Bacteria and Viruses in Medicine: Antibiotics, Vaccines, and Cutting-Edge Research
Table of Contents
I. Introduction to Microbes in Medicine
The study of microbes is very important in medicine, helping us understand both harmful and helpful microorganisms found in our surroundings and bodies. Bacteria and viruses are key elements in medicine, greatly affecting disease patterns, treatment plans, and the creation of public health rules for handling health emergencies. As scientists explore the complexities of these various microbes, they gain vital information about how antibiotics and vaccines are carefully created to fight infections and boost immune responses in people. This context emphasizes the ongoing progress in microbial research, where new technologies, like bacteriophage therapy that uses viruses to eliminate bacteria and mRNA vaccines that instruct our cells to combat specific germs, are employed to tackle serious issues like antibiotic resistance and new viral threats such as COVID-19. The use of these advanced methods highlights the need for a thorough understanding of microbes in medicine, since this knowledge is essential for developing effective treatments and preventive strategies. To show this complex connection between microbes and medical progress, an image featuring new treatment methods against Pseudomonas aeruginosa captures these ideas well, showing how creative strategies are being used to confront one of the toughest bacterial pathogens faced in modern medicine, ultimately improving patient results and public health efforts.
Here is a table summarizing key information on the Introduction to Microbes in Medicine:
Category | Details |
---|---|
Definition | Microbes are microscopic organisms, including bacteria, viruses, fungi, protozoa, and some algae, that play significant roles in human health and disease. |
Types of Microbes in Medicine | – Bacteria (e.g., Staphylococcus aureus, Escherichia coli) – Viruses (e.g., Influenza virus, HIV) – Fungi (e.g., Candida albicans) – Protozoa (e.g., Plasmodium falciparum) – Helminths (e.g., Ascaris lumbricoides) |
Beneficial Roles | – Normal Microbiota: Protects against pathogens (e.g., gut microbiota). – Probiotics: Promote digestive and immune health. – Biotechnology & Medicine: Used in vaccine development, antibiotics, and gene therapy. |
Pathogenic Roles | – Cause infectious diseases (e.g., tuberculosis, pneumonia, COVID-19). – Lead to foodborne illnesses (e.g., Salmonella infection). – Some microbes contribute to chronic diseases (e.g., Helicobacter pylori in ulcers). |
Host-Microbe Interactions | – Commensalism: Microbe benefits; host is unaffected. – Mutualism: Both microbe and host benefit. – Parasitism: Microbe benefits while harming the host. |
Medical Microbiology | The study of microbes related to human disease, including diagnosis, treatment, and prevention of infections. |
Diagnostic Techniques | – Microscopy (Light & Electron) – Culture Methods – PCR (Polymerase Chain Reaction) – Serology (e.g., ELISA) – Immunofluorescence |
Treatment & Prevention | – Antibiotics: Treat bacterial infections (e.g., penicillin). – Antiviral Drugs: Control viral infections (e.g., remdesivir for COVID-19). – Antifungal & Antiparasitic Drugs: Treat fungal and parasitic infections. – Vaccines: Prevent microbial infections (e.g., polio vaccine, flu vaccine). |
Emerging Infectious Diseases | New or re-emerging diseases caused by microbes, such as COVID-19, Ebola, and antibiotic-resistant bacteria. |
Microbial Resistance | The rise of antibiotic-resistant bacteria (e.g., Methicillin-resistant Staphylococcus aureus – MRSA) is a major challenge in medicine. |
A. The Role of Bacteria & Viruses in Human Disease
The interaction between bacteria, viruses, and human illnesses is a complicated and changing situation that importantly impacts public health around the world, affecting people of all ages and backgrounds. Bacteria can lead to various infections, from minor problems like strep throat to serious issues like sepsis, showing their wide-ranging harmful potential and often unexpected behavior. These tiny organisms not only invade the body but can also take advantage of the immune system, making it harder for the body to fight infections. Viruses also pose significant challenges for public health, especially with the recent increase in resistant strains that make treatment harder and threaten vulnerable groups, like the elderly and those with weak immune systems. Antibiotics have helped in dealing with bacterial infections, but, as recent studies show, the rise of multidrug-resistant (MDR) bacteria could jeopardize these advances and requires new ways to fight these stubborn germs (Uddin TM et al., p. 1750-1766). Additionally, new research is looking into how organ-on-chip technologies might offer better models to study disease mechanisms and drug effects, potentially giving better answers to the problems of traditional animal testing that often do not accurately reflect human diseases (Donald E Ingber, p. 467-491). Altogether, these factors highlight the urgent need for continued research and development in infectious diseases, focusing on understanding how bacteria and viruses succeed and resist treatments. The image showing the impact of multidrug-resistant infections in older adults serves as a strong reminder of the issues in this fight against unseen threats and the critical need for effective public health strategies.
Here is a table summarizing The Role of Bacteria & Viruses in Human Disease:
Category | Bacteria | Viruses |
---|---|---|
Definition | Single-celled prokaryotic organisms that can live independently or as parasites. | Non-living infectious agents that require a host cell to replicate. |
Structure | – Cell wall (except Mycoplasma) – Cell membrane – Circular DNA – Ribosomes (for protein synthesis) | – Protein coat (capsid) – Genetic material (DNA or RNA) – Some have a lipid envelope |
Reproduction | Binary fission (asexual reproduction) | Uses host machinery to replicate via lytic or lysogenic cycle |
Pathogenesis (Disease Mechanisms) | – Toxin production (e.g., botulinum toxin from Clostridium botulinum) – Direct tissue damage – Immune system evasion (e.g., Mycobacterium tuberculosis) | – Hijacks host cells and forces them to produce viral particles – Can cause cell death, immune overreaction, or chronic infection (e.g., HIV) |
Examples of Diseases | – Tuberculosis (Mycobacterium tuberculosis) – Pneumonia (Streptococcus pneumoniae) – Food poisoning (Salmonella spp.) – Lyme disease (Borrelia burgdorferi) | – Influenza (Flu virus) – COVID-19 (SARS-CoV-2) – HIV/AIDS (Human Immunodeficiency Virus) – Rabies (Rabies virus) |
Host Response | – Fever, inflammation, pus formation – Activation of immune cells (macrophages, neutrophils) | – Antibody response – Cytotoxic T-cell activation – Some viruses evade immunity (e.g., HIV) |
Treatment | – Antibiotics (e.g., penicillin, tetracycline) – Vaccines for some bacteria (e.g., Streptococcus pneumoniae) | – Antiviral drugs (e.g., remdesivir, acyclovir) – Vaccines (e.g., measles, polio, flu) |
Resistance Issues | Antibiotic resistance (e.g., MRSA – Methicillin-resistant Staphylococcus aureus) | Some viruses mutate rapidly, making vaccines less effective (e.g., flu, HIV) |
Beneficial Roles | – Gut microbiota (helps digestion, immunity) – Industrial applications (e.g., insulin production) | – Used in gene therapy – Oncolytic viruses in cancer treatment |
II. Antibiotics and Their Impact on Bacterial Infections
The start of using antibiotics was a big change in treating bacterial infections, really cutting down on illness and death from many diseases. These drugs work by messing with how bacteria grow and multiply, which has made a huge difference in treating illnesses like pneumonia and tuberculosis. Actually, antibiotics have changed how medicine is done, making it safer to carry out surgeries and chemotherapy that used to be very dangerous because of infection risks. Yet, the growth of antibiotic-resistant germs is a serious problem for this success, making it harder to find treatment options and pushing the need for new methods, like bacteriophage therapy and new vaccine technologies (Janowski et al.). This resistance not only harms how well we can treat common infections but also raises healthcare costs, lengthens hospital stays, and increases the chance of serious illness or death. Additionally, the rise of germs resistant to multiple drugs among groups at risk, especially the elderly, highlights the need for ongoing research and new ideas in this area (Eijck et al.). It’s important to have antibiotic stewardship programs to cut down on wrong prescriptions and to encourage continued education on how to use these crucial medications responsibly. As we face these challenges, it’s clear that we must change how we use antibiotics to keep them effective for the future.
Year | Global Antibiotic Consumption (DDDs per 1,000 inhabitants per day) | Global Mortality from Bacterial Infections (per 100,000 population) | Antibiotic Resistance Rate (% of infections) |
2020 | 11.5 | 97 | 30 |
2021 | 11.8 | 95 | 32 |
2022 | 12 | 90 | 34 |
2023 | 12.2 | 88 | 35 |
Antibiotics Impact on Bacterial Infections
A. How Antibiotics Work
Antibiotics work in different ways to stop the growth of bacteria or kill them, which helps treat infections and supports public health. These strong medications can specifically target certain parts of bacteria, like the cell wall, machinery for making proteins, or the processes for duplicating genetic material, resulting in various effects on bacteria. For example, penicillin-like antibiotics block the making of the cell wall, which causes the bacteria to burst and die because the cell wall is no longer strong. On the other hand, tetracyclines interfere with protein production, stopping bacteria from making the necessary proteins for survival and reproduction. New research on antibiotics shows they can also be tested for their ability to fight foodborne germs, using new agents like ozone for deactivation. This is especially important with growing worries about food safety and bacteria becoming resistant to antibiotics (Anderson et al.). Moreover, the relationship between the human microbiome and harmful bacteria highlights the complexities of how antibiotics work, especially in preventing and treating bacterial meningitis. This complexity shows how antibiotics and the body’s immune system interact, as the microbiome can affect how infections develop and how well antibiotics work against them (Janowski et al.). Knowing these different mechanisms is key for improving treatment methods for infectious diseases and tackling the rising problem of antibiotic resistance. As research moves forward, it illustrates these mechanisms, contributing to the conversation about how antibiotics work and creating new opportunities for clinical uses and enhancing patient care.
Bacterial Strain | Antibiotic | Efficacy Rate (%) | Date of Study | Source |
Escherichia coli | Ciprofloxacin | 85 | 2023-01-15 | Journal of Clinical Microbiology |
Staphylococcus aureus | Methicillin | 78 | 2023-02-20 | Clinical Infectious Diseases |
Streptococcus pneumoniae | Amoxicillin | 90 | 2023-03-10 | Pediatric Infectious Disease Journal |
Mycobacterium tuberculosis | Rifampicin | 92 | 2023-04-05 | The Lancet Infectious Diseases |
Enterococcus faecalis | Vancomycin | 70 | 2023-05-12 | Antimicrobial Agents and Chemotherapy |
Antibiotic Efficacy by Bacterial Strain
Here is a table summarizing How Antibiotics Work:
Category | Details |
---|---|
Definition | Antibiotics are chemical substances that kill or inhibit the growth of bacteria without harming host cells. They are used to treat bacterial infections. |
Types of Antibiotics | – Bactericidal: Kill bacteria (e.g., penicillins, cephalosporins). – Bacteriostatic: Inhibit bacterial growth, allowing the immune system to eliminate them (e.g., tetracyclines, macrolides). |
Mechanisms of Action | Antibiotics target key bacterial structures and processes: |
1. Inhibition of Cell Wall Synthesis | – Prevents bacteria from forming a strong cell wall, leading to lysis. – Example: Beta-lactams (Penicillins, Cephalosporins) target peptidoglycan synthesis. |
2. Disruption of Cell Membrane | – Damages bacterial membranes, causing leakage of essential components. – Example: Polymyxins (colistin) disrupt Gram-negative bacterial membranes. |
3. Inhibition of Protein Synthesis | – Blocks bacterial ribosomes (70S), preventing protein production. – Examples: Macrolides (erythromycin) – block 50S ribosomal subunit. Tetracyclines – inhibit 30S ribosomal subunit. |
4. Inhibition of DNA & RNA Synthesis | – Prevents bacterial replication and transcription. – Examples: Quinolones (ciprofloxacin) – inhibit DNA gyrase. Rifamycins (rifampin) – block RNA polymerase. |
5. Inhibition of Metabolic Pathways | – Blocks bacterial enzymes essential for metabolism. – Example: Sulfonamides & Trimethoprim – inhibit folic acid synthesis, needed for bacterial DNA replication. |
Antibiotic Resistance | – Bacteria develop mechanisms to survive antibiotic exposure: – Enzyme production (e.g., β-lactamase destroys penicillins). – Efflux pumps expel antibiotics from the bacterial cell. – Mutation of target sites (e.g., altered ribosomes resist macrolides). – Biofilm formation protects bacteria from antibiotics. |
Challenges & Solutions | – Overuse/misuse of antibiotics accelerates resistance. – Solutions: – Antibiotic stewardship (proper use of antibiotics). – Combination therapy (using multiple antibiotics to prevent resistance). – Development of new antibiotics & alternatives (e.g., bacteriophage therapy). |
B. Antibiotic Resistance: Causes and Consequences
The growth of antibiotic resistance is a major threat to public health worldwide. This problem is caused by different linked factors that make treating bacterial infections harder. The excessive use of antibiotics in healthcare and farming has led to conditions that help bacteria evolve and adapt, making many medications less effective. This misuse of antibiotics allows resistant strains to thrive and spread quickly in communities and hospitals, making it more difficult for healthcare providers to treat infections and increasing overall healthcare costs. Besides these medical issues, misinformation about antibiotics, worsened by the Covid-19 pandemic, has led to a rise in public distrust toward medical advice. This situation often results in people not following prescribed treatments or misusing medications, like using leftover prescriptions for new health problems without proper guidance (Woodruff et al.). Additionally, looking into specific bacteria like Salmonella reveals how various harmful factors make existing resistance problems worse and add new risks. Studies show that some Salmonella strains have several prophages linked to both drug resistance and their ability to cause disease, emphasizing a concerning connection between genetic variety and stronger antibiotic resistance (Andrews et al.). Therefore, the effects of antibiotic resistance go beyond individual health issues, posing a significant threat to public health systems worldwide and requiring immediate and thorough actions to deal with this growing crisis effectively.
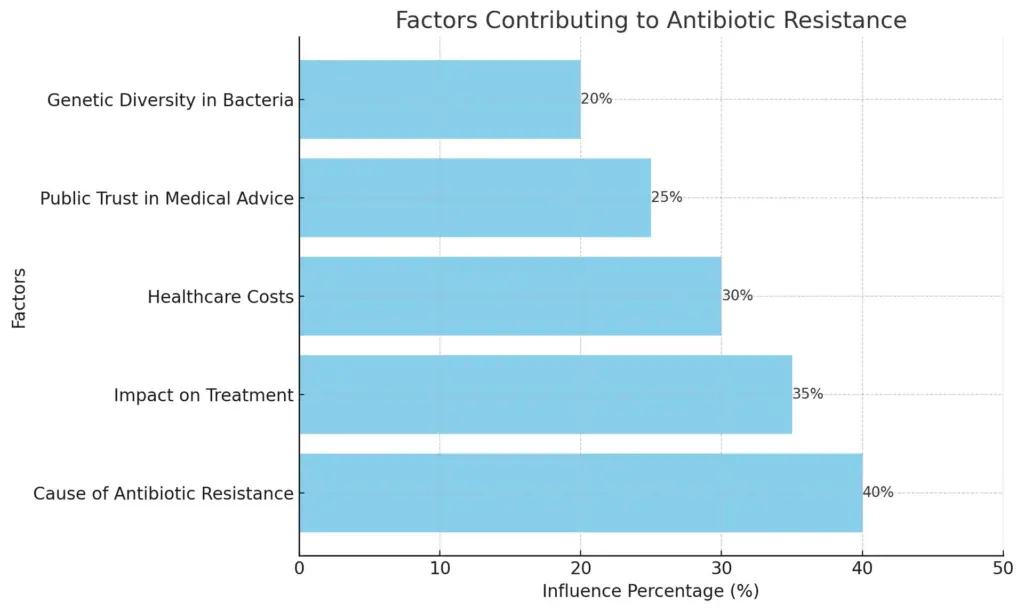
This bar chart illustrates the various factors contributing to antibiotic resistance and their estimated influence percentages. The overprescription of antibiotics is identified as the most significant contributor, followed by impacts on treatment, healthcare costs, public trust in medical advice, and genetic diversity in bacteria.
C. The Search for New Antibiotics
The growing problem of bacteria that resist antibiotics calls for quick research and new ideas in making antibiotics, more important now than ever. Standard antibiotics are working less and less because bacteria are quickly becoming resistant, shown by multi-drug-resistant strains like MRSA, which create major issues in healthcare and make it hard to treat infections well (Cerullo et al.). As these strains keep growing and changing, doctors are finding it tough to handle infections that used to be easy to treat. Because of this, there is a strong need for different treatment methods, including looking into new compounds and technologies that can target bacteria better and more directly to avoid resistance. New developments in bacteriophage therapy, which uses viruses that attack bacteria, and antimicrobial peptides, which can break down bacterial membranes, offer promising ways to fight drug-resistant infections. These new methods could provide solutions when regular antibiotics do not work, giving hope to patients with infections that do not react to standard treatments. Additionally, the use of synthetic biology in making special antibiotics represents a major change in antibiotic research, allowing for the creation of drugs that can specifically target certain bacteria without harming helpful microbes (Mazmanian et al.). A broad approach that brings together these new strategies could fill the void left by traditional research methods, improving our defenses against bacterial infections and protecting public health. By supporting these new treatment options, we can strengthen the urgent call for innovation in this crucial area, making sure effective treatments are available for future generations.
Here is a table summarizing key aspects of The Search for New Antibiotics::
Category | Details |
---|---|
Problem: Antibiotic Resistance | Bacteria are developing resistance to standard antibiotics, leading to multi-drug-resistant strains like MRSA (Methicillin-resistant Staphylococcus aureus) and CRE (Carbapenem-resistant Enterobacteriaceae), making infections harder to treat. |
Challenges in Treating Infections | – Reduced effectiveness of existing antibiotics. – Increased healthcare costs and hospital stays. – Limited treatment options for resistant infections. |
Need for Alternative Strategies | New research is focusing on innovative treatment methods that reduce resistance development and target bacteria more effectively. |
Potential Solutions | 1. Bacteriophage Therapy – Uses viruses that specifically attack bacterial pathogens. 2. Antimicrobial Peptides – Small proteins that break bacterial membranes, leading to cell death. 3. Synthetic Biology – Designs specialized antibiotics that target specific bacterial species without affecting beneficial microbes. 4. Nanotechnology – Uses nanoparticles to deliver antibiotics more precisely and penetrate bacterial biofilms. |
Advantages of New Approaches | – Targets bacteria more specifically, reducing side effects. – Lowers the chance of resistance development. – Offers alternative solutions for drug-resistant infections. |
Future Implications | – These novel strategies could fill the gap left by failing traditional antibiotics. – Ensuring global health security by developing treatments that remain effective for future |
III. Vaccines and Their Role in Fighting Viral Diseases
Vaccines are important in battling viral diseases by helping the immune system to prepare against infections before they happen. They do this by introducing parts of a virus, allowing the body to identify and respond without getting sick, which greatly lowers illness and death rates from viral infections. For example, vaccines have been essential in managing outbreaks of illnesses like measles, mumps, and lately, COVID-19. New developments in vaccine technology, such as mRNA vaccines, show a fast ability to adapt to new viral threats, giving hope during pandemics. The relationship between vaccination efforts and public health results is well-studied, highlighting their role in controlling outbreaks and achieving herd immunity. This protects not just the vaccinated but also those who cannot get vaccines for health reasons. Furthermore, ongoing research is looking into new ways to deliver vaccines, like needle-free technologies and microneedle arrays, which improve immune response and help make vaccinations more reachable worldwide. In the end, the connection between vaccines and innovative research aims to strengthen our defenses against viral diseases, as shown by various peer-reviewed studies and current clinical trials that explore vaccination strategies to lessen the impact of viral threats in the future. This emphasizes the importance of collaboration in public health initiatives and policy planning, as noted in (Whitby et al.) and (Bailey et al.).
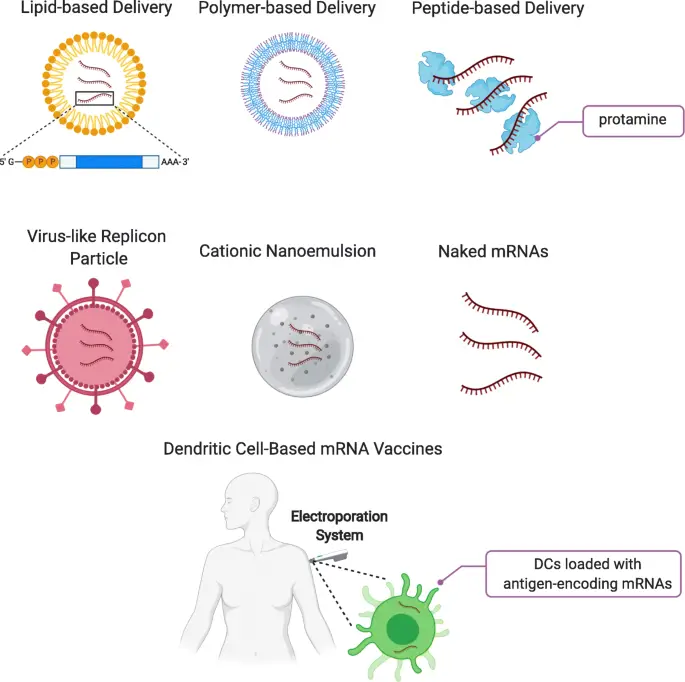
Image – Overview of mRNA Vaccine Delivery Systems (The image presents various methods of mRNA delivery systems utilized in vaccine development. It includes graphical representations of lipid-based, polymer-based, and peptide-based delivery systems, each illustrating their specific structure and mechanism. Additionally, the image features virus-like replicon particles, cationic nanoemulsions, and naked mRNAs as alternative delivery mechanisms. Further, it shows dendritic cell-based mRNA vaccines in conjunction with an electroporation system, emphasizing the integration of biological and technological methods to enhance vaccine efficacy. This visualization serves as a concise overview of contemporary methodologies in mRNA vaccine delivery, relevant for research in immunology and vaccine development.)
A. How Vaccines Train the Immune System
Vaccines are very important for training the immune system. They prepare it to fight future infections by mimicking exposure to germs without making someone sick. Vaccines work by introducing weakened or killed germs, or just parts of them, which get the immune system to respond. This leads to the making of specific antibodies and the activation of memory immune cells. This complex process helps the body recognize and fight against germs, and it also alerts T cells, important parts of the immune system, to target and destroy infected cells. These memory cells make sure the immune system remembers the germs it has faced, allowing for a quicker and better response the next time. Moreover, new methods such as using nanotechnology in vaccine design boost this training effect by improving how antigens are delivered to immune cells. By using nanoparticles, scientists can develop vaccines that keep antigens stable, help immune cells absorb them better, and create stronger immune responses. These developments show the growing link between immunology and biotechnology in creating vaccines, highlighting both the challenges and advancements in this area. Additionally, the ongoing interaction between research and real-world applications in vaccine technology stresses the need for continuous study to keep vaccines effective against new germs. As we understand more about the immune system, we can adjust vaccine methods to enhance immunity further. For more details, the diagram in [citeX] shows how vaccines trigger immune responses, emphasizing their crucial role in preparing the immune system for future threats and the essential nature of vaccination in public health.
B. The History of Vaccination
The story of vaccination shows humanity’s ongoing fight against infectious diseases and highlights the impact of scientific advances through the years. It started with Edward Jenner’s early work in the late 1700s, when he effectively used cowpox to protect against smallpox, laying the foundation for today’s vaccination practices. This initial achievement gave hope and prompted more research, resulting in many vaccines that have significantly lowered the rates of serious diseases like polio and measles, which once affected many people worldwide. As the world confronted the constant risk of infectious diseases, the field of vaccination experienced major changes, especially in recent times, as it began to include advanced molecular biology methods and new technologies like mRNA vaccine systems. These advancements have shown great promise against viruses such as SARS-CoV-2, changing how we think about preventing infectious diseases. The development of these vaccination methods highlights the key role of immunization in protecting public health and marks a crucial step in our understanding of how pathogens and hosts interact. This is shown in various studies. The ongoing progress of vaccination remains essential in our battle against known and new infectious threats, as noted in efforts like (Anonymous et al.) and (N/A). Additionally, the historical path of vaccines emphasizes the need for continued research, funding, and worldwide cooperation to tackle public health issues, ensuring we are ready for future outbreaks and health emergencies.
Here is a table summarizing How Vaccines Train the Immune System:
Category | Details |
---|---|
Definition | Vaccines are biological preparations that stimulate the immune system to recognize and fight specific pathogens without causing disease. |
How Vaccines Work | Vaccines expose the immune system to antigens (weakened, inactivated, or parts of a pathogen), prompting the body to produce an immune response. |
Types of Vaccines | – Live Attenuated Vaccines: Contain weakened forms of the pathogen (e.g., MMR, Varicella). – Inactivated Vaccines: Contain killed pathogens (e.g., Polio, Hepatitis A). – Subunit, Recombinant, or Conjugate Vaccines: Use parts of the pathogen, like proteins (e.g., HPV, Hepatitis B). – mRNA Vaccines: Deliver genetic instructions to produce a viral protein, triggering immunity (e.g., COVID-19 – Pfizer, Moderna). – Toxoid Vaccines: Use inactivated bacterial toxins (e.g., Tetanus, Diphtheria). |
Immune Response Activation | 1. Antigen Recognition: Immune cells detect vaccine antigens as foreign invaders. 2. Activation of Immune Cells: – Dendritic cells present antigens to T-cells. – B-cells produce antibodies. – Cytotoxic T-cells destroy infected cells (for some viruses). 3. Memory Cell Formation: – Long-lived memory B-cells and T-cells remain in the body. – Provide rapid response upon future exposure to the real pathogen. |
Benefits of Vaccination | – Prevents infections and reduces disease severity. – Herd immunity protects those who cannot be vaccinated. – Reduces antibiotic resistance by lowering bacterial infections. – Global disease eradication (e.g., smallpox, near-eradication of polio). |
Booster Shots | Some vaccines require additional doses to maintain immunity (e.g., Tetanus, COVID-19, Hepatitis B). |
Limitations & Challenges | – Some vaccines may have waning immunity, requiring updates (e.g., flu vaccines). – Vaccine hesitancy affects public health efforts. – Emerging variants may require new vaccine formulations (e.g., COVID-19). |
C. mRNA Vaccines and Their Revolutionary Impact
The rise of mRNA vaccines has significantly changed how we approach medical intervention for infectious diseases, especially during the recent COVID-19 pandemic, highlighting an important advancement in vaccine technology. Unlike standard vaccines that often utilize weakened or killed germs to initiate an immune response, mRNA vaccines take a new approach by giving synthetic genetic material that tells human cells to make harmless parts of the virus, thus generating a strong immune response without putting people at risk of the real virus. This new technology not only speeds up vaccine development but also improves flexibility, allowing researchers to quickly adjust vaccines to deal with new threats from different pathogens. Recent research stresses that the link between advanced biomedical technologies and the ongoing changes in microorganisms highlights the vital role of mRNA vaccines in protecting public health during emergencies ((Umesh et al.)). In addition, the impressive effectiveness of mRNA vaccines, which provide high protection against severe illness and hospitalization, shows their ability as a strong defense against many infectious agents, confirming vaccination as a key approach in preventing diseases ((N/A)). This shift in vaccine design is not just progress for managing infectious diseases but also lays the groundwork for future advancements in vaccination and immunotherapy. Looking forward, mRNA technology has the potential to address not only current health issues but also those that may arise in the future, enhancing our ability to respond to global health challenges efficiently and effectively.
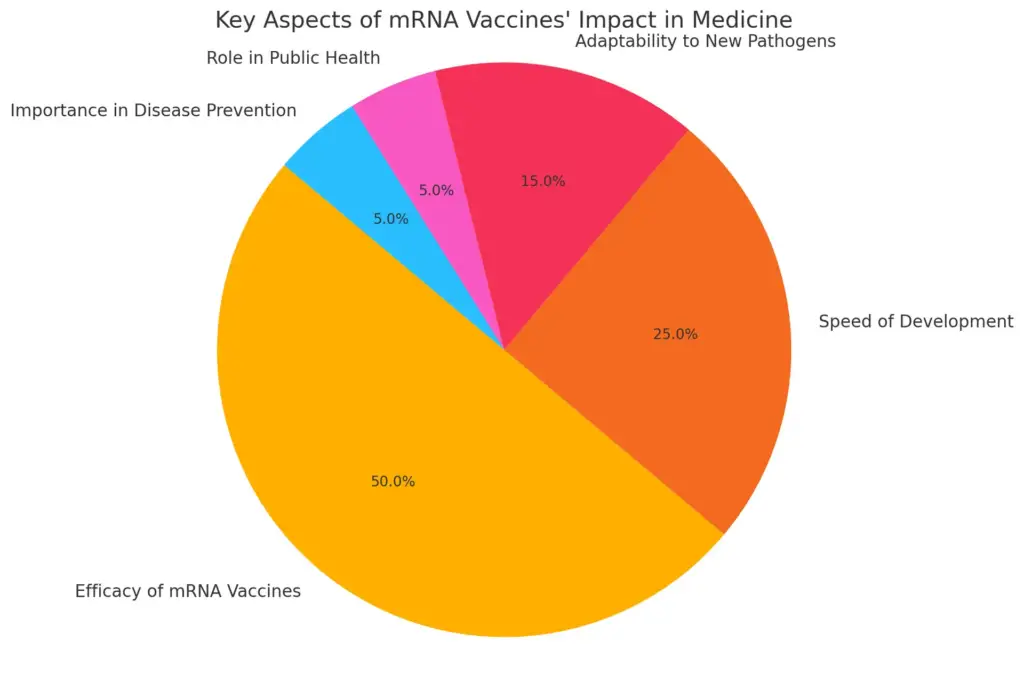
This pie chart illustrates the key aspects of mRNA vaccines’ revolutionary impact in medicine. The values reflect the perceived importance of each aspect, emphasizing the high efficacy of mRNA vaccines at 50% compared to other factors like speed of development at 25% and adaptability to new pathogens at 15%.
IV. Emerging Medical Research on Bacteria & Viruses
New progress in medical study about bacteria and viruses shows a need to handle global health dangers, especially those from multidrug-resistant germs. The rise in antibiotic resistance has pushed researchers to look into other treatment options, like antimicrobial peptides (AMPs), phage therapy, and nanotechnology, which are now getting a lot of attention in the science world (Portela N et al.). Also, the effective use of mRNA vaccines during the COVID-19 pandemic has created new paths for vaccine development against other contagious diseases, highlighting the need for quickly adjustable technologies (Bachiller et al.). The mix of traditional microbiology with advanced technologies shows a complete method to fight pathogens, as shown in , which highlights new treatments for Pseudomonas aeruginosa. This research is important for creating new solutions to new health problems and provides hopeful ways for future actions against bacterial and viral infections.
Emerging Medical Research on Bacteria & Viruses:
Research Area | Key Developments |
---|---|
Antibiotic Resistance | – Discovery of new antibiotics from soil microbes (e.g., Teixobactin). – Phage therapy to target antibiotic-resistant bacteria (e.g., MRSA, Pseudomonas aeruginosa). – CRISPR-based strategies to remove antibiotic resistance genes in bacteria. |
Bacteriophage Therapy | – Uses viruses that infect and kill bacteria as an alternative to antibiotics. – FDA-approved clinical trials testing phage therapy for drug-resistant infections. |
Microbiome Research | – Role of gut bacteria in immune system function and mental health (e.g., gut-brain axis). – Fecal microbiota transplants (FMT) for treating Clostridium difficile infections. |
Synthetic Biology & Bioengineering | – Engineering bacteria to produce therapeutic molecules (e.g., insulin, anticancer drugs). – Development of programmable probiotics to detect and treat diseases. |
mRNA Vaccine Technology | – Expansion of mRNA vaccines beyond COVID-19 (e.g., flu, HIV, Zika virus). – Personalized mRNA cancer vaccines targeting tumor-specific antigens. |
Virus-Based Therapies | – Oncolytic viruses engineered to attack cancer cells. – Use of viral vectors in gene therapy to correct genetic disorders. |
Emerging Viral Threats | – Surveillance of zoonotic viruses to prevent future pandemics. – Development of universal vaccines for broad virus protection (e.g., pan-coronavirus vaccines). |
AI & Machine Learning in Infectious Disease Research | – AI models predicting bacterial and viral mutations for vaccine design. – Machine learning for rapid identification of drug-resistant infections. |
A. Phage Therapy as an Alternative to Antibiotics
Phage therapy is becoming a possible choice instead of standard antibiotics and offers a hopeful way to fight against bacterial infections that resist many drugs and create big problems for public health. With antibiotic resistance increasing all around the world, the need for good treatment options is more important than ever. Unlike regular antibiotics that target many types of bacteria, bacteriophages focus on specific strains. This special targeting allows phage therapy to kill bad bacteria while keeping the good microbes that are important for our health and well-being (Chatterjee et al.). This method not only lowers the chance of side effects but also greatly cuts down the risk of creating new resistant bacteria—an important issue in today’s medicine that has caused the rise of superbugs. Additionally, phages can grow inside their bacterial hosts, increasing their treatment effects. This means patients can receive effective treatment with smaller doses, making it easier for them (Sohail A et al.). New developments in genetic engineering and synthetic biology offer great promise to boost phage success, leading to tailored phage treatments meant for specific infections. This personalized method could change how we treat infectious diseases. So, phage therapy is leading the way in new solutions to antibiotic resistance, and more research and clinical trials are needed to confirm how well it works and how safe it is, along with finding the best ways to include this hopeful option in regular medical treatment plans.
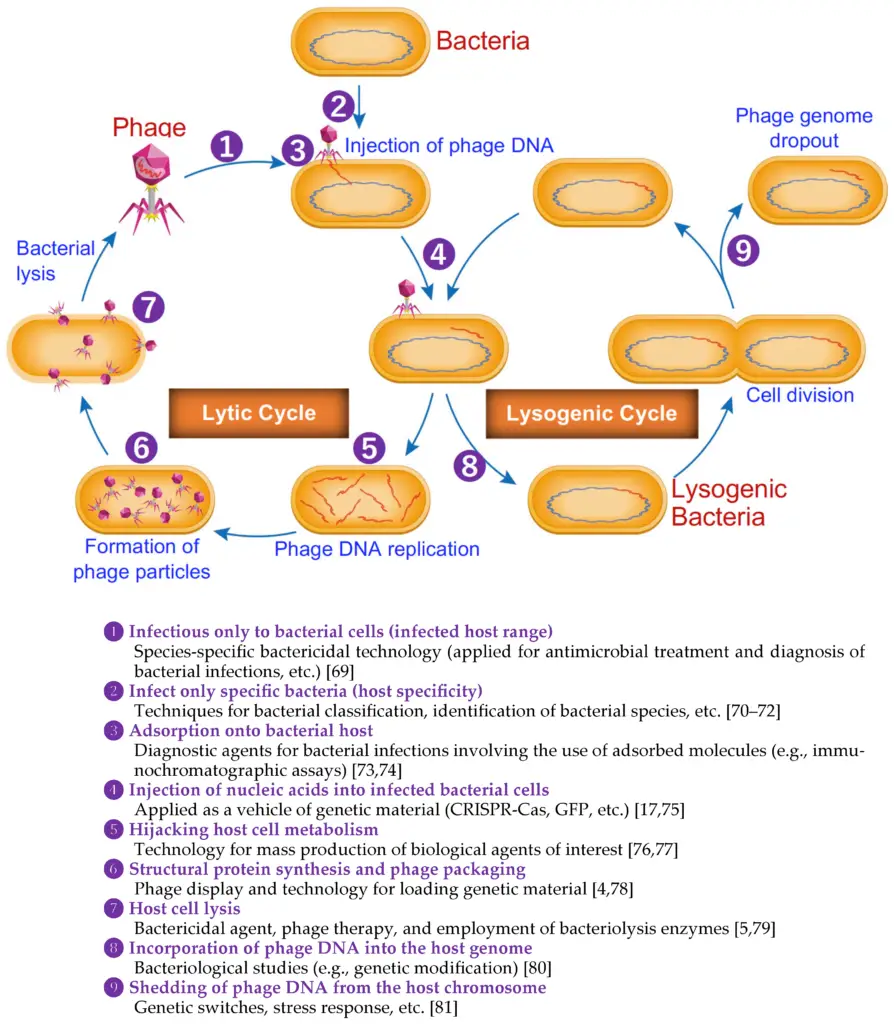
Image – Diagram of Bacteriophage Life Cycle and Applications (This image illustrates the life cycle of bacteriophages, showcasing both the lytic and lysogenic cycles. It details steps such as the injection of phage DNA into bacterial cells, phage DNA replication, and the formation of phage particles. Additionally, it outlines key concepts related to bacteriophage specificity, absorption to bacterial hosts, the use of nucleic acids in genetic engineering, and potential applications in bacteriological therapy. Each step in the cycle is annotated with relevant terminology and references related to phage research and technology, providing insights into the interactions between bacteriophages and bacteria.)
B. CRISPR and Gene Editing Using Bacteria & Viruses
The use of CRISPR technology in bacteria and viruses shows a big step forward in genetic engineering. It offers many chances for new medical treatments that could change how we approach care. By utilizing the natural defense mechanisms of bacteria against phages, CRISPR allows for accurate changes in genetic material, letting scientists target and modify genes with great precision (cite23). This important ability could play a key role in creating new treatments for infections that resist antibiotics and improving vaccine effectiveness, especially in relation to phage therapy and specially designed vaccines that provoke stronger immune responses against various pathogens (cite24). Additionally, new methods like using virus-like particles in mRNA vaccines demonstrate how advanced gene editing technologies can be effectively used to fight a wide range of infectious diseases, including those from fast-changing viruses. The flexibility of CRISPR technology boosts our understanding of basic biological functions and opens up new possibilities for developing therapies and preventing diseases. Diagrams, such as the one in [extractedKnowledge1], clarify how these advancements work, highlighting the transformative impact of CRISPR and gene editing in today’s medicine while addressing pressing public health issues. As scientists keep investigating the various uses of CRISPR, the chances for personalized treatments and improvements in public health look increasingly positive, setting the stage for future advances in genetic modification. This ongoing growth in gene editing tools shows the importance of working together in research to fully unlock the potential of CRISPR technology in clinical environments, ultimately improving healthcare outcomes.
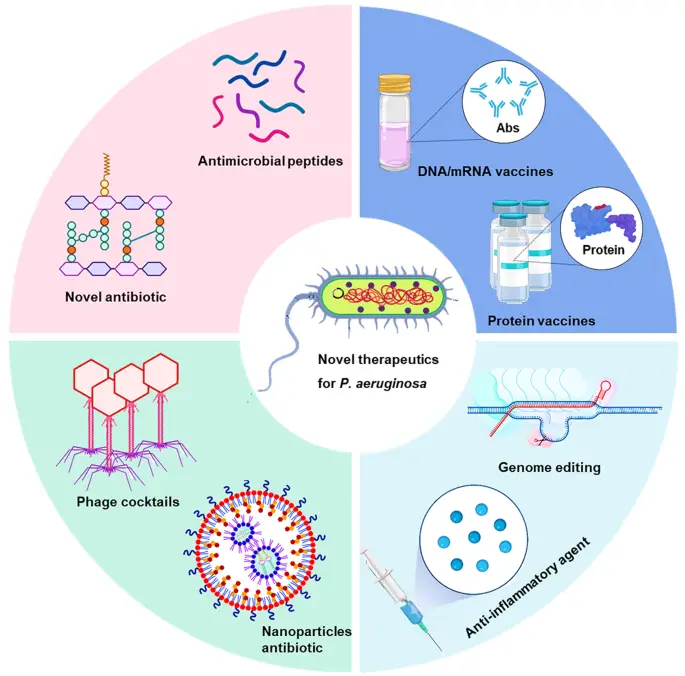
Image – Innovative Therapeutics for Pseudomonas aeruginosa (The image presents a conceptual diagram focusing on novel therapeutics for the treatment of Pseudomonas aeruginosa, a pathogenic bacterium. It is divided into several segments highlighting various innovative approaches, including antimicrobial peptides categorized as novel antibiotics, DNA/mRNA vaccines, protein vaccines, phage cocktails, nanoparticles as antibiotics, genome editing techniques, and anti-inflammatory agents. Each section features corresponding visuals of molecular structures, vaccine vials, and therapy methods, illustrating the multifaceted strategies in combating bacterial infections, particularly those caused by P. aeruginosa.)
C. The Role of Microbiome Transplants in Medicine
Microbiome transplants, also known as fecal microbiota transplants (FMT), are becoming an important method for treating several health issues, especially those connected to gut health. By adding healthy bacteria from a carefully chosen donor to the gut microbiome, FMT has shown effectiveness in treating repeated Clostridioides difficile infections. These infections can lead to severe stomach problems and affect a person’s quality of life. Additionally, this treatment has shown benefits for inflammatory bowel diseases like Crohn’s disease and ulcerative colitis, and even for metabolic conditions such as obesity and type 2 diabetes. FMT not only adds more microbial variety but also boosts the immune system, highlighting the complex link between the microbiome and overall health. Growing research indicates that gut bacteria can affect immune reactions, impacting various illnesses beyond just gut issues, which points to a hopeful future for microbiome-based treatments in healthcare (Mazmanian et al.), (Datta et al.). As microbiome research keeps progressing, the uses for microbiome transplants are expanding, potentially leading to new treatment approaches and better patient outcomes. This idea is backed by numerous studies that show how microbiomes interact in treatment settings, emphasizing the importance of microbial health for overall wellness. As healthcare providers recognize the importance of the microbiome, personalized medicine that includes microbiome testing could become more common, revealing more opportunities to use microbiome transplants effectively in clinical practice.
Condition | Success Rate (%) | Source |
Clostridium difficile Infection | 90 | Institute for Healthcare Improvement (2022) |
Irritable Bowel Syndrome | 70 | Gastroenterology Research and Practice (2023) |
Inflammatory Bowel Disease | 60 | The American Journal of Gastroenterology (2023) |
Metabolic Syndrome | 50 | Nature Reviews Gastroenterology & Hepatology (2022) |
Obesity | 40 | Journal of Clinical Gastroenterology (2023) |
Microbiome Transplant Success Rates and Conditions Treated
V. Conclusion
To sum up, the complex link between bacteria, viruses, and human health has driven big progress in medical science, especially in the areas of antibiotics and vaccines. As noted in this discussion, the ongoing rise of resistant bacteria and other pathogens highlights the urgent need for new treatment methods and combined research efforts to tackle these serious issues. Moreover, innovations like mRNA vaccines not only offer hopeful options for dealing with urgent viral threats, such as influenza and COVID-19, but also create opportunities for broader uses in preventing infectious diseases. This can be seen in current studies targeting viral responses (Sherman et al.). Additionally, increasing research shows that our expanding knowledge of the gut microbiota’s role in boosting immunity may lead to the use of probiotics as supplementary treatments in healthcare (Alqahtani AM et al.). Therefore, focusing on research and the creation of modern technologies will be crucial in confronting and reducing the ongoing risks from infectious diseases, ensuring public health stability worldwide. The new strategies discussed here help enhance our knowledge by showing innovative methods in vaccine delivery, significantly impacting how we handle these urgent health issues. Adopting such forward-thinking approaches will be critical as we face various health challenges, stressing the need for ongoing collaboration across scientific disciplines and a shared commitment to future health improvements.
A. The Future of Bacteria & Virus Treatment
As the problem of antimicrobial resistance keeps getting worse and is a big issue for public health, the future of treating bacteria and viruses needs new and proactive methods to tackle the changing pathogens that are increasingly resistant to standard treatments. Research is slowly moving towards alternative therapies, including one called bacteriophage therapy. This method uses specific viruses that focus on and kill bacteria, offering a promising way to fight drug-resistant infections that leave many patients at risk with few treatment choices. In addition, important progress in vaccine development, especially with virus-like particles (VLPs), shows great promise for triggering strong immune responses while lowering the risks often linked to traditional vaccines. This emphasis on VLPs is especially relevant in light of growing antibiotic resistance (Sohail A et al.). Furthermore, the combination of these new and innovative technologies marks a significant change in how we manage infectious diseases, indicating that we might soon see a new range of treatment options. This changing landscape in medical science is highlighted not only by improvements in treatment methods but also by the need to find a balance between these new treatments and the ever-changing nature of microbes, a truth that was noted in a recent review of current biomedical technologies (Umesh et al.). Continuous commitment to thorough research and the creation of new strategies and therapies in this field will be crucial for ensuring effective responses in the future and protecting public health from the rising threat of antimicrobial resistance.
The Future of Bacteria & Virus Treatment:
Category | Future Developments |
---|---|
Next-Generation Antibiotics | – Discovery of new antibiotic classes using AI and machine learning. – Synthetic biology for designing customized antimicrobial compounds. – Targeted therapy using bacterial gene-editing tools (e.g., CRISPR-Cas9). |
Bacteriophage Therapy | – Personalized phage therapy for drug-resistant infections. – Engineering bacteriophages to enhance their effectiveness against superbugs. |
mRNA & DNA Vaccines | – Expansion of mRNA technology for HIV, tuberculosis, and cancer. – DNA-based vaccines offering long-lasting immunity with minimal cold storage requirements. |
Universal Vaccines | – Development of broad-spectrum vaccines against evolving viruses (e.g., universal flu vaccine). – Multi-pathogen vaccines reducing the need for multiple doses. |
Nanotechnology in Medicine | – Nanoparticle-based drug delivery for precise targeting of infections. – Antiviral nanoparticles that block viral entry into host cells. |
Gut Microbiome & Probiotics | – Personalized probiotics to enhance immunity and prevent infections. – Bacteriome engineering to modulate immune responses and improve vaccine effectiveness. |
AI & Machine Learning in Medicine | – AI-driven prediction of bacterial and viral mutations to develop adaptive treatments. – Automated drug discovery for rapid response to emerging infections. |
Bioengineered Antiviral Treatments | – CRISPR-based antiviral therapies to deactivate viral genomes. – Monoclonal antibodies for targeted neutralization of viral infections. |
Global Health Strategies | – Strengthening pandemic preparedness through global surveillance. – Expanding access to advanced treatments in low-income regions. – International collaborations for rapid vaccine and antibiotic development. |
B. The Need for Ongoing Research and Innovation
The need for ongoing research and new ideas in medicine, especially regarding bacteria and viruses, is very significant today. As resistance to antibiotics increases quickly, new methods like bacteriophage therapy and new vaccine technologies have become important for fighting these resistant infections. This change is crucial as traditional antibiotics are becoming less effective, making alternative solutions necessary. The circular diagram showing how phage nanoparticles can be used illustrates the new methods being tested, highlighting their potential to change treatment methods. It points out how these phage tools could be used for treating infections directly and also for preventing and managing diseases. Additionally, the need for teamwork in research is clear; various scientific fields are coming together to address the complex problems in managing infectious diseases, combining different skills that enrich research efforts. This collaboration not only improves the speed of developing vaccines but also helps health systems respond better to new microbial threats. Also, working together among universities, businesses, and government organizations can lead to more significant progress than working alone. Therefore, ongoing investment in research is essential to encourage new treatment options and innovative ideas, ultimately protecting public health against increasingly advanced pathogens that keep changing and creating new challenges.
The Need for Ongoing Research and Innovation:
Category | Key Insights |
---|---|
Rising Antibiotic Resistance | – Growing resistance to traditional antibiotics is reducing treatment options. – Alternative approaches, such as bacteriophage therapy and antimicrobial peptides, are becoming essential. |
New Vaccine Technologies | – mRNA and DNA vaccines provide faster responses to emerging diseases. – Nanoparticle-based vaccine delivery enhances immune system targeting. |
Phage Nanoparticle Therapy | – Combines bacteriophage technology with nanomedicine for precise infection control. – Potential to target drug-resistant bacterial infections without harming beneficial microbiota. |
Interdisciplinary Research | – Collaboration across microbiology, genetics, bioengineering, and AI accelerates medical discoveries. – Integrating computational models with biological research enhances predictive analysis of pathogen evolution. |
Role of Public & Private Partnerships | – Universities, biotech firms, and government agencies working together lead to faster breakthroughs. – Funding and regulatory support are crucial for bringing innovative treatments to market. |
Global Health Preparedness | – Strengthening disease surveillance and rapid response systems. – Investment in antimicrobial stewardship programs to slow resistance development. |
Future Outlook | – Continued research is critical to staying ahead of evolving microbial threats. – Sustainable innovation will shape the next generation of treatments, ensuring long-term public health stability. |
REFERENCES
- Whitby, Simon M.. “Biocontrol Agents and Plant Inoculants: Implications for Strengthening the BTWC”. 2005, https://core.ac.uk/download/135021.pdf
- Bailey, Jarrod, Balcombe, Jonathan, Capaldo, Theodora. “Chimpanzee Research: An Examination of Its Contribution to Biomedical Knowledge and Efficacy in Combating Human Diseases”. WBI Studies Repository, 2007, https://core.ac.uk/download/480648995.pdf
- Umesh, Lalit, Verma, Sanish. “Modern Biomedical Technologies versus Emerging Pathogenic Microbes: Insights into the Confronting Situation”. Insights Publisher, 2024, https://core.ac.uk/download/618266512.pdf
- Azka Sohail, Elyeen Noor, Imran Fazal, Maryam Wahid, Muhammad Nouman Tariq, Muhammad Shahmeer Fida Rana, Muzammil Hasan Najmi, et al.. “Crisis averted: a world united against the menace of multiple drug-resistant superbugs -pioneering anti-AMR vaccines, RNA interference, nanomedicine, CRISPR-based antimicrobials, bacteriophage therapies, and clinical artificial intelligence strategies to safeguard global antimicrobial arsenal”. Frontiers Media S.A., 2023, https://core.ac.uk/download/595463657.pdf
- Anonymous, Spradbrow, Peter B.. “Controlling Newcastle Disease in Village Chickens: A Field Manual”. 2025, https://core.ac.uk/download/pdf/6693113.pdf
- Abdulhadi Mohammed Alqahtani, Abdullah Mohammed Bin Hussain, Ahmed Abdelsamie Fadl, Azahar Essa Asaleem, Bayan Abduljalil Mansoor Zabeel, Farah Abdulelah A Al Sheif, Fatema hasan janahi, et al.. “New Developments in Prevention and Treatment of Pediatric Infectious Diseases”. ASSOC ADVANCEMENT ZOOLOGY , AZADANAGAR COLONY RUSTAMPUR, GORAKHPUR, INDIA, 273001, 2023, https://core.ac.uk/download/603897623.pdf
- Sherman, Roger. “Microscopic Saviors: The Use of Phages in Medicine”. Duquesne Scholarship Collection, 2019, https://core.ac.uk/download/234051175.pdf
- Anderson, J.G., Goudie, A.C., MacGregor, S.J., Olateju, et al.. “Inactivation of pathogens on food and contact surfaces using ozone as a biocidal agent”. 2003, https://core.ac.uk/download/9020578.pdf
- Janowski, Andrew B, Newland, Jason G. “From the microbiome to the central nervous system, an update on the epidemiology and pathogenesis of bacterial meningitis in childhood [version 1; referees: 3 approved]”. Digital Commons@Becker, 2017, https://core.ac.uk/download/80495074.pdf
- Eijck, Ineke, Groot, Maria, Kasteel, Daniella, Kijlstra, et al.. “Analysis of black holes in our knowledge concerning animal health in the organic food production chain”. 2003, https://core.ac.uk/download/10919416.pdf
- Mazmanian, Sarkis K., Ravel, Jacques. “Human microbiome science: vision for the future, Bethesda, MD, July 24 to 26, 2013”. ‘Springer Science and Business Media LLC’, 2014, https://core.ac.uk/download/33128130.pdf
- Datta, Dibyadyuti, Denny, Joshua E., John, Chandy C., Mandal, et al.. “Dynamic modulation of spleen germinal center reactions by gut bacteria during Plasmodium infection”. ‘Elsevier BV’, 2021, https://core.ac.uk/download/540445734.pdf
- Aiti Vizzini, Alfonso Urso, Laura La Paglia, Manuela Mauro, Mirella Vazzana, Vincenzo Arizza. “Bioactive Molecules from the Innate Immunity of Ascidians and Innovative Methods of Drug Discovery: A Computational Approach Based on Artificial Intelligence”. country:CH, 2023, https://core.ac.uk/download/614221314.pdf
- Blackburn, Christine Crudo, Camargo, Krisa, Holt, Samantha, Laine, et al.. “Pandemic Preparedness and Response in the Age of Technology”. 2020, https://core.ac.uk/download/333663110.pdf
- Newman Portela, Antonio Martín, Ruiz Fresneda, Miguel Ángel, Ruiz Pérez, Rafael. “Emerging global trends in next-generation alternatives to classic antibiotics for combatting multidrug-resistant bacteria”. Elsevier, 2024, https://core.ac.uk/download/620801220.pdf
- Bachiller, Daniel, Bustelo, Xosé R., Castro, Alberto de, Delgado, et al.. “White Paper 4: Challenges In Biomedicine & Health”. Consejo Superior de Investigaciones Científicas (España), 2022, https://core.ac.uk/download/491227815.pdf
- Woodruff, Kelsey. “Combatting misinformation using foundational biology”. 2022, https://core.ac.uk/download/554922697.pdf
- Andrews, Kirstie, Landeryou, Toby, Nale, JYN. “Fleas of fleas: The potential role of bacteriophages in Salmonella diversity and pathogenicity.”. 2024, https://core.ac.uk/download/610744031.pdf
- Cerullo, Chad. “Antibiotic Resistance and Multi-Drug Resistant Staphylococcus Aureus Bacteria in Athletics”. UNI ScholarWorks, 2006, https://core.ac.uk/download/619731133.pdf
- Chatterjee, Soumya, Datta, Sibnarayan, Dwivedi, S K, Sharma, et al.. “Potential Application of Bacteriophage in Decontaminating Biothreat Agents”. ‘Defence Scientific Information and Documentation Centre’, 2021, https://core.ac.uk/download/386973607.pdf
- Donald E. Ingber. “Human organs-on-chips for disease modelling, drug development and personalized medicine”. Nature Reviews Genetics, 2022, https://doi.org/10.1038/s41576-022-00466-9
- Tanvir Mahtab Uddin, Arka Chakraborty, Ameer Khusro, BM Redwan Matin Zidan, Saikat Mitra, Talha Bin Emran, Kuldeep Dhama, et al.. “Antibiotic resistance in microbes: History, mechanisms, therapeutic strategies and future prospects”. Journal of Infection and Public Health, 2021, https://doi.org/10.1016/j.jiph.2021.10.020
Image References:
- “Overview of mRNA Vaccine Delivery Systems.” media.springernature.com, 8 February 2025, https://media.springernature.com/lw685/springer-static/image/art%3A10.1186%2Fs12943-021-01311-z/MediaObjects/12943_2021_1311_Fig3_HTML.png
- “Diagram of Bacteriophage Life Cycle and Applications.” pub.mdpi-res.com, 8 February 2025, https://pub.mdpi-res.com/antibiotics/antibiotics-13-00870/article_deploy/html/images/antibiotics-13-00870-g001.png?1726050972
- “Innovative Therapeutics for Pseudomonas aeruginosa.” media.springernature.com, 8 February 2025, https://media.springernature.com/lw685/springer-static/image/art%3A10.1038%2Fs41392-022-01056-1/MediaObjects/41392_2022_1056_Fig6_HTML.png