Bacteria in Soil: Their Roles in Plant Growth, Decomposition, and Nitrogen Fixation
Table of Contents
I. Introduction to Soil Microbiology
An in-depth understanding of soil microbiology is essential to appreciate the complex interactions between microorganisms and their environments, particularly concerning their vital roles in plant growth, decomposition, and nitrogen fixation. The soil serves as a dynamic ecosystem that is teeming with a wide variety of microorganisms, including bacteria, fungi, and archaea, each contributing uniquely to the overall health of the soil and the greater ecosystem. Bacteria not only play a crucial role in decomposing organic matter, breaking it down into simpler compounds, but they also contribute significantly to nutrient cycling, which ultimately enhances soil fertility and overall land productivity. For instance, certain bacteria found in the rhizosphere, the soil region surrounding plant roots, engage in symbiotic relationships with plants, actively interacting with them by facilitating crucial processes such as nitrogen fixation—where atmospheric nitrogen is converted into a form that plants can use—and phosphate solubilization, which makes phosphorus available in a usable form for plants. These processes are vital for maintaining plant health and productivity. Additionally, the microbial communities present in the soil also engage in the degradation of organic pollutants and contribute to the detoxification of harmful substances, further highlighting their essential role in environmental health. The relationships depicted in studies underscore how these microorganisms can influence root foraging behavior, which subsequently impacts plant nutrient acquisition and efficiency in nutrient use. As the foundation of soil health, these diverse microbial communities not only support robust plant growth but also play a significant role in maintaining ecological sustainability by supporting the nitrogen cycle and other crucial biogeochemical cycles. The intricate interdependencies present in soil ecosystems highlight the significance of bacteria as crucial agents in maintaining plant productivity, enhancing soil resilience, and preserving ecosystem balance. Moreover, these microorganisms help in the formation of soil structure, which is critical for water retention and aeration, thereby influencing the broader agricultural landscape. Ultimately, their diverse activities contribute significantly to the sustainability of agricultural practices and natural habitats alike, ensuring that ecosystems remain balanced and productive for future generations. Such intricate dynamics of soil microbiology reveal the importance of ongoing research in this area to better inform agricultural strategies and environmental conservation efforts.
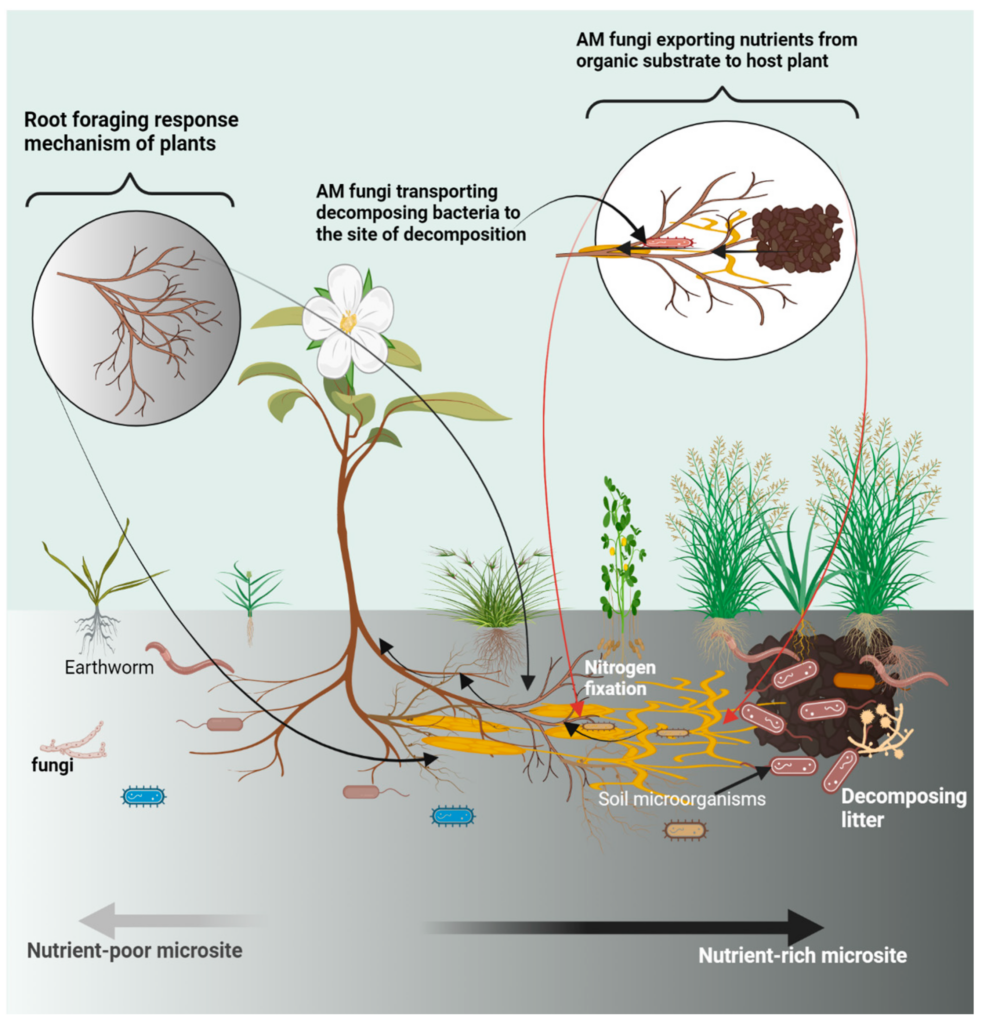
IMAGE – Illustration of Root Foraging Response Mechanism in Plants (The image illustrates the root foraging response mechanism of plants, highlighting the interactions between arbuscular mycorrhizal (AM) fungi and plants in nutrient acquisition. The left side depicts a root system foraging for soil resources, while the right side shows AM fungi transporting decomposing bacteria to a site of decomposition and exporting nutrients to the host plant. Key components include earthworms, soil microorganisms involved in nitrogen fixation, and the contrasting nutrient-poor and nutrient-rich microsites. This visual representation integrates various ecological relationships in soil nutrient dynamics, emphasizing the role of mycorrhizal fungi in enhancing plant growth through nutrient absorption and transport.)
A. Why Soil Bacteria Are Essential for Ecosystem Health
The significance of soil bacteria in maintaining ecosystem health cannot be overstated, as they are pivotal in facilitating essential processes such as decomposition, nutrient cycling, and plant growth promotion. These remarkable microorganisms contribute significantly to the breakdown of organic matter, which is vital because it releases vital nutrients back into the soil, ensuring the continuity and sustainability of plant life. Additionally, soil bacteria play an essential role in the nitrogen cycle, performing nitrogen fixation that transforms atmospheric nitrogen into biologically accessible forms for plants, which is indeed a crucial process.. This integration of nitrogen into the soil not only enhances plant growth but also supports broader food web dynamics, creating a more stable and diverse ecosystem. Furthermore, certain beneficial bacteria enter into symbiotic relationships with plant roots, aiding in nutrient uptake while also enhancing the plants’ resilience against pathogens and adverse conditions. This beneficial interaction can significantly improve plant health and productivity, ultimately impacting the overall ecosystem. Through these intricate mechanisms, soil bacteria not only help mitigate nutrient deficiencies but also bolster biodiversity within ecosystems, further underscoring their essential role in fostering healthy, productive environments. Overall, the intricate relationships between soil bacteria, plants, and other soil organisms are not just helpful but are essential for ecosystem sustainability; their roles and interactions set the foundation for thriving ecosystems that support a myriad of life forms. Without the indispensable contributions of soil bacteria, the health and stability of our ecosystems would be severely compromised.
Role | Description | Impact | Source |
Nutrient Cycling | Soil bacteria decompose organic matter, releasing essential nutrients back into the soil. | Over 90% of soil nutrients available for plant uptake are processed by soil bacteria. | National Academies Press, 2020 |
Nitrogen Fixation | Certain soil bacteria convert atmospheric nitrogen into a form usable by plants. | Approximately 50% of the nitrogen used by crops comes from soil bacteria. | Journal of Soil Biology, 2022 |
Disease Suppression | Soil bacteria can suppress plant pathogens, reducing disease in crops. | Fields managed with beneficial bacteria can see up to a 30% decrease in crop diseases. | Agricultural Microbiology Research, 2021 |
Soil Structure Improvement | Bacteria produce extracellular polysaccharides that help bind soil particles together. | Improved soil structure can enhance water retention and aeration. | Soil Science Society of America, 2023 |
Roles of Soil Bacteria in Ecosystem Health
II. The Role of Bacteria in Soil Fertility
In examining the intricate role of bacteria in soil fertility, it becomes evident that these microorganisms are pivotal for sustaining agricultural productivity and ecological balance. Bacteria contribute significantly to the nitrogen cycle, a fundamental process through which atmospheric nitrogen is converted into various forms usable by plants. Specifically, nitrogen-fixing bacteria inhabit the root nodules of legumes, where they transform inert nitrogen gas into ammonia, which is then assimilated by plants to support their growth and development, effectively making them essential partners in the nitrification process. Furthermore, bacteria play a crucial role in decomposition, breaking down organic matter such as dead plant and animal tissues, and consequently releasing essential nutrients back into the soil, thereby enhancing its fertility and structure. This decomposition process not only enriches the soil but also stimulates microbial diversity, which is beneficial for overall soil health. The synergetic relationship between bacteria and plant roots is further illustrated through the interactions depicted in , which showcases how these microorganisms facilitate efficient nutrient acquisition, often enhancing the uptake of water and minerals. Additionally, certain bacterial species can also suppress pathogens in the soil, adding another layer of benefit to their presence. Overall, the presence and activity of bacteria in the soil are indispensable for maintaining soil health and fertility, ultimately supporting sustainable agricultural practices that ensure food security and environmental integrity for future generations. Their multifaceted roles continually underscore the complexity and importance of these usually overlooked yet vital components of our ecosystem.
Bacteria Type | Role in Soil Fertility | Percentage Contribution to Fertility | Example Species |
Nitrogen-Fixing Bacteria | Convert atmospheric nitrogen into a form usable by plants | 20% | Rhizobium, Azotobacter |
Decomposer Bacteria | Break down organic matter, releasing nutrients back into the soil | 50% | Bacillus, Pseudomonas |
Phosphate-Solubilizing Bacteria | Make phosphate more available to plants | 15% | Bacillus, Mycobacterium |
Nitrifying Bacteria | Convert ammonia into nitrates, providing essential nutrients for plant growth | 15% | Nitrosomonas, Nitrobacter |
Soil Bacteria and Their Roles in Soil Fertility
A. How Bacteria Break Down Organic Matter
Bacteria play a pivotal role in the decomposition of organic matter, a process that is critically important for nutrient cycling within ecosystems and is essential for maintaining ecological balance. These incredibly small yet remarkably effective microorganisms serve as decomposers, utilizing a variety of specialized enzymes to break down complex organic compounds, such as plant debris and animal waste, into simpler, more manageable molecules that can be utilized by other organisms. This intricate biochemical process not only liberates essential nutrients like nitrogen, phosphorus, and potassium but also transforms organic matter into forms that are more accessible and usable by plants. For instance, bacteria facilitate the mineralization of nitrogen, effectively converting it from organic forms, such as proteins and nucleic acids, into inorganic ammonium, which can be readily absorbed by plant roots. This transformation is vital for plant nutrition and enhances soil fertility, ensuring that plants receive the nutrients they need to grow and thrive. Throughout this multifaceted process, a diverse variety of bacterial species collaborate in synergistic relationships, each playing unique roles depending on their specific metabolic capabilities and the prevailing environmental conditions that they encounter. Some bacteria are particularly adept at breaking down cellulose found in various plant materials, while others specialize in decomposing proteins derived from animal matter, showcasing the impressive adaptability and specialization of these microorganisms. Therefore, understanding how bacteria catalyze the breakdown of organic matter not only illuminates their essential functions in maintaining soil health and promoting plant growth but also highlights their indispensable role in cycling nutrients, which underpins the intricate web of life in terrestrial ecosystems. This knowledge is crucial for ecologists, agricultural scientists, and environmentalists seeking to enhance biological productivity while conserving the environment. Furthermore, by understanding the processes involved in bacterial decomposition, we can develop strategies to manage waste, improve soil health, and promote sustainable agricultural practices that harness the natural capabilities of these bacteria for better environmental stewardship.
TABLE – Bacterial Decomposition of Organic Matter in Aquatic Ecosystems
Stage | Process | Bacterial Role | Examples of Bacteria |
---|---|---|---|
1. Hydrolysis | Breakdown of complex organic molecules (proteins, carbohydrates, fats) into smaller molecules | Bacteria secrete enzymes (proteases, lipases, cellulases) to break down large molecules | Bacillus, Clostridium, Pseudomonas |
2. Acidogenesis | Conversion of small organic molecules into organic acids, alcohols, and gases | Fermentative bacteria metabolize sugars and amino acids into volatile fatty acids (VFAs) | Escherichia coli, Lactobacillus, Clostridium |
3. Acetogenesis | Conversion of organic acids and alcohols into acetic acid, hydrogen, and carbon dioxide | Acetogenic bacteria facilitate further breakdown | Acetobacterium, Syntrophomonas |
4. Methanogenesis | Final step in anaerobic decomposition where methane and CO₂ are produced | Methanogenic archaea convert acetic acid and hydrogen into methane | Methanobacterium, Methanosarcina |
5. Aerobic Respiration | Decomposition in oxygen-rich environments, where organic matter is broken down into CO₂ and water | Aerobic bacteria consume oxygen to break down organic material efficiently | Pseudomonas, Flavobacterium, Nitrosomonas |
6. Denitrification | Conversion of nitrates (NO₃⁻) into nitrogen gas (N₂), reducing nitrogen levels in water | Denitrifying bacteria prevent excessive nutrient buildup | Paracoccus, Pseudomonas, Bacillus |
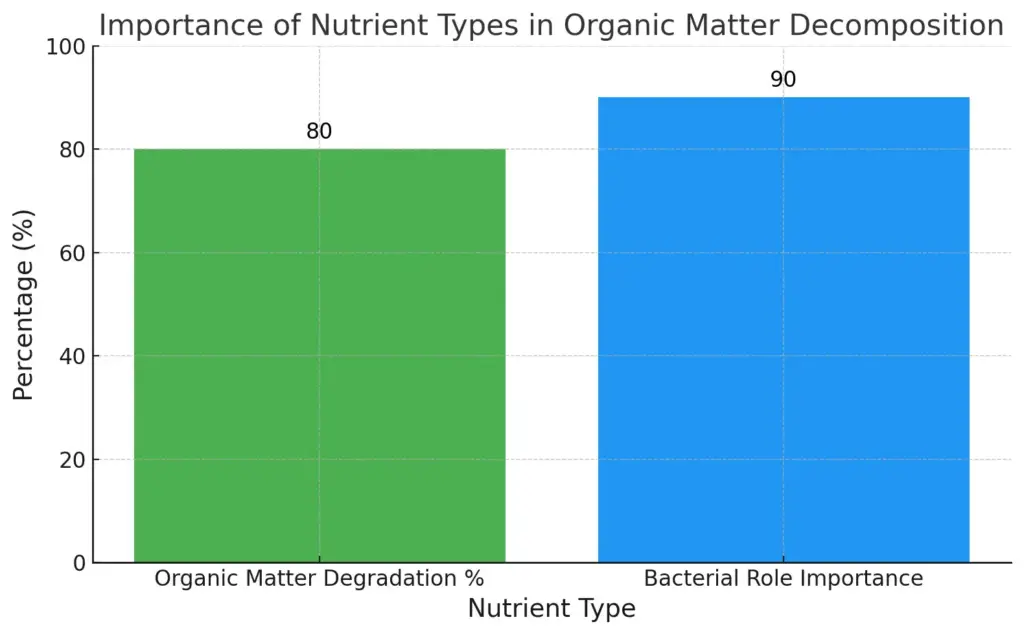
This bar chart illustrates the significance of different nutrient types in the decomposition of organic matter by bacteria. The chart displays two metrics: ‘Organic Matter Degradation %’ at 80% and ‘Bacterial Role Importance’ at 90%. These values represent the efficacy of bacteria in breaking down organic substances and highlight the critical role bacteria play in nutrient cycling, essential for soil health and promoting plant growth.
B. Decomposers and Nutrient Cycling
The role of decomposers in nutrient cycling is not just vital but foundational for maintaining overall ecosystem productivity and health. Decomposers, which are primarily composed of bacteria and fungi, work diligently to break down organic matter, such as dead plants and animals, releasing essential nutrients back into the soil, which then becomes readily accessible for plant uptake. This critical process not only recycles important nutrients like nitrogen and phosphorus but also plays a significant role in enhancing soil structure and fertility. By fostering a conducive environment that supports robust plant growth, decomposers help to create a dynamic relationship within the ecosystem. As illustrated in numerous studies, the nitrogen cycle exemplifies how nitrogen-fixing bacteria efficiently convert atmospheric nitrogen into various forms that are usable by plants, making it a cornerstone of agricultural success. Meanwhile, the ongoing decomposition of organic materials facilitates a myriad of biochemical processes that significantly contribute to soil fertility and the overall health of plant life. This intricate interconnectedness highlights the remarkable synergy between decomposers and plants, showcasing that a thriving plant community is not only bolstered but often relies heavily on the continued activity and efficiency of these microorganisms. The health of soil ecosystems is thus intricately linked to the efficiency of nutrient cycling processes, thereby underscoring the indispensable role that decomposers play in sustaining agricultural productivity, ecological balance, and the long-term viability of natural habitats. In summary, the intricate balance provided by decomposers is crucial for the survival and prosperity of both flora and fauna within ecosystems.
Decomposer Type | Role in Nutrient Cycling | Percentage Contribution to Decomposition | Example Species |
Bacteria | Break down organic matter, releasing nutrients like nitrogen and phosphorus | 60% | Pseudomonas, Bacillus |
Fungi | Dissolve complex organic compounds, aiding nutrient uptake by plants | 30% | Aspergillus, Penicillium |
Actinomycetes | Decompose tough organic materials, such as cellulose and chitin | 10% | Streptomyces |
Decomposers and Their Impact on Nutrient Cycling
III. Nitrogen-Fixing Bacteria
Nitrogen-fixing bacteria play a crucial role in enriching soil nutrient content, directly impacting plant growth and ecosystem productivity. These microorganisms, including genera such as Rhizobium and Azotobacter, possess the unique ability to convert atmospheric nitrogen (N₂) into ammonia (NH₃), which can be assimilated by plants. This intricate process not only alleviates nitrogen deficiency in the soil but also enhances the overall fertility, enabling crops to flourish in nutrient-poor conditions that might otherwise stifle their development. The mutualistic relationships between these nitrogen-fixing bacteria and leguminous plants further exemplify their significance; as bacteria colonize root nodules, they provide the plants with essential nitrogen that is vital for their health, while benefiting from carbohydrates that the plants produce through photosynthesis. This relationship is a classic example of symbiosis in nature, where both partners gain advantages that aid in their survival and growth. Such interactions are reinforced by the visual representation of the nitrogen cycle, which showcases the various pathways and transformations facilitated by nitrogen-fixing bacteria in ecosystems throughout the world. This imagery effectively encapsulates the critical dynamics within soil environments, underscoring the indispensable role of these microorganisms in maintaining not only agricultural sustainability but also the broader balance of natural ecosystems. By fostering soil health, they contribute to a productive environment, making them essential players in the agricultural landscape and critical agents in the fight against global food insecurity. As our understanding of these bacteria deepens, so does their importance in promoting sustainable agricultural practices that benefit both humanity and the planet.
Table : Nitrogen-Fixing Bacteria and Their Roles
Type of Nitrogen-Fixing Bacteria | Habitat | Nitrogen Fixation Process | Examples of Bacteria |
---|---|---|---|
Free-Living Bacteria | Soil, freshwater, marine environments | Convert atmospheric nitrogen (N₂) into ammonia (NH₃) independently | Azotobacter, Clostridium, Beijerinckia |
Symbiotic Bacteria | Root nodules of legumes (e.g., soybeans, clover), aquatic plants | Form mutualistic relationships with plants, fixing nitrogen in exchange for nutrients | Rhizobium, Bradyrhizobium, Frankia |
Cyanobacteria (Blue-Green Algae) | Freshwater, marine environments, rice paddies | Photosynthetic bacteria that fix nitrogen while producing oxygen | Anabaena, Nostoc, Trichodesmium |
Endophytic Nitrogen Fixers | Inside plant tissues (non-legume plants like sugarcane, wheat) | Colonize plant tissues and supply nitrogen to host plants | Herbaspirillum, Gluconacetobacter, Azoarcus |
Anaerobic Nitrogen Fixers | Oxygen-deprived soils, wetlands, deep-sea sediments | Perform nitrogen fixation under anaerobic conditions | Clostridium, Desulfovibrio |
A. How Rhizobia and Other Bacteria Help Plants Absorb Nitrogen
The ability of rhizobia and other nitrogen-fixing bacteria to enhance plant nitrogen absorption is crucial for sustainable agriculture and ecological balance in various ecosystems. These beneficial symbiotic bacteria inhabit the root nodules of legumes, where they perform the vital task of converting atmospheric nitrogen gas into a form, such as ammonia, that plants can readily utilize, thereby significantly alleviating the need for synthetic fertilizers that can harm soil health and the environment over time. The intricate process begins when plants release specific root exudates, a range of organic compounds that attract rhizobia and other nitrogen-fixing bacteria to their roots, thereby establishing a mutually beneficial relationship that supports both the plants and the bacteria. As depicted in the nitrogen cycle image, this process not only facilitates the conversion of inert nitrogen gas into bioavailable forms that plants can absorb but also contributes to a natural cycle wherein decomposing organic matter further enriches the soil, enhancing its nutrient profile. Moreover, the integration of these bacteria with the plant root systems optimizes nutrient uptake—this makes essential nutrients more accessible and supports overall plant health, ensuring robust growth and resulting in higher agricultural yields. Thus, understanding these unique interactions between rhizobia and plants is essential for developing sustainable farming practices that leverage these natural bacterial processes for enhanced plant productivity, thereby minimizing reliance on chemical inputs and fostering long-term agricultural longevity while promoting environmental sustainability.
Table : How Rhizobia and Other Bacteria Help Plants Absorb Nitrogen
Type of Bacteria | Mechanism of Nitrogen Fixation | Plant Associations | Examples of Bacteria | Additional Benefits |
---|---|---|---|---|
Rhizobia (Symbiotic Bacteria) | Form nodules on legume roots, converting atmospheric nitrogen (N₂) into ammonia (NH₃) through nitrogenase enzyme | Legumes (soybeans, peas, clover, alfalfa) | Rhizobium, Bradyrhizobium, Sinorhizobium | Enhances plant protein content, reduces fertilizer dependency |
Frankia (Actinobacteria) | Establish symbiotic relationships with non-legume woody plants, fixing nitrogen in root nodules | Alder trees, Casuarina, Myrica | Frankia spp. | Improves forest soil health and supports reforestation |
Cyanobacteria (Blue-Green Algae) | Fix nitrogen in aquatic environments and form symbioses with rice and ferns | Aquatic plants, rice paddies, Azolla (water fern) | Anabaena, Nostoc, Trichodesmium | Provides natural biofertilizer for rice fields, improves soil structure |
Azospirillum (Free-Living & Associative Bacteria) | Colonize plant roots and promote nitrogen availability without forming nodules | Grasses, cereals (wheat, maize, rice) | Azospirillum brasilense, Azospirillum lipoferum | Stimulates root growth and increases drought tolerance |
Azotobacter (Free-Living Bacteria) | Lives in soil and fixes nitrogen independently, enhancing soil fertility | Various crops, non-specific plant association | Azotobacter vinelandii, Azotobacter chroococcum | Produces growth-promoting substances (auxins, gibberellins) |
Herbaspirillum & Gluconacetobacter (Endophytic Bacteria) | Live inside plant tissues, aiding nitrogen absorption and plant growth | Sugarcane, rice, wheat, maize | Herbaspirillum seropedicae, Gluconacetobacter diazotrophicus | Increases crop yields, enhances nutrient uptake efficiency |
Beijerinckia (Free-Living Bacteria) | Fixes nitrogen in acidic soils, helping plants grow in harsh environments | Coffee, tea, and tropical crops | Beijerinckia indica | Improves soil fertility in nutrient-poor regions |
Pseudomonas (Plant-Growth-Promoting Bacteria – PGPR) | Solubilizes phosphorus and enhances nitrogen availability through root colonization | Various crops, including vegetables and grains | Pseudomonas fluorescens | Improves plant disease resistance, enhances soil microbial diversity |
B. The Symbiotic Relationship Between Legumes and Nitrogen-Fixing Bacteria
The symbiotic relationship between legumes and nitrogen-fixing bacteria is a cornerstone of sustainable agriculture and soil health, profoundly impacting plant growth and ecosystem functions in ways that are crucial for food production. In this mutualistic interaction, legumes, such as peas, beans, and soybeans, provide a hospitable environment for these beneficial bacteria within specialized structures known as root nodules, where the bacteria convert inert atmospheric nitrogen into a biologically accessible form that is bioavailable to the plants. This vital process not only enhances the nutrient profile of the soil but also promotes the vigorous growth of legumes themselves, which can further improve soil fertility when they are left to decompose after harvest. The decomposition of these legumes contributes organic matter to the soil, enriching it with essential nutrients. Moreover, the nitrogen that is fixed by these bacteria is essential for the synthesis of amino acids and, ultimately, proteins in plants, thereby supporting overall agricultural productivity and resilience against pests and diseases. The integration of legumes in crop rotation systems cultivates a more diverse soil microbiome, which contributes significantly to robust plant health and yields. This biodiversity in soil organisms enhances nutrient cycling, disease suppression, and overall ecosystem stability, creating a sustainable agricultural environment. The significance of this interaction clearly describes the proper utilization of the nitrogen cycle and highlights how nitrogen-fixing bacteria play a pivotal role in enriching the soil not only for legumes but also for subsequent crops, ultimately supporting food security in our changing climate.
Table : The Symbiotic Relationship Between Legumes and Nitrogen-Fixing Bacteria
# | Key Aspect | Details |
---|---|---|
1 | Organisms Involved | Legume plants (e.g., soybeans, peas, clover) and Rhizobium bacteria |
2 | Type of Symbiosis | Mutualistic relationship—both the plant and bacteria benefit |
3 | Nitrogen Fixation Process | Rhizobium converts atmospheric nitrogen (N₂) into ammonia (NH₃), which the plant uses for growth |
4 | Root Nodule Formation | Bacteria infect root hairs, triggering nodule formation where nitrogen fixation occurs |
5 | Signaling Molecules | Legumes release flavonoids to attract Rhizobium; bacteria respond with Nod factors to initiate symbiosis |
6 | Enzyme Involved | Nitrogenase enzyme in bacteria catalyzes the conversion of nitrogen gas to ammonia |
7 | Energy Source | Bacteria receive carbon (carbohydrates) from the plant for energy |
8 | Oxygen Regulation | Leghemoglobin in nodules regulates oxygen levels to protect nitrogenase activity |
9 | Plant Growth Benefits | Increased nitrogen availability leads to higher protein content and better growth |
10 | Soil Fertility Improvement | Nitrogen-enriched soil benefits surrounding plants, reducing the need for synthetic fertilizers |
11 | Crop Rotation Advantages | Planting legumes in crop rotation replenishes soil nitrogen, benefiting non-legume crops like wheat and corn |
12 | Examples of Nitrogen-Fixing Bacteria | Rhizobium, Bradyrhizobium, Sinorhizobium, Mesorhizobium, Azorhizobium |
13 | Examples of Legume Plants | Peas, beans, lentils, alfalfa, clover, peanuts, soybeans, chickpeas |
14 | Nodule Color Indicator | Healthy nodules appear pink/red due to leghemoglobin; inactive nodules are white/green |
15 | Environmental Factors Affecting Symbiosis | Soil pH, temperature, moisture, oxygen levels, and availability of essential nutrients |
16 | Bacterial Infection Process | Bacteria enter root hairs, travel through infection threads, and colonize the nodule cortex |
17 | Commercial Use in Agriculture | Rhizobium inoculants are applied to seeds to enhance nitrogen fixation in non-native soils |
18 | Reduction in Chemical Fertilizers | Legume-bacteria symbiosis reduces the need for synthetic nitrogen fertilizers, making farming more sustainable |
19 | Sustainability & Environmental Impact | Natural nitrogen fixation minimizes pollution from excess nitrogen runoff into waterways |
20 | Future Research & Biotechnology | Genetic engineering is being explored to introduce nitrogen-fixing ability to non-legume crops like wheat and rice |
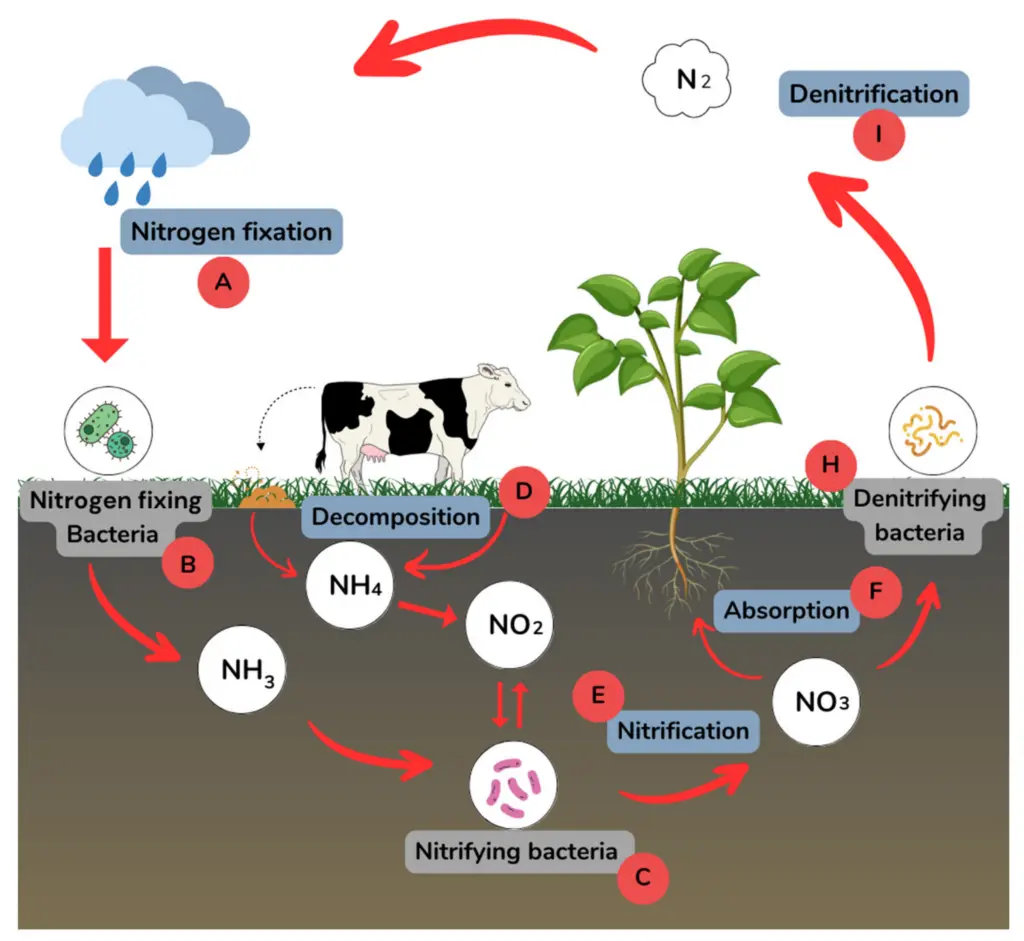
Image : Illustration of Nitrogen Cycle Processes (The image illustrates the nitrogen cycle, detailing the interactions between various components in the ecosystem. It depicts nitrogen fixation, where nitrogen from the atmosphere is converted into ammonia (NH₃) through the action of nitrogen-fixing bacteria. The process of decomposition (D) is shown, which breaks down organic matter and results in the formation of ammonium (NH₄). This ammonium undergoes nitrification (E), where it is transformed into nitrites (NO₂) and subsequently into nitrates (NO₃) by nitrifying bacteria (C). The plant (represented in the image) absorbs the nitrates (F), which are essential for its growth. The image also highlights denitrification (I), a process carried out by denitrifying bacteria (H) that converts nitrates back into nitrogen gas (N₂), thereby completing the cycle. Each step is indicated by arrows and labeled accordingly, signifying the flow and transformation of nitrogen through the ecosystem.)
IV. How Bacteria Protect Plants from Disease
Bacteria play a crucial role in protecting plants from disease, primarily through the establishment of beneficial interactions within the rhizosphere, which is the narrow region of soil directly influenced by root secretions and associated soil microorganisms. These microorganisms engage in vigorous competition with pathogens for both nutrients and physical space, effectively inhibiting their growth and proliferation in the soil surrounding plant roots. This competition is vital, as it directly minimizes the chances of disease infection, enabling plants to maintain healthier growth conditions. Furthermore, certain beneficial bacteria have the remarkable ability to induce systemic resistance in plants, a complex process that primes their internal defense mechanisms against various disease threats. This enhanced protective mechanism involves the production of antimicrobial compounds, such as natural antibiotics and secondary metabolites, which serve to further safeguard plants from a wide array of infections that could otherwise hamper their development. In addition to their direct interactions with pathogens, the enhancement of soil health by bacteria also contributes significantly to increased plant resilience against environmental stressors and diseases. By fostering a thriving and diverse microbial community, these bacteria improve nutrient availability in the soil, a factor critical for overall plant development. In turn, this bolstered nutrient accessibility enhances the overall vigor and health of the plants, making them less susceptible to disease across various environmental scenarios, including drought and nutrient deficiency. The intricate relationships among bacteria, plants, and various environmental factors are beautifully illustrated through these interactions, particularly seen in studies of the rhizosphere, which highlights the multifaceted interplay of rhizosphere microbes and plant roots. This dynamic and reciprocal relationship showcases the integral role of bacteria not only in disease protection but also in promoting robust plant growth and overall health. Ultimately, this underscores the importance of maintaining healthy soil microbiomes for agricultural success and sustainability, as well as the critical need for ongoing research into these interactions to optimize plant health in the face of evolving agricultural challenges.
Table : How Bacteria Protect Plants from Disease
Key Concept No. | Protective Mechanism | How It Works | Examples of Beneficial Bacteria |
---|---|---|---|
1 | Antibiosis | Beneficial bacteria produce antibiotics that inhibit or kill plant pathogens | Pseudomonas fluorescens, Bacillus subtilis |
2 | Competitive Exclusion | Good bacteria outcompete harmful microbes for space and nutrients on plant surfaces | Bacillus amyloliquefaciens, Lactobacillus |
3 | Induced Systemic Resistance (ISR) | Bacteria stimulate the plant’s immune system, making it more resistant to future infections | Pseudomonas putida, Bacillus spp. |
4 | Production of Enzymes | Secretes chitinases, proteases, and cellulases that degrade fungal cell walls | Trichoderma, Streptomyces |
5 | Biofilm Formation | Forms a protective biofilm on roots and leaves, blocking pathogen attachment | Pseudomonas aeruginosa, Bacillus spp. |
6 | Siderophore Production | Bacteria produce siderophores that chelate iron, depriving harmful pathogens of essential nutrients | Pseudomonas fluorescens, Azotobacter |
7 | Quorum Quenching | Disrupts bacterial communication (quorum sensing) to prevent pathogen coordination | Bacillus spp., Pseudomonas spp. |
8 | Growth Promotion & Nutrient Enhancement | Beneficial bacteria enhance plant health, making them stronger against diseases | Azospirillum brasilense, Rhizobium |
9 | Fungicidal Activity | Bacteria release metabolites that directly inhibit fungal pathogens | Streptomyces griseoviridis, Bacillus subtilis |
10 | Biocontrol of Root Diseases | Protects against soil-borne pathogens like Fusarium, Pythium, and Rhizoctonia | Paenibacillus polymyxa, Bacillus spp. |
11 | Detoxification of Pathogen Toxins | Some bacteria degrade toxic compounds released by pathogens, reducing damage to plants | Pseudomonas spp., Actinobacteria |
12 | Mycorrhizal Symbiosis Enhancement | Helps beneficial fungi like mycorrhizae colonize roots, improving plant resistance | Bacillus spp., Paenibacillus |
13 | Protection Against Viral Diseases | Some bacteria interfere with viral replication in plants, reducing viral infections | Pseudomonas spp., Streptomyces spp. |
14 | Immune Priming | Triggers mild immune responses in plants, preparing them for future pathogen attacks | Pseudomonas fluorescens, Bacillus spp. |
15 | Reduction of Plant Stress | Helps plants tolerate environmental stress (drought, salinity), reducing susceptibility to diseases | Azospirillum, Herbaspirillum |
16 | Disruption of Pathogen Biofilms | Breaks down biofilms formed by harmful bacteria, making them more vulnerable to plant defenses | Bacillus spp., Lactobacillus |
17 | Insect Pest Suppression | Produces toxins or metabolites that deter or kill insect pests that spread diseases | Bacillus thuringiensis (Bt) |
18 | Root Colonization & Protection | Colonizes root surfaces, preventing pathogenic bacteria and fungi from taking hold | Pseudomonas putida, Paenibacillus |
19 | Production of Plant Hormones | Produces auxins, gibberellins, and cytokinins that strengthen plant growth and immunity | Azospirillum, Bacillus |
20 | Biological Seed Coating | Seeds treated with beneficial bacteria germinate with built-in protection against soil pathogens | Pseudomonas spp., Bacillus spp. |
A. Beneficial Microbes That Outcompete Harmful Pathogens
Beneficial microbes play a pivotal role in outcompeting harmful pathogens in the soil, thereby enhancing plant health and productivity. These beneficial microorganisms, which include specific strains of bacteria and fungi, establish symbiotic relationships with plant roots. This interaction creates a protective barrier that inhibits pathogen colonization around the plant’s root zone. By producing antimicrobial compounds and competing for essential nutrients and physical space within the soil, these organisms effectively suppress the growth of harmful pathogens that threaten plant integrity. In addition to their competitive advantages, the activities of these beneficial microbes also stimulate plant immune responses, further bolstering the plant’s natural resistance against various diseases. The intricate interactions between these beneficial microbes and plant roots are vividly illustrated in studies and research, which showcase how rhizosphere microbes enhance plant health and resilience by engaging in complex biochemical exchanges. This dynamic relationship not only aids in preventing disease but also contributes significantly to vital ecological processes, such as nutrient cycling and improvement of soil structure, which are crucial for sustainable agricultural systems. Ultimately, harnessing the power of beneficial microbes presents an eco-friendly strategy for sustainable agriculture, offering an effective alternative to conventional chemical pesticides. By integrating these natural allies into farming practices, farmers can significantly reduce their reliance on synthetic chemicals while simultaneously promoting plant growth, health, and overall ecosystem vitality. The role of beneficial microbes serves as a testament to the importance of biodiversity in agriculture and the potential of natural solutions to foster environmental balance and crop success.
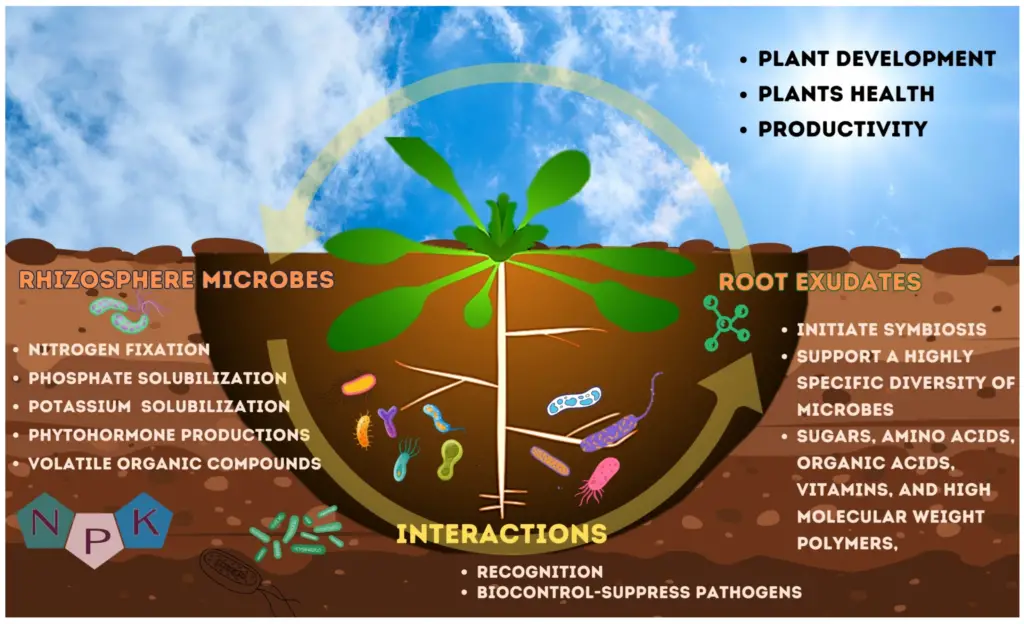
Image : Interactions Between Rhizosphere Microbes and Plant Roots (The image illustrates the interactions between rhizosphere microbes and plant roots, emphasizing the importance of microbes in plant development and health. It highlights key processes such as nitrogen fixation, phosphate solubilization, and potassium solubilization, as well as the role of root exudates in initiating symbiotic relationships and supporting microbial diversity. The visual representation provides a clear connection between the microbial activities in the soil and their contributions to plant productivity. This diagram is particularly useful for studies related to soil science, plant biology, and agricultural productivity.)
Microbe Type | Role | Benefit | Source |
Trichoderma spp. | Outcompetes harmful fungi | Enhances plant growth and disease resistance | Journal of Fungi, 2021 |
Bacillus subtilis | Inhibits bacterial pathogens | Promotes plant health and yield | Applied Soil Ecology, 2022 |
Pseudomonas fluorescens | Suppresses soil-borne pathogens | Improves nutrient availability | Soil Biology & Biochemistry, 2020 |
Mycorrhizae | Forms symbiotic relationships with plants | Increases nutrient uptake and disease resistance | Mycorrhiza, 2023 |
Lactobacillus spp. | Outcompetes harmful bacteria | Boosts soil health and microbial diversity | Agricultural Sciences, 2021 |
Beneficial Microbes vs. Harmful Pathogens in Soil
B. The Use of Bacteria in Organic Farming and Biopesticides
The integration of bacteria in organic farming and the application of biopesticides exemplify a sustainable approach to agriculture that harnesses the natural processes vital for plant health and soil fertility. Utilizing specific strains of beneficial bacteria enables the enhancement of nitrogen fixation, promotes plant growth, and aids in the decomposition of organic matter, which is crucial for maintaining soil fertility. These beneficial bacteria work symbiotically with the plants, enhancing their ability to absorb essential nutrients from the soil, thus culminating in healthier and more robust crops. Furthermore, biopesticides derived from bacteria can effectively control pest populations while minimizing environmental impact, as they specifically target harmful pests without adversely affecting beneficial organisms such as pollinators and predatory insects. This specificity is particularly important as it helps maintain the ecological balance in agricultural ecosystems. Additionally, the use of bacteria in this context reduces the need for synthetic chemicals, thereby lessening the risk of chemical runoff into waterways and contributing to a healthier environment overall. This synergy between plant-microbe interactions fosters a resilient ecosystem, promoting biodiversity and reducing reliance on synthetic chemicals. The role of these bacteria is visually represented in below images, which illustrates how Plant Growth-Promoting Bacteria (PGPB) enhance plant resilience against environmental stressors while facilitating nutrient uptake. This diagram exemplifies the dynamic relationships at play in healthy soils where bacteria thrive. Collectively, this microbial activity underscores the critical importance of bacteria in developing sustainable agricultural practices that promote health and productivity within organic farming systems, shaping a future where food production is both effective and environmentally friendly.
Table : Beneficial Microbes That Outcompete Harmful Pathogens
Example No. | Beneficial Microbe | Type | Mechanism of Action | Targeted Pathogens/Diseases | Example Crops Protected |
---|---|---|---|---|---|
1 | Pseudomonas fluorescens | Bacteria (PGPR) | Produces antibiotics, siderophores, and enzymes that degrade fungal cell walls | Fusarium wilt, Pythium, Rhizoctonia | Wheat, rice, vegetables |
2 | Bacillus subtilis | Bacteria (PGPR) | Forms biofilms, produces antifungal compounds, and induces systemic resistance | Botrytis (gray mold), powdery mildew | Grapes, tomatoes, cucumbers |
3 | Trichoderma harzianum | Fungi | Competes for space and nutrients, produces antifungal enzymes | Root rot, damping-off disease | Beans, soybeans, corn |
4 | Streptomyces spp. | Actinobacteria | Produces antibiotics that suppress bacterial and fungal pathogens | Bacterial wilt, Fusarium spp. | Potatoes, tomatoes, peppers |
5 | Lactobacillus spp. | Lactic Acid Bacteria | Lowers pH, inhibits pathogen growth with organic acids | Post-harvest rots, fungal infections | Fruits, leafy greens |
6 | Azospirillum brasilense | Nitrogen-Fixing Bacteria | Outcompetes soil-borne pathogens by rapid colonization | Root pathogens like Fusarium | Maize, wheat, sugarcane |
7 | Paenibacillus polymyxa | Bacteria (PGPR) | Secretes antimicrobial peptides, fixes nitrogen | Verticillium wilt, damping-off | Cotton, potatoes, strawberries |
8 | Rhizobium spp. | Nitrogen-Fixing Bacteria | Enhances plant immunity while improving nitrogen availability | Soil-borne fungal diseases | Legumes (peas, lentils, soybeans) |
9 | Bacillus amyloliquefaciens | Bacteria (PGPR) | Produces antifungal metabolites and siderophores | Powdery mildew, bacterial leaf spot | Grapes, strawberries, lettuce |
10 | Frankia spp. | Actinobacteria | Competes with soil pathogens and fixes nitrogen | Root rot pathogens | Alders, Casuarina trees |
11 | Burkholderia cepacia | Bacteria (PGPR) | Inhibits fungal pathogens via antimicrobial compounds | Fusarium, Pythium | Onions, potatoes, peanuts |
12 | Gluconacetobacter diazotrophicus | Endophytic Bacteria | Enhances plant growth, indirectly reducing disease susceptibility | Soil-borne diseases | Sugarcane, rice, bananas |
13 | Anabaena spp. | Cyanobacteria | Fixes nitrogen, improves plant health, and competes with pathogens | Rice blast disease | Rice, aquatic plants |
14 | Serratia marcescens | Bacteria (PGPR) | Produces proteases that degrade fungal cell walls | Root pathogens like Fusarium | Tomatoes, cucumbers, melons |
15 | Pseudomonas putida | Bacteria (PGPR) | Produces siderophores, competes for iron and nutrients | Bacterial wilt, damping-off | Wheat, soybeans, vegetables |
16 | Beauveria bassiana | Entomopathogenic Fungi | Controls insect pests, reducing disease transmission | Aphids, whiteflies (which spread viruses) | Fruit trees, cotton, coffee |
17 | Metarhizium anisopliae | Entomopathogenic Fungi | Targets insect vectors of plant diseases | Thrips, weevils, nematode carriers | Vegetables, grains |
18 | Saccharomyces cerevisiae | Yeast | Outcompetes fungal pathogens by rapid colonization | Powdery mildew, post-harvest rot | Grapes, apples, citrus |
19 | Actinobacteria spp. | Soil Microbe | Secretes antibiotics that inhibit soil-borne fungi | Fusarium, Verticillium wilt | Peanuts, potatoes, soybeans |
20 | Chryseobacterium spp. | Bacteria (PGPR) | Produces enzymes that degrade pathogen biofilms | Bacterial canker, leaf spot | Stone fruits, peppers |
V. How Understanding Soil Bacteria Can Improve Sustainable Agriculture
A comprehensive understanding of soil bacteria is pivotal for enhancing sustainable agricultural practices, particularly in the realms of nutrient cycling and plant health. Soil bacteria play an essential role in the nitrogen cycle, as depicted in the nitrogen cycle image, where they convert atmospheric nitrogen into forms accessible for plant uptake, thereby significantly reducing reliance on synthetic fertilizers that can harm the environment. Additionally, beneficial soil bacteria influence plant growth through various mechanisms such as the production of phytohormones and the facilitation of nutrient solubilization, which are vital for crops grown in nutrient-poor conditions, as outlined in the Plant Growth-Promoting Bacteria framework. These processes not only assist in nutrient availability but also promote a healthier root system, leading to increased crop yield and quality. By fostering microbial diversity in the soil, farmers can improve crop resilience against stresses, including drought and pest pressures, while also promoting organic matter decomposition. This decomposition process is crucial for releasing essential nutrients back into the soil ecosystem, creating a cycle of regeneration that supports agricultural sustainability. Moreover, the presence of diverse soil bacteria can enhance soil structure, improve moisture retention, and contribute to carbon sequestration, thereby playing a significant role in climate change mitigation. This symbiotic relationship underscores the importance of soil health in achieving sustainable agricultural systems, thereby bridging the gap between ecological viability and agricultural productivity. Ultimately, recognizing the importance of soil bacteria not only supports the cultivation of healthier crops but also leads to more sustainable farming practices that can benefit the environment and future farming generations.
Table : How Understanding Soil Bacteria Improves Sustainable Agriculture
Type No. | Soil Bacteria Type | Function in Agriculture | Benefits for Sustainable Farming | Examples of Bacteria |
---|---|---|---|---|
1 | Nitrogen-Fixing Bacteria | Convert atmospheric nitrogen (N₂) into plant-usable forms | Reduces reliance on synthetic nitrogen fertilizers, improving soil health | Rhizobium, Azotobacter, Frankia |
2 | Phosphate-Solubilizing Bacteria (PSB) | Break down insoluble phosphate into forms plants can absorb | Enhances phosphorus availability, reducing need for chemical fertilizers | Pseudomonas putida, Bacillus megaterium |
3 | Potassium-Solubilizing Bacteria (KSB) | Release potassium from soil minerals | Improves crop stress tolerance and yield | Bacillus mucilaginosus, Frateuria aurantia |
4 | Plant Growth-Promoting Rhizobacteria (PGPR) | Enhance root development and nutrient uptake | Increases plant resilience to environmental stress and reduces input costs | Bacillus subtilis, Pseudomonas fluorescens |
5 | Decomposer Bacteria | Break down organic matter into essential nutrients | Improves soil fertility and organic carbon levels | Actinobacteria, Bacillus spp. |
6 | Biocontrol Bacteria | Produce antibiotics and enzymes that suppress plant pathogens | Reduces need for chemical pesticides, promoting eco-friendly pest management | Trichoderma, Streptomyces, Bacillus thuringiensis |
7 | Siderophore-Producing Bacteria | Produce iron-chelating compounds, depriving pathogens of iron | Inhibits growth of harmful microbes, improving plant defense | Pseudomonas aeruginosa, Bacillus amyloliquefaciens |
8 | Mycorrhizal-Associated Bacteria | Help beneficial fungi colonize plant roots | Enhances nutrient and water absorption efficiency | Bacillus simplex, Pseudomonas synxantha |
9 | Organic Matter Decomposers | Break down crop residues and manure | Promotes nutrient recycling and soil structure improvement | Cellulomonas, Paenibacillus polymyxa |
10 | Drought-Tolerant Bacteria | Improve plant water-use efficiency | Helps crops withstand water scarcity and climate change effects | Azospirillum brasilense, Bacillus licheniformis |
11 | Salt-Tolerant Bacteria | Aid plant growth in saline soils | Reclaims degraded land for agriculture | Halomonas, Pseudomonas stutzeri |
12 | Carbon-Sequestering Bacteria | Capture and store atmospheric carbon in the soil | Reduces carbon footprint, mitigating climate change | Bradyrhizobium, Frankia |
13 | Biofertilizer-Producing Bacteria | Provide essential nutrients naturally | Reduces dependency on synthetic fertilizers, promoting organic farming | Azotobacter, Phosphobacteria |
14 | Compost-Enriching Bacteria | Accelerate compost decomposition | Produces nutrient-rich soil amendments for sustainable farming | Bacillus thermoamylovorans, Lactobacillus |
15 | Heavy Metal Detoxifying Bacteria | Remediate toxic soils by breaking down pollutants | Reduces soil contamination, making land safer for farming | Pseudomonas putida, Rhodococcus |
16 | Methanotrophic Bacteria | Consume methane, a potent greenhouse gas | Lowers greenhouse gas emissions from agriculture | Methylobacterium, Methylosinus |
17 | Disease-Suppressing Bacteria | Protect against soil-borne pathogens | Reduces crop losses, improving farm productivity | Serratia marcescens, Bacillus cereus |
18 | Microbial Consortia | Work together to improve soil health | Increases biodiversity and ecosystem stability | Rhizobium + Bacillus, Trichoderma + Pseudomonas |
19 | Nutrient Cycling Bacteria | Decompose dead organisms and release nutrients | Maintains soil fertility without synthetic inputs | Nitrosomonas, Nitrobacter |
20 | Biofilm-Forming Bacteria | Protect plant roots and increase drought resistance | Enhances root health and resilience to stress | Pseudomonas fluorescens, Bacillus subtilis |
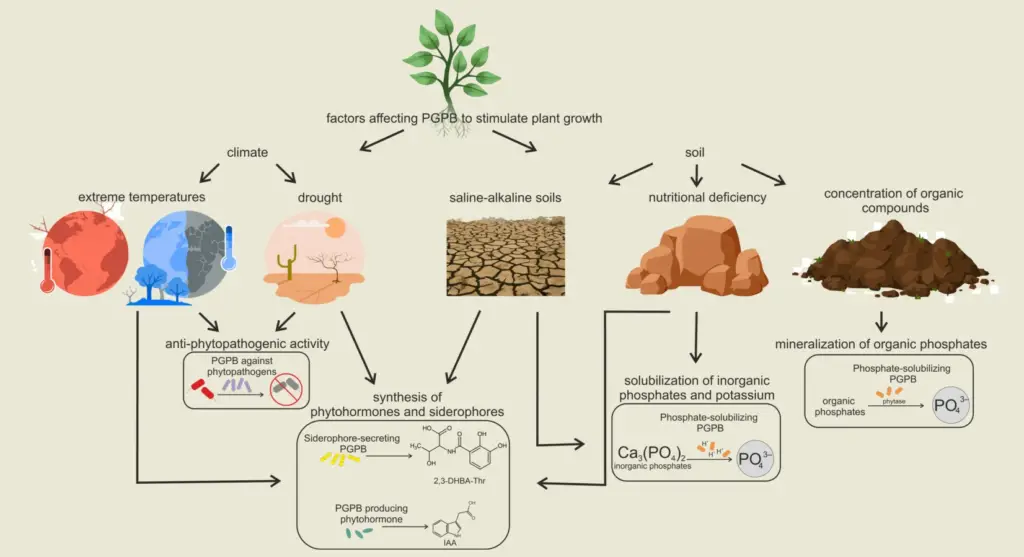
Image : Factors Affecting PGPB in Stimulating Plant Growth (The image illustrates the factors affecting Plant Growth-Promoting Bacteria (PGPB) that stimulate plant growth. It depicts various stressors such as extreme temperatures, drought, saline-alkaline soils, soil nutritional deficiency, and the concentration of organic compounds. The diagram further explains how these factors lead to anti-phytopathogenic activity, synthesis of phytohormones and siderophores, and solubilization of inorganic phosphates and potassium, essential processes for improving plant health and resilience. Each component is visually represented, helping to clarify their relationships and contributions to plant growth.)
REFERENCES
- Sue Ellen Johnson. ‘Crop Rotation on Organic Farms.’ A Planning Manual, Charles L. Mohler, Natural Resource, Agriculture, and Engineering Service (NRAES) Cooperative Extension, 1/1/2009
- K. E. Giller. ‘Nitrogen Fixation in Tropical Cropping Systems.’ CABI, 1/1/2001
- Felix Bärlocher. ‘Methods to Study Litter Decomposition.’ A Practical Guide, Manuel A.S. Graça, Springer Science & Business Media, 4/5/2005
- Zdenko Rengel. ‘Nutrient Cycling in Terrestrial Ecosystems.’ Petra Marschner, Springer Science & Business Media, 5/1/2007
- Dinesh K. Maheshwari. ‘Plant Growth and Health Promoting Bacteria.’ Springer Science & Business Media, 9/28/2010
- Eldor Paul. ‘Soil Microbiology, Ecology and Biochemistry.’ Academic Press, 11/14/2014
- Alistair McCleery. ‘An Introduction to Book History.’ David Finkelstein, Routledge, 3/13/2006
Image References:
- Image: Illustration of Root Foraging Response Mechanism in Plants, Accessed: 2025.https://www.mdpi.com/microorganisms/microorganisms-10-02399/article_deploy/html/images/microorganisms-10-02399-g001.png
- Image: Illustration of Nitrogen Cycle Processes, Accessed: 2025.https://www.mdpi.com/nitrogen/nitrogen-04-00022/article_deploy/html/images/nitrogen-04-00022-g001.png
- Image: Interactions Between Rhizosphere Microbes and Plant Roots, Accessed: 2025.https://www.mdpi.com/microorganisms/microorganisms-12-00558/article_deploy/html/images/microorganisms-12-00558-g001.png
- Image: Illustration of the Nitrogen Cycle in Agriculture, Accessed: 2025.https://extension.umn.edu/sites/extension.umn.edu/files/biological-nitrogen-fixation-graphic.png
- Image: Factors Affecting PGPB in Stimulating Plant Growth, Accessed: 2025.https://pub.mdpi-res.com/plants/plants-12-04074/article_deploy/html/images/plants-12-04074-ag.png?1701766954