Ecological Pyramids: Types, Characteristics, and Limitations
I. Introduction
Ecological pyramids serve as vital tools for understanding the relationships and energy dynamics within ecosystems, offering a visual representation of how energy and biomass are distributed among various trophic levels. These pyramids can be categorized into three primary types: the pyramid of numbers, the pyramid of biomass, and the pyramid of energy, each illustrating distinct aspects of ecological organization. While these frameworks provide invaluable insights into the structure and functioning of ecosystems, they also come with certain limitations, including oversimplification of complex ecological interactions and varying methodologies for measurement. This essay will explore the types of ecological pyramids, their characteristics, and their inherent limitations, analyzing how these frameworks enrich our comprehension of ecological principles and the implications for conservation efforts. Through this examination, the significance of ecological pyramids in the broader context of environmental science will be established, emphasizing their role in guiding ecological research and preserving biodiversity.
Type | Characteristics | Limitations |
Pyramid of Numbers | Shows the number of individual organisms at each trophic level. | Does not account for the size of organisms; large trees may outweigh numerous herbivores. |
Pyramid of Biomass | Represents the total mass of living matter at each trophic level. | Seasonal changes can affect biomass; requires specific time frames for accurate measurement. |
Pyramid of Energy | Illustrates the flow of energy through each trophic level, measured in joules. | Difficult to measure accurately; energy flow is often lost as heat. |
Ecological Pyramids Data
Definition and Importance of Ecological Pyramids
Ecological pyramids serve as vital tools for understanding the dynamics of energy flow and trophic relationships within ecosystems. Defined as graphical representations that categorize organisms into different levels based on their energy roles, these pyramids typically include three primary types: the pyramid of numbers, the pyramid of biomass, and the pyramid of energy. Each type elucidates crucial aspects of ecological structure— from the sheer number of organisms at varying trophic levels to the total biomass they collectively represent. Most notably, they illustrate the inefficiencies of energy transfer, where significant energy loss occurs at each trophic level, enhancing our understanding of ecological balance and productivity. As highlighted by recent research, ecological pyramids also reflect responses to anthropogenic pressures, underscoring their importance in conservation biology and resource management (A Friedlander et al.), (Andersen et al.). By leveraging these frameworks, ecologists can predict shifts in biodiversity and community dynamics in the face of environmental change.
Type | Description | Example Organisms | Importance |
Pyramid of Numbers | Illustrates the number of individual organisms at each trophic level. | 1000 plants, 100 herbivores, 10 carnivores | Shows the distribution of organisms, indicating ecosystem health. |
Pyramid of Biomass | Represents the total mass of living matter at each trophic level. | 500 kg of plants, 100 kg of herbivores, 10 kg of carnivores | Indicates the energy available at each level, essential for energy flow analysis. |
Pyramid of Energy | Depicts the energy flow through each trophic level, measured in kilocalories. | 5000 kcal for producers, 1000 kcal for primary consumers, 100 kcal for secondary consumers | Highlights the efficiency of energy transfer and ecosystem productivity. |
Ecological Pyramid Data
II. Types of Ecological Pyramids
The exploration of ecological pyramids reveals three primary types: the pyramid of numbers, the pyramid of biomass, and the pyramid of energy. Each type offers a unique perspective on the structure and dynamics of ecosystems. The pyramid of numbers illustrates the population size of different trophic levels, emphasizing the decreasing number of organisms from producers to apex predators. In contrast, the pyramid of biomass accounts for the total mass of living matter at each level, providing insight into the energy stored within each trophic stage. The pyramid of energy is particularly significant, as it delineates the flow and conversion of energy through the ecosystem, highlighting inefficiencies in energy transfer. According to recent studies, these pyramidal models not only depict biological interactions but also reflect the impacts of anthropogenic pressures on ecosystems, underscoring the need for sustainable practices as outlined in frameworks that connect human and ecological well-being (A Friedlander et al.), (Butkus et al.).
Type | Description | Example |
Pyramid of Numbers | Represents the number of organisms at each trophic level. | A single tree may support numerous insects, representing a higher number at the producer level. |
Pyramid of Biomass | Illustrates the total mass of living matter at each trophic level. | The biomass of all producers is generally greater than that of primary consumers. |
Pyramid of Energy | Displays the flow of energy through each trophic level over a specific time period. | Energy is lost at each trophic level, typically following the 10% rule. |
Types of Ecological Pyramids
Pyramid of Numbers, Biomass, and Energy
The Pyramid of Numbers, Biomass, and Energy serves as a foundational concept in ecological studies, illustrating the hierarchical structure of ecosystems. Each type of pyramid reflects different aspects of ecological interactions, revealing the relationship between organisms at various trophic levels. The Pyramid of Biomass, for example, depicts the total mass of living matter at each level, thereby indicating the energy that can be retrieved by higher trophic organisms. In contrast, the Pyramid of Numbers emphasizes the count of individual organisms within each tier. These pyramids not only aid in visualizing energy flow and trophic dynamics but also underscore the limitations inherent in ecosystems, particularly in their capacity to support biomass as environmental changes occur. Such changes, often driven by anthropogenic activities, can disrupt the balance depicted in these pyramids, leading to phenomena such as hypoxia in aquatic systems, as noted in (A Friedlander et al.) and (II B et al.).
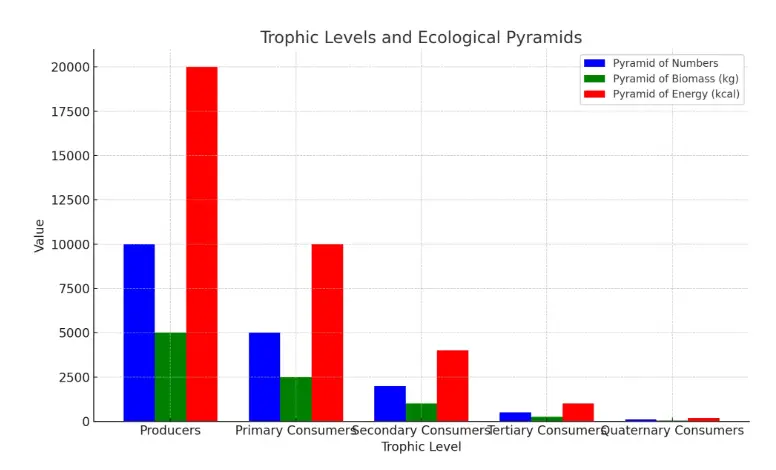
The chart presents the ecological pyramids for different trophic levels, illustrating the Pyramid of Numbers, Pyramid of Biomass in kilograms, and Pyramid of Energy in kilocalories. The data shows that producers have the highest values across all three pyramids, while higher trophic levels like quaternary consumers show significantly reduced numbers, biomass, and energy, reflecting the diminishing energy transfer through the food chain.
III. Characteristics of Ecological Pyramids
Ecological pyramids, including pyramids of numbers, biomass, and energy, serve as vital tools for understanding the dynamics of ecosystems. Characteristically, these pyramids illustrate the hierarchical structure of energy flow and organism distribution across various trophic levels. At the base, producers form the broadest layer, capturing solar energy and supplying it to herbivores and subsequently to carnivores, which occupy higher levels. A notable feature is the significant energy loss at each trophic transition, typically around 90%, leading to a decrease in biomass and species abundance higher up the pyramid. Moreover, ecological pyramids are pivotal in modeling ecosystem responses to stressors, as detailed in studies involving mechanistic models of ecosystem structure, highlighting the intricate interplay between biotic components and environmental conditions (A Friedlander et al.). Additionally, the principles of sustainability and the common good, as explored within Catholic social teaching, further contextualize the significance of these ecological models in addressing environmental crises (Butkus et al.).
Type | Definition | Characteristics | Example Organisms |
Pyramid of Numbers | Displays the number of individual organisms at each trophic level in an ecosystem. | Can be inverted, shows primary producers at the base, and may vary with season. | Trees, herbivores, carnivores |
Pyramid of Biomass | Represents the total mass of living matter at each trophic level. | Generally upright, biomass decreases at higher trophic levels, indicates energy availability. | Phytoplankton, zooplankton, fish |
Pyramid of Energy | Illustrates the flow of energy through different trophic levels over time. | Always upright, demonstrates energy loss at each level, based on production over a specific time. | Producers, primary consumers, secondary consumers |
Characteristics of Ecological Pyramids
Structural Features and Ecological Significance
The structural features of ecological pyramids, such as energy, biomass, and numbers, highlight significant relationships between organisms and their environment, illustrating the flow of energy through trophic levels. The energy pyramid, for instance, not only demonstrates the diminishing energy available at successive levels but also emphasizes the importance of primary producers, which form the foundation of ecosystems by capturing solar energy through photosynthesis. This foundational role underpins the entire food web, as secondary and tertiary consumers depend on these producers for sustenance and energy (Delmas et al.). Similarly, research on reef sharks reveals how they interact within their ecosystems, influencing the dynamics of coral reefs by regulating prey populations and maintaining biodiversity (Bierwagen et al.). Thus, understanding the structural intricacies of ecological pyramids enhances our comprehension of ecological dynamics and the ecological significance of species interactions within various environments.
Type | Example | Producers | Primary Consumers | Secondary Consumers | Tertiary Consumers | Notes |
Pyramid of Numbers | Oak Tree Ecosystem | 1000 | 500 | 50 | 5 | Shows the number of individual organisms at each trophic level. |
Pyramid of Biomass | Freshwater Pond Ecosystem | 300 | 200 | 75 | 10 | Illustrates the total mass of living material at each trophic level. |
Pyramid of Energy | Terrestrial Grassland Ecosystem | 2000 | 1000 | 300 | 50 | Depicts the flow of energy through each trophic level over time. |
Ecological Pyramid Data
IV. Limitations of Ecological Pyramids
While ecological pyramids serve as valuable tools for visualizing energy dynamics and organism distribution within ecosystems, they exhibit notable limitations that hinder their comprehensiveness. One significant drawback is their failure to account for omnivorous species that occupy multiple trophic levels, leading to potential inaccuracies in depicting energy transfer and population sizes. Additionally, ecological pyramids often simplify complex food webs, neglecting the intricate interdependencies among species that contribute to ecosystem stability. Furthermore, these models can misrepresent the actual biomass and energy present in ecosystems, especially in aquatic environments where inverted pyramids may arise due to high primary producer biomass such as phytoplankton. This limitation is compounded by the static nature of pyramids, failing to capture temporal changes in populations and energy flow, which are essential for understanding ecological dynamics over time. Such considerations underscore the need for complementary models to enhance our understanding of ecological interactions (Singh J et al.), (Ceschin F et al., p. 118-163).
Limitation | Description | Impact Level |
Simplicity of Representation | Ecological pyramids often oversimplify complex ecosystems, ignoring species interactions and biodiversity. | High |
Temporal Changes | Ecological pyramids are often static and may not represent dynamic changes in populations over time. | Medium |
Biomass vs. Energy Flow | Pyramids may not accurately represent energy flow, as biomass does not equate to energy available at each trophic level. | High |
Data Availability | In many cases, accurate data on species numbers and biomass is not readily available, leading to erroneous representations. | Medium |
Trophic Level Overlap | Some species exist at multiple trophic levels, complicating the categorization in ecological pyramids. | Medium |
Focus on Quantitative Data | Ecological pyramids heavily rely on quantitative data, potentially neglecting qualitative aspects of ecosystems. | Low |
Limitations of Ecological Pyramids Data
Challenges in Representation and Interpretation
The representation and interpretation of ecological pyramids present several challenges that can obscure the complexities of ecosystem dynamics. One significant issue lies in the oversimplification of trophic structures, where the intricate relationships between species are often distilled into linear hierarchies. This reductionist approach may fail to capture the nuances of energy transfer and species interactions, potentially leading to misleading conclusions about ecological balance. Moreover, the visual depictions of ecological pyramids can exaggerate the differences in population sizes or energy flow among trophic levels, creating a distorted understanding of ecosystem function. Recent studies suggest that such inaccuracies can impede effective environmental policy formulation, emphasizing the need for improved analytical techniques, including those discussed in materials flow analysis, that consider stock demographics in material management (Allwood J M et al.). Therefore, addressing these challenges is crucial to enhance our comprehension and management of ecological systems (Andersen et al.).
V. Conclusion
In conclusion, the examination of ecological pyramids reveals not only the intricate hierarchical relationships within ecosystems but also highlights significant implications for sustainability and social equity. Understanding the structure of these pyramids, from producers to apex predators, underscores the critical importance of each trophic level in maintaining ecological balance. Moreover, as discussed in the context of Catholic social teaching, the connection between environmental stewardship and the common good becomes increasingly apparent; sustainability should be integrated into developmental frameworks that ensure justice and equity among communities (Butkus et al.). Additionally, while ecological pyramids provide valuable insights, limitations such as energy loss and biodiversity decline, exacerbated by human actions, must be addressed. A concerted effort to mitigate these challenges through practices such as plastic up-cycling exemplifies the potential for harmonious coexistence with nature while achieving sustainability goals (Li et al.). Thus, fostering awareness and action is essential for the preservation of ecological integrity.
Summary of Key Points and Implications for Ecology
In summary, ecological pyramids serve as fundamental tools for understanding energy flow and biomass distribution within ecosystems. They come in three primary types: energy pyramids, biomass pyramids, and pyramids of numbers, each elucidating different aspects of ecological dynamics. Energy pyramids highlight the significant energy loss at each trophic level, typically illustrating that only about ten percent of energy is transferred upwards, which has profound implications for species abundance and ecosystem stability. Biomass pyramids, on the other hand, emphasize the total mass of organisms at each level, revealing the often stark contrasts between producers and consumers. Additionally, pyramids of numbers represent the sheer quantity of organisms per trophic level, which can vary widely across different ecosystems. Ultimately, a deeper understanding of these pyramids aids in the assessment of ecological health and the management of biodiversity, making them crucial for conservation efforts and sustainable ecosystem management.
References:
- Fabrizio Ceschin, İdil Gaziulusoy. “Evolution of design for sustainability: From product design to design for system innovations and transitions”. Design Studies, 2016, https://doi.org/10.1016/j.destud.2016.09.002
- A Friedlander, A Hardisty, B Cho, B Collen, B-E Sæther, BJ McGill, C Mora, et al.. “Emergent global patterns of ecosystem structure and function from a mechanistic general ecosystem model”. ‘Public Library of Science (PLoS)’, 2014, https://core.ac.uk/download/20048026.pdf
- Butkus, Russell, Kolmes, Steven. “Ecology and the Common Good: Sustainability and Catholic Social Teaching”. 2007, https://core.ac.uk/download/210995274.pdf
- Andersen, Erling, Bezlepkina, Irina V., Brouwer, Floor M., Ewert, et al.. “Development of a conceptual framework for integrated analysis and assessment of agricultural systems in SEAMLESS-IF”. 2025, https://core.ac.uk/download/pdf/6413882.pdf
- Allwood J. M., André Cabrera Serrenho, Birat J.-P., CAR, Fischedick M., ICCT, Julian M. Allwood, et al.. “Material Stock Demographics: Cars in Great Britain.”. Environ Sci Technol, 2016, https://core.ac.uk/download/35280448.pdf
- Li, Bwen. “Transformation from High Density Polyethylene Waste to a Multifunctional Diamond”. 2012, https://core.ac.uk/download/84320393.pdf
- Brumfield II, Jimmie Arnold. “Dynamic model of ecological factors impacting the Gulf of Mexico dead zone”. LSU Digital Commons, 2010, https://core.ac.uk/download/217399844.pdf
- Delmas, Eva. “What does a bioenergetic network approach tell us about the functioning of ecological communities?”. 2020, https://papyrus.bib.umontreal.ca/xmlui/bitstream/1866/24832/2/Delmas_Eva_2020_these.pdf
- Bierwagen, Stacy L.. “Functional roles of reef sharks on the Great Barrier Reef”. 2019, https://core.ac.uk/download/351178087.pdf
- Jagpreet Singh, Tanushree Dutta, Ki‐Hyun Kim, Mohit Rawat, Pallabi Samddar, Pawan Kumar. “‘Green’ synthesis of metals and their oxide nanoparticles: applications for environmental remediation”. Journal of Nanobiotechnology, 2018, https://doi.org/10.1186/s12951-018-0408-4