mars or the icy moons of Jupiter and Saturn. For instance, the diagram illustrating extremophilic conditions and microbial ecosystems clearly highlights how diverse habitats support life forms that adapt to extreme temperature and pressure variations. Such findings underscore the remarkable adaptability of life and expand our comprehension of biological possibilities, reshaping the criteria for what constitutes a habitable environment beyond our planet.
Extremophile Type | Habitat | Temperature Range (°C) | Examples | Significance |
Thermophile | Hot Springs | 50 – 122 | Thermus aquaticus | Enzymes from thermophiles are used in biotechnology. |
Psychrophile | Deep Oceans | -12 – 10 | Psychrobacter | Study of cold-adapted enzymes for biotechnological applications. |
Halophile | Salt Flats | 0 – 100 | Halobacterium | Research on unique adaptations to high salinity. |
Acidophile | Acidic Hot Springs | 25 – 75 | Ferroplasma | Understanding metal recovery processes. |
Alkaliphile | Alkaline Lakes | 20 – 60 | Natronobacterium | Insights into biogeochemical cycles. |
Extremophiles and Their Habitats
B. The Future of Astrobiology and Microbial Research
As research progresses into the realms of astrobiology and microbial ecology, a deeper understanding of extremophiles—organisms that thrive in extreme environments—becomes increasingly pivotal. Investigations into life forms residing in hot springs, deep oceans, and even extraterrestrial landscapes have the potential to reshape our comprehension of lifes adaptability and resilience. Microbial research, particularly regarding the metabolic pathways of these organisms, highlights their applications in biotechnology, including bioremediation and pharmaceutical developments. The exploration of microbial biogeography, as depicted in the diagram of extremophilic conditions , offers crucial insights into how these organisms may inform our search for life on other planets. The adaptability of extremophiles not only illuminates the conditions necessary for life but also raises essential questions about the potential for life beyond Earth, emphasizing the critical intersection between astrobiology and microbial research in shaping our understanding of lifes potential across the cosmos.
The Future of Astrobiology and Microbial Research
Aspect | Description | Potential Impact & Examples |
---|---|---|
Search for Extraterrestrial Life | Investigating microbial life on other planets and moons using advanced space missions. | – NASA’s Perseverance Rover searching for biosignatures on Mars. – Europa Clipper mission exploring Jupiter’s moon Europa for possible subsurface microbial life. |
Extremophiles & Habitability | Studying microbes that thrive in extreme environments on Earth to understand potential alien life. | – Deinococcus radiodurans surviving high radiation levels, suggesting possible Martian life. – Hydrothermal vent microbes helping redefine life’s potential habitats. |
Panspermia Hypothesis | Exploring the idea that life on Earth originated from microbial life transported via comets or meteoroids. | – Experiments on the International Space Station (ISS) showing microbial survival in space. – Research on meteorite composition and ancient microbial fossils. |
Synthetic Biology & Space Colonization | Engineering microbes for survival in space, resource production, and terraforming. | – Genetically modified bacteria producing oxygen and food for Mars colonization. – Bioremediation using microbes to recycle waste on long-term space missions. |
Microbial Evolution in Space | Studying how microorganisms adapt to microgravity and space radiation. | – Escherichia coli experiments on the ISS showing increased antibiotic resistance. – Yeast and bacterial mutations in space informing planetary protection policies. |
Exoplanet Biosignatures | Detecting chemical markers of life on exoplanets using advanced telescopes. | – James Webb Space Telescope (JWST) analyzing planetary atmospheres for methane and oxygen. – Future space missions targeting habitable-zone exoplanets. |
Impact of Space Travel on Human Microbiome | Understanding how long-duration spaceflight affects human-associated microbes and health. | – Changes in astronaut gut microbiomes during extended ISS missions. – Increased virulence of pathogens like Salmonella in microgravity. |
Planetary Protection & Contamination Control | Preventing contamination of other planets with Earth microbes and vice versa. | – Stringent sterilization protocols for Mars landers and rovers. – COSPAR (Committee on Space Research) guidelines for preventing forward and backward contamination. |
Future Research & Technological Advances | Advancing tools and techniques for microbial detection and space exploration. | – AI-powered biosensors detecting microbial life in extraterrestrial environments. – CRISPR-based genetic tools for studying microbial adaptation in space. |
REFERENCES
- Howell G. M. Edwards. ‘Life in Extreme Environments.’ Insights in Biological Capability, Guido di Prisco, Cambridge University Press, 10/15/2020
- Vivek Sharma. ‘Physiological and Biotechnological Aspects of Extremophiles.’ Richa Salwan, Academic Press, 6/4/2020
- Constance M. Bertka. ‘Exploring the Origin, Extent, and Future of Life.’ Philosophical, Ethical and Theological Perspectives, Cambridge University Press, 9/3/2009
- Alexander Choukèr. ‘Stress Challenges and Immunity in Space.’ From Mechanisms to Monitoring and Preventive Strategies, Springer Nature, 11/27/2019
- Anuj K. Chandel. ‘Extremophiles and Their Applications in Medical Processes.’ Prasanti Babu, Springer, 10/29/2014
- Neil deGrasse Tyson. ‘Cosmic Horizons.’ Astronomy at the Cutting Edge, Steven Soter, New Press, 1/1/2001
- Clementina Sansone. ‘Marine Algal Antioxidants.’ Christophe Brunet, MDPI – Multidisciplinary Digital Publishing Institute, 1/1/2020
- Christon J. Hurst. ‘Viral Ecology.’ Academic Press, 5/2/2000
- Georges Fournier. ‘Light Scattering by Particles in Water.’ Theoretical and Experimental Foundations, Miroslaw Jonasz, Elsevier, 8/29/2011
- Paul A. Tyler. ‘Deep-Sea Biology.’ A Natural History of Organisms at the Deep-Sea Floor, John D. Gage, Cambridge University Press, 4/18/1991
- Jakob K. Kristjansson. ‘Thermophilic Bacteria.’ CRC Press, 1/31/2021
- Pabulo H. Rampelotto. ‘Extremophiles and Extreme Environments.’ Mdpi AG, 10/1/2018
- Alistair McCleery. ‘An Introduction to Book History.’ David Finkelstein, Routledge, 3/13/2006