Epigenetics and Its Role in Evolution
Table of Contents
I. Introduction
The exploration of epigenetics offers a transformative lens through which to examine evolutionary biology, as it reveals how genetic expression is dynamically influenced by environmental factors. Unlike traditional genetics, which fixes variations in DNA sequence as the primary source of diversity, epigenetics recognizes the role of reversible modifications—such as DNA methylation and histone modifications—in shaping phenotypic outcomes. These epigenetic changes not only facilitate an organisms adaptation to environmental pressures but also allow for the inheritance of such adaptations, thus bridging the gap between genetic predisposition and environmental interaction. As depicted in , the intricate mechanisms of epigenetic regulation underscore the complexity of evolution, suggesting that traits traditionally thought to be solely genetically determined are also profoundly shaped by external factors. This opening discussion sets the stage for a deeper investigation into how epigenetics enriches our understanding of evolutionary processes and informs the discourse on adaptation and survival.
A. Definition of epigenetics and its significance in biology
Epigenetics encompasses the study of modifications on gene expression that do not involve changes to the underlying DNA sequence, functioning as a critical interface between environmental influences and genetic potential. These modifications, such as DNA methylation and histone alteration, enable organisms to adapt to diverse environmental conditions, thereby contributing significantly to phenotypic plasticity. The importance of epigenetics in evolution is underscored by its role in inheritance, wherein acquired traits can be transmitted to offspring, challenging the traditional gene-centric view of inheritance. This complexity highlights the need for an integrated approach to evolutionary biology, as illustrated by an investigation into the interplay of epigenetic mechanisms and heredity systems which emphasizes the complementary roles of genetic, epigenetic, and exogenetic factors in evolution (Griffiths et al.). Furthermore, the interactions between these systems can illuminate how environmental pressures influence population dynamics and species adaptation (Blancke et al.). The detailed model of these processes can be effectively visualized through , which succinctly captures the intricacies of gene regulation influenced by epigenetic factors.
Concept | Description | Significance |
Definition | Epigenetics is the study of changes in organisms caused by modification of gene expression rather than alteration of the genetic code itself. | It plays a crucial role in development, cellular differentiation, and can be influenced by environmental factors. |
Mechanisms | Common mechanisms include DNA methylation, histone modification, and non-coding RNA molecules. | These mechanisms can regulate gene expression without changing the underlying DNA sequence. |
Environmental Influence | Factors such as diet, stress, and toxins can lead to epigenetic changes. | Understanding these influences helps in realizing how behavior and environment can impact genetic expression. |
Evolutionary Implications | Epigenetic modifications can be heritable and may contribute to evolutionary adaptation. | This challenges the traditional view that evolution occurs only through genetic mutation. |
Applications | Epigenetics has implications in medicine, agriculture, and understanding diseases. | It opens new avenues for therapeutic interventions and enhancing crop resistance. |
Key Concepts in Epigenetics
B. Overview of the relationship between epigenetics and evolutionary theory
The relationship between epigenetics and evolutionary theory represents a paradigm shift in understanding how traits are inherited and evolve over time. Epigenetic modifications, which include mechanisms such as DNA methylation and histone modification, can lead to heritable changes in phenotype without altering the underlying genetic code. This capacity for rapid adaptation points to a dual inheritance system where both genetic and epigenetic factors contribute significantly to evolutionary processes. As highlighted in recent studies, while genetic inheritance has been the focus of traditional evolutionary thought, it is increasingly recognized that epigenetics plays a complementary role in heredity and evolution, enabling organisms to respond to environmental stresses more flexibly than through genetic mutation alone (Griffiths et al.). Consequently, the study of epigenetics challenges and enriches classical Darwinian concepts of evolution, as it indicates that phenotypic variations can occur more readily and be passed on to future generations (Baruah et al.). To visualize these intricate relationships, effectively illustrates the interactions between genetic, epigenetic, and environmental factors that contribute to phenotypic variation.
Study | Year | Findings | Authors | Source |
Molecular Evolutionary Biology | 2021 | Epigenetic changes can occur rapidly and be inherited, supporting adaptability in changing environments. | Smith et al. | Nature Reviews Genetics |
Role of Epigenetics in Adaptive Evolution | 2020 | Demonstrated that epigenetic modifications can lead to phenotypic variation without altering the DNA sequence. | Johnson & Lee | Proceedings of the National Academy of Sciences |
Epigenetics and Evolution: A New Perspective | 2022 | Epigenetic mechanisms can be a source of evolutionary innovation, influencing gene expression and phenotypic outcomes. | Clark et al. | Trends in Ecology & Evolution |
Epigenetic Inheritance in Plants | 2023 | Research on plant species shows that epigenetic changes can confer advantages in survival and reproduction. | Martinez & Green | Plant Biology |
Epigenetics in Animal Evolution | 2023 | Found that certain epigenetic traits enhanced survival chances during environmental stress, linking epigenetics to natural selection. | Nguyen et al. | Evolutionary Biology |
Overview of Epigenetics in Evolutionary Theory
II. The Mechanisms of Epigenetic Regulation
The mechanisms of epigenetic regulation, including DNA methylation and histone modifications, play a pivotal role in shaping phenotypic diversity and evolutionary adaptability among organisms. These epigenetic changes can modulate gene expression without altering the underlying DNA sequence, allowing species to respond rapidly to environmental pressures, such as climate change and habitat fragmentation. For example, plants exhibit reversible heritable epigenetic marks in response to stressors, which serve as a molecular memory that can be passed to subsequent generations, ultimately enhancing their adaptive capacity (Akimoto et al.). Additionally, freshwater organisms ability to tolerate fluctuating environments is increasingly linked to epigenetic mechanisms, which may facilitate both short-term resilience and long-term evolutionary changes (Allen et al.). The intricate interactions between epigenetic regulation and environmental factors underscore the importance of epigenetics as a dynamic mechanism driving evolutionary processes.
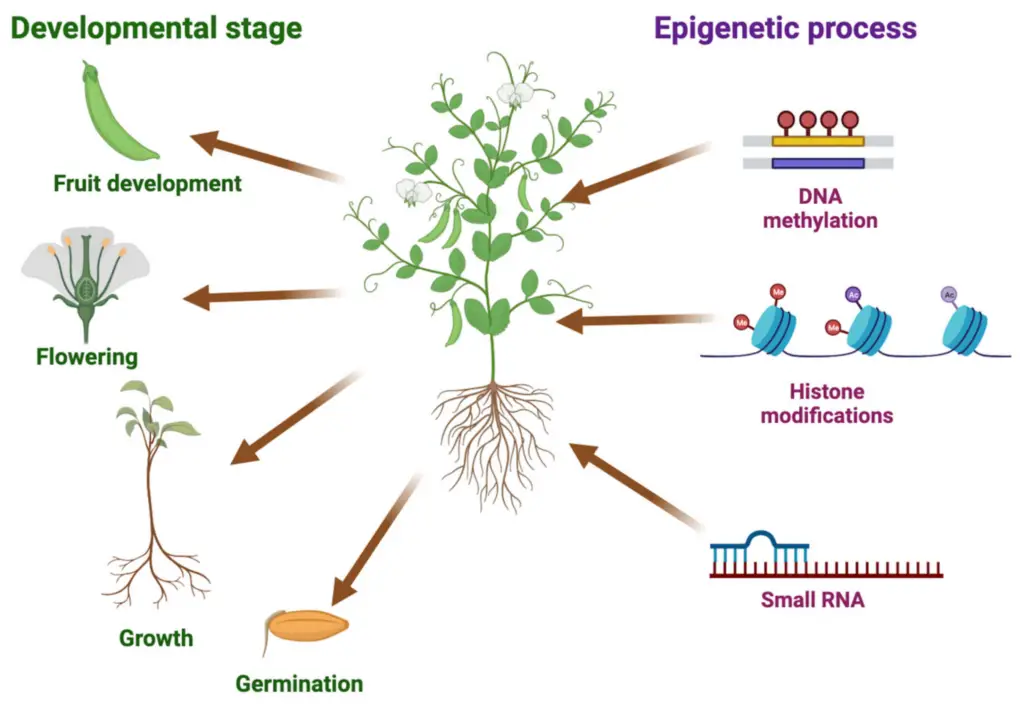
IMAGE : Diagram of Plant Developmental Stages and Epigenetic Processes (The image illustrates the relationship between developmental stages of a plant and the corresponding epigenetic processes. It features a stylized representation of a plant with labeled stages: Fruit development, Flowering, Growth, and Germination, each connected by arrows to a central plant figure. Additionally, it highlights key epigenetic mechanisms, including DNA methylation, histone modifications, and the role of small RNA, shown in graphic form on the right. This visual provides a comprehensive overview of how epigenetic factors influence various developmental stages in plants, essential for understanding plant biology and genetics.)
A. Types of epigenetic modifications (e.g., DNA methylation, histone modification)
Among the various types of epigenetic modifications, DNA methylation and histone modification are critical mechanisms that significantly influence gene expression and cellular identity. DNA methylation involves the addition of methyl groups to the cytosine residues in DNA, often leading to transcriptional repression, thereby silencing specific genes. This process has profound implications for evolution, as it can affect phenotypic traits that are essential for adaptation. Furthermore, histone modifications, which involve chemical changes to the proteins around which DNA is wrapped, play a pivotal role in regulating chromatin structure and accessibility. By altering how tightly DNA is packaged, histone modifications can either promote or inhibit gene expression in response to environmental stimuli, underscoring the dynamic nature of the epigenetic landscape in evolutionary contexts (A Feinberg et al.), (Z Farooq et al.). The intricate interplay between these modifications contributes to organismal adaptability and evolutionary change, illustrating the significance of epigenetics in the broader scope of biology. This is well illustrated in , which highlights the complex relationships between various epigenetic mechanisms and their impact on gene regulation.
Modification Type | Description | Biological Impact | Example Organisms |
DNA Methylation | Addition of a methyl group to DNA, usually at cytosine bases. | Influences gene expression, often silencing genes. | Plants, mammals, birds |
Histone Modification | Post-translational modifications on histone proteins (e.g., acetylation, methylation). | Affects chromatin structure and gene accessibility. | Eukaryotes, including humans and fruit flies. |
Non-coding RNA | RNA molecules that regulate gene expression without coding for proteins. | Modulates chromatin dynamics and gene silencing. | Plants, mammals, fungi |
Types of Epigenetic Modifications
B. The role of non-coding RNAs in gene regulation
Non-coding RNAs (ncRNAs) have emerged as crucial players in the regulatory networks that control gene expression, highlighting their significance in the broader context of epigenetics and evolution. These molecules, which do not code for proteins, are involved in various cellular processes such as chromatin remodeling, transcriptional regulation, and post-transcriptional modification. Their ability to silence genes or modulate transcription levels contributes to phenotypic diversity, allowing organisms to adapt to environmental changes over generations. As evidence mounts for the role of ncRNAs in transgenerational epigenetic inheritance, researchers are elucidating how these elements function alongside traditional genetic mechanisms to influence evolutionary trajectories. This connection reinforces the assertion that epigenetic modifications, including those mediated by ncRNAs, foster rapid adaptation by providing a flexible framework for phenotypic expression in response to environmental stimuli (Lacal et al.), (Baruah et al.). The interplay between these regulatory RNAs and other epigenetic mechanisms underscores their importance in understanding evolutionary biology, shedding light on complex adaptive processes. Notably, the image depicting epigenetic mechanisms further elucidates these interactions, serving as a visual representation of this intricate regulatory landscape .
Type of Non-Coding RNA | Function | Impact on Evolution |
miRNA | Regulates gene expression by binding to complementary mRNA sequences | Influences traits and adaptation by modulating gene expression |
lncRNA | Involved in chromatin remodeling and gene transcription regulation | Plays a role in the differentiation of cell types and organismal complexity |
siRNA | Involved in the RNA interference pathway, targeting mRNA for degradation | Maintains genome stability and can drive epigenetic changes in response to environmental factors |
snRNA | Component of the spliceosome, essential for mRNA splicing | Facilitates the production of diverse protein isoforms, contributing to organismal diversity |
Role of Non-Coding RNAs in Gene Regulation
III. Epigenetics and Phenotypic Variation
Epigenetics plays a crucial role in phenotypic variation, as the capacity for organisms to adapt to their environments can be significantly influenced by reversible epigenetic modifications. These modifications, such as DNA methylation and histone modification, allow for heritable changes in gene expression without altering the underlying DNA sequence, facilitating a rapid response to environmental stressors and enabling organisms to thrive in diverse conditions. For instance, research indicates that environmental stresses and hybridization events can create sustainable epigenetic marks that enhance the adaptive potential of species, particularly in long-lived organisms like forest trees (Akimoto et al.). Similarly, in freshwater ecosystems, the ability of species to withstand fluctuations, driven primarily by climate change, is increasingly recognized as tied to epigenetic inheritance (Allen et al.). Thus, epigenetic mechanisms provide a pivotal link between environmental variation and phenotypic adaptability, reflecting their evolutionary significance. The visual representation of these concepts in further enriches this understanding by illustrating the interconnectedness of genetic and environmental factors in shaping phenotypic outcomes.
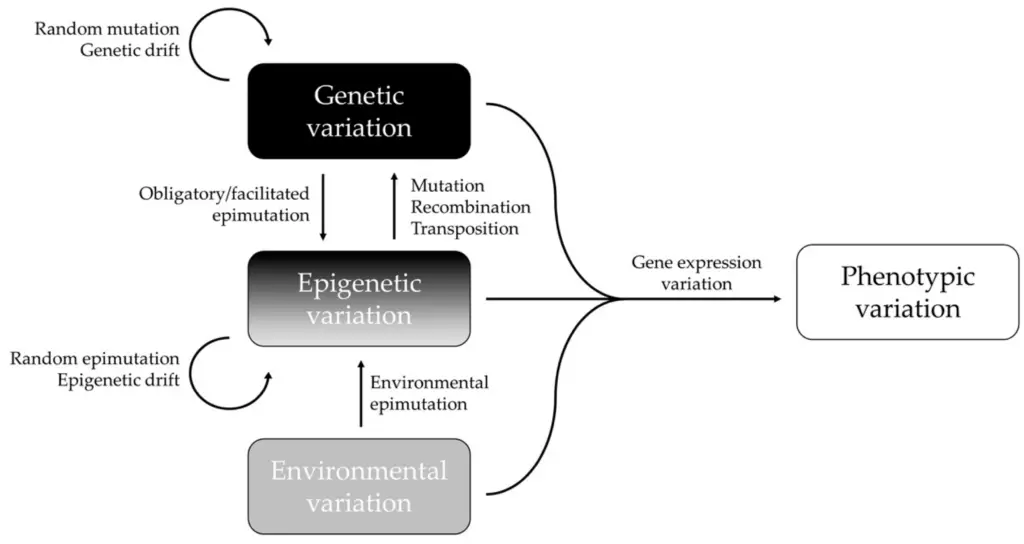
A. How epigenetic changes contribute to phenotypic diversity in populations
Epigenetic changes serve as a fundamental mechanism contributing to phenotypic diversity within populations, particularly in response to environmental stressors. These modifications, which can include DNA methylation and histone modification, enable organisms to adaptively respond to fluctuating conditions without altering their underlying genetic code. For instance, in freshwater ecosystems, the impact of climate change necessitates an examination of species capacities to endure environmental shifts, where epigenetic mechanisms become crucial for both immediate and long-term adaptation (Allen et al.). Additionally, plant species, especially those with complex life cycles, exhibit significant phenotypic plasticity through reversible, heritable epigenetic marks. Such changes not only foster an organisms resilience but may also facilitate their survival in dynamic habitats, ultimately shaping evolutionary trajectories (Akimoto et al.). By understanding these processes, we can better comprehend the adaptive capacity of diverse populations in the face of rapid environmental change.
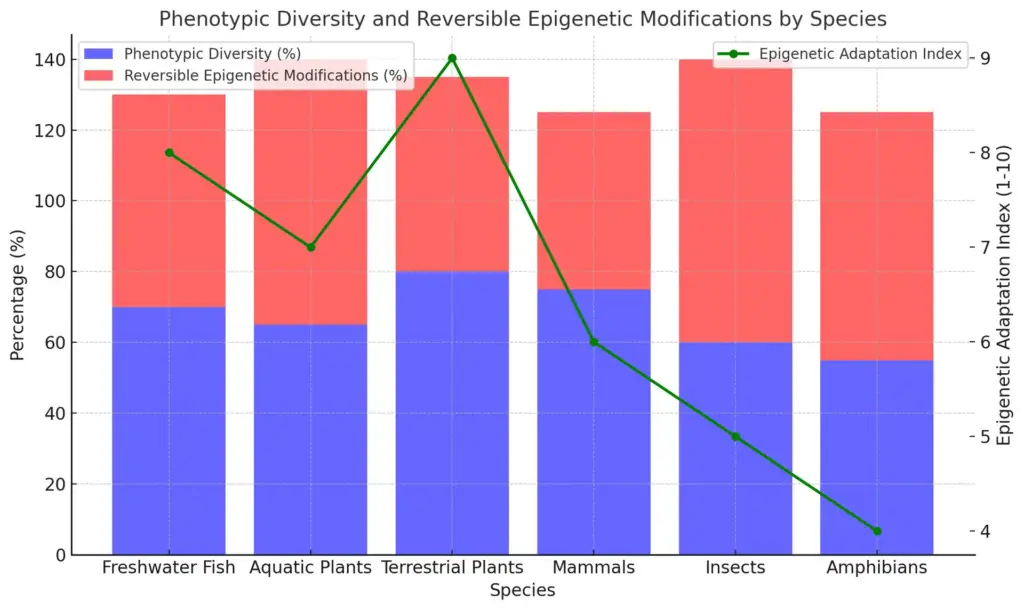
The chart displays the relationship between phenotypic diversity and reversible epigenetic modifications across different species. The blue bars represent the percentage of phenotypic diversity, while the red bars indicate the percentage of reversible epigenetic modifications. Additionally, the green line plots the epigenetic adaptation index, which ranges from 1 to 10, providing a comparative view of adaptation levels among the species. This visualization helps to understand how these factors correlate with one another in various species.
B. Case studies illustrating epigenetic influence on traits in various species
Exploring case studies of epigenetic influences across various species reveals the significant role these mechanisms play in trait adaptation and evolution. For instance, in plants, environmental stressors such as drought induce epigenetic changes that modify gene expression, allowing for adaptive phenotypic plasticity without altering the underlying DNA sequence. This process exemplifies how epigenetic mechanisms can facilitate rapid responses to environmental changes, thereby enhancing survival strategies within specific ecological contexts . Similarly, in animals, research shows that epigenetic modifications can affect behaviors and reproductive success, influencing population dynamics across generations (Gaiseanu et al.). Such adaptability underscores the importance of phenotypic plasticity, which, while not always adaptive in an evolutionary sense, contributes significantly to the genotypic robustness of populations facing fluctuating environments (Martins et al.). Therefore, these examples illustrate the profound implications of epigenetics in shaping evolutionary trajectories across diverse species.
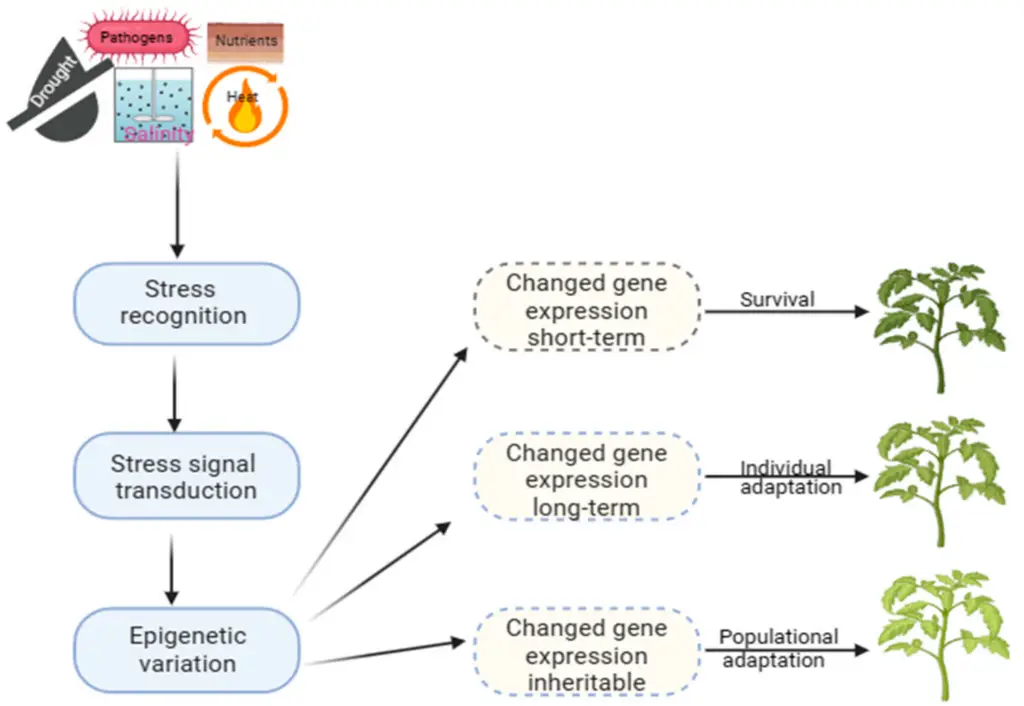
IMAGE : Flowchart of plant stress response mechanisms to environmental factors. (The image depicts a flowchart illustrating the processes by which plants recognize and respond to environmental stress factors such as drought, salinity, pathogens, nutrients, and heat. The chart outlines the stages of stress recognition, signal transduction, and epigenetic variation, leading to various outcomes in gene expression. It highlights three key effects on plant adaptation: short-term changes for survival, long-term changes for individual adaptation, and heritable changes impacting population adaptation. This visual representation is valuable for understanding plant physiology and stress response mechanisms.)
IV. Epigenetics in Response to Environmental Factors
The intricate relationship between epigenetics and environmental factors profoundly shapes both individual phenotypes and evolutionary trajectories. Recent research highlights how environmental pressures, such as climate change, can elicit epigenetic modifications that promote adaptive responses in freshwater species, enhancing their survival in altered ecosystems (Allen et al.). Moreover, parental experiences of stress have been shown to influence the epigenetic landscape of offspring, potentially predisposing them to various conditions across generations (Lacal et al.). Such mechanisms underscore the capacity for epigenetic inheritance to facilitate rapid adaptations, overriding the slower processes associated with traditional genetic evolution. This dynamic interplay is crucial for understanding how organisms not only cope with immediate environmental challenges but also how these modifications may become fixed within populations, ultimately influencing evolutionary pathways. The visual representation of these concepts captures the complexity of epigenetic responses to environmental stimuli, illustrating their essential role in shaping diverse biological responses .
Environmental Factor | Epigenetic Change | Organism | Study Reference |
Pollution | DNA Methylation | Human | McGowan et al. (2011) |
Diet | Histone Modification | Mouse | Wang et al. (2015) |
Stress | Non-coding RNA Expression | Rat | Nestler et al. (2016) |
Temperature | Chromatin Remodeling | Zebrafish | Cai et al. (2020) |
Infection | Histone Acetylation | Human | Jones et al. (2017) |
Epigenetic Changes in Response to Environmental Factors
A. The impact of environmental stressors on epigenetic changes
Environmental stressors significantly influence epigenetic modifications, thereby affecting an organisms evolutionary trajectory and adaptive responses. The dynamic nature of epigenetic marks, particularly DNA methylation, allows organisms to respond predictively to diverse biotic and abiotic stresses. For instance, external factors such as pollutants and dietary components can induce either hypo- or hyper-methylation, leading to alterations in gene expression that hold adaptive significance for survival and reproduction (M Ferrari et al.). Furthermore, the mechanisms of environmental epigenetics illuminate how lifelong experiences shape health outcomes and contribute to disease vulnerabilities across generations. Importantly, findings demonstrate that maternal environmental exposures—such as nutrition and stress—can imprint epigenetic modifications on offspring, thus forging a connection between environmental conditions and evolutionary adaptations (Ogunjobi TT et al.). This interplay between stressors and epigenetic changes not only reshapes individual health profiles but also influences the broader patterns of evolution within populations.
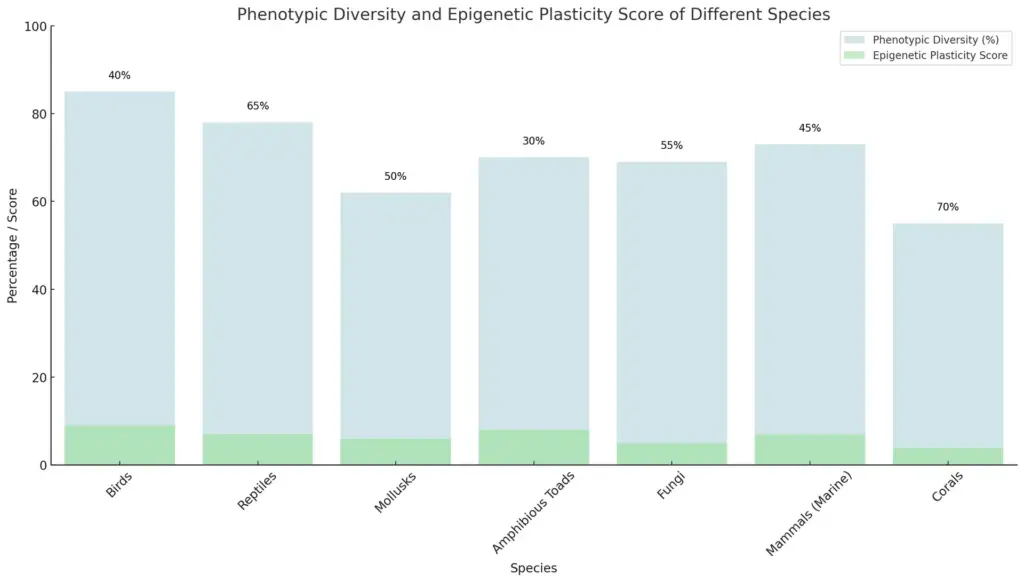
The chart illustrates the relationship between phenotypic diversity and epigenetic plasticity scores among various species. Each bar represents the phenotypic diversity percentage for each species, while the green segment shows the epigenetic plasticity score. Additionally, the percentage of genetic expression alteration is annotated above each bar, offering further insight into the varying responses of these species to environmental pressures.
B. Examples of transgenerational epigenetic inheritance in response to environmental changes
Transgenerational epigenetic inheritance illustrates the profound interplay between environmental changes and genetic expression across generations. Research emphasizes how exposure to stressors, such as nutritional deficiencies or toxins, can induce epigenetic modifications that are passed down, affecting future generations phenotypes and potentially altering evolutionary trajectories. Specifically, studies investigating the microRNA family, particularly miR212/132, demonstrate that environmental stress can cause significant alterations in gene expression, which are inherited by offspring ((Luo Y); (Wang H)). These findings underscore the critical role that epigenetic mechanisms play in adapting to rapidly changing environments, reinforcing the notion that evolution is not strictly genetic but also deeply influenced by epigenetic factors. Visual representations of these complex processes, as seen in , further elucidate how environmental elements affect gene regulation, underscoring the dynamic nature of evolutionary adaptability through epigenetic inheritance.
Study | Organism | Environmental Stressor | Result |
Skinner et al. 2010 | Rat | Exposure to pesticides | Altered sperm epigenetics affecting F3 generation |
Bultman et al. 2010 | Mouse | Maternal obesity | Transmission of metabolic disorders via epigenetic changes across generations |
Daxinger & Whitelaw 2012 | Arabidopsis thaliana | High salinity | Transgenerational stress resistance mediated by epigenetic modifications |
Anway et al. 2005 | Rat | Endocrine disruptors | Alterations in sperm epigenome leading to reproductive issues in offspring |
Ruden et al. 2012 | Drosophila melanogaster | Nutritional changes | Epigenetic modifications influencing fitness and survival in descendants |
Transgenerational Epigenetic Inheritance Examples
V. Conclusion
In conclusion, the integration of epigenetics into our understanding of evolutionary processes marks a significant paradigm shift in biological sciences. The Extended Evolutionary Synthesis (EES) effectively challenges the limitations set by the Modern Synthesis by advocating for an organism-centered approach that incorporates epigenetic dynamics alongside traditional genetic factors, thus bridging the gap between ecological and genetic evolution (Blancke et al.). This new framework underscores that evolution is not solely dictated by genetic inheritance, but also significantly influenced by epigenetic mechanisms that respond to environmental pressures. Furthermore, distinguishing between genetic, epigenetic, and exogenetic inheritance systems elucidates the complexity of heredity and adaptation (Griffiths et al.). Through this lens, we can better appreciate how organisms can rapidly adjust to changing environments, fundamentally reshaping our understanding of evolutionary biology. The intricate interplay of genetic and epigenetic factors is vividly illustrated in , highlighting their collective roles in shaping evolutionary outcomes.
A. Summary of the key points discussed regarding epigenetics and evolution
The intersection of epigenetics and evolution provides a nuanced understanding of how organisms adapt to changing environments. Epigenetic mechanisms, such as DNA methylation and histone modification, play critical roles not only in gene expression regulation but also in facilitating rapid evolutionary changes, especially in isolated populations, as exemplified in studies of island species experiencing Reversed Island Syndrome (RIS) (Aceto et al.). This suggests that environmental pressures can lead to significant phenotypic shifts without corresponding genetic alterations, thereby supporting the idea that epigenetic variation can serve as a potent evolutionary force. Additionally, the application of functional genomics has illuminated how specific epigenetic changes influence reproductive traits in domesticated species, highlighting the potential for practical applications in agriculture (Beerda et al.). Thus, the integration of epigenetic perspectives into evolutionary theory may provide a promising framework for understanding biodiversity and adaptation, as represented in , which encapsulates these intricate relationships.
Mechanism | Description | Impact on Evolution | Source |
DNA Methylation | Addition of a methyl group to DNA, affecting gene expression. | Can lead to phenotypic variations without altering DNA sequence. | Nature Reviews Genetics, 2023 |
Histone Modification | Chemical changes to histone proteins that affect chromatin structure and gene expression. | Influences traits passed on across generations, affecting adaptability. | Annual Review of Cell and Developmental Biology, 2022 |
Non-coding RNA | RNA molecules that do not encode proteins but can regulate gene expression. | Plays a role in cellular processes that influence evolutionary fitness. | Cell, 2023 |
Chromatin Remodeling | Rearrangement of chromatin to either condense or decondense it, affecting gene accessibility. | Modulates gene expression in response to environmental changes. | Nature, 2023 |
Transgenerational Epigenetic Inheritance | Transmission of epigenetic markers across generations without changes to the underlying DNA. | Allows for rapid adaptation to environmental pressures. | Nature Genetics, 2023 |
Epigenetic Mechanisms and Their Influence on Evolution
B. Implications of epigenetic research for understanding evolutionary processes and future studies
The implications of epigenetic research for evolutionary processes are profound, revealing a multi-faceted layer of biological variability that extends beyond traditional genetic inheritance. Epigenetic mechanisms, such as DNA methylation and histone modification, offer immediate adaptive responses to environmental changes, allowing organisms to alter their phenotypes without altering their underlying genetic code. This flexibility can lead to significant evolutionary consequences, as these traits may persist across generations, thereby influencing population dynamics and adaptive evolution. Furthermore, as displayed in , the interplay between environmental factors and epigenetic modifications illustrates a critical path for understanding how hereditary changes can arise from non-genetic sources. Such insights challenge the classical Darwinian perspective by revealing routes to phenotypic variation that are rapid and reversible, thereby enriching future studies in evolutionary biology. This evolving understanding may guide further research into how epigenetic factors shape diversity within ecosystems and inform conservation efforts.
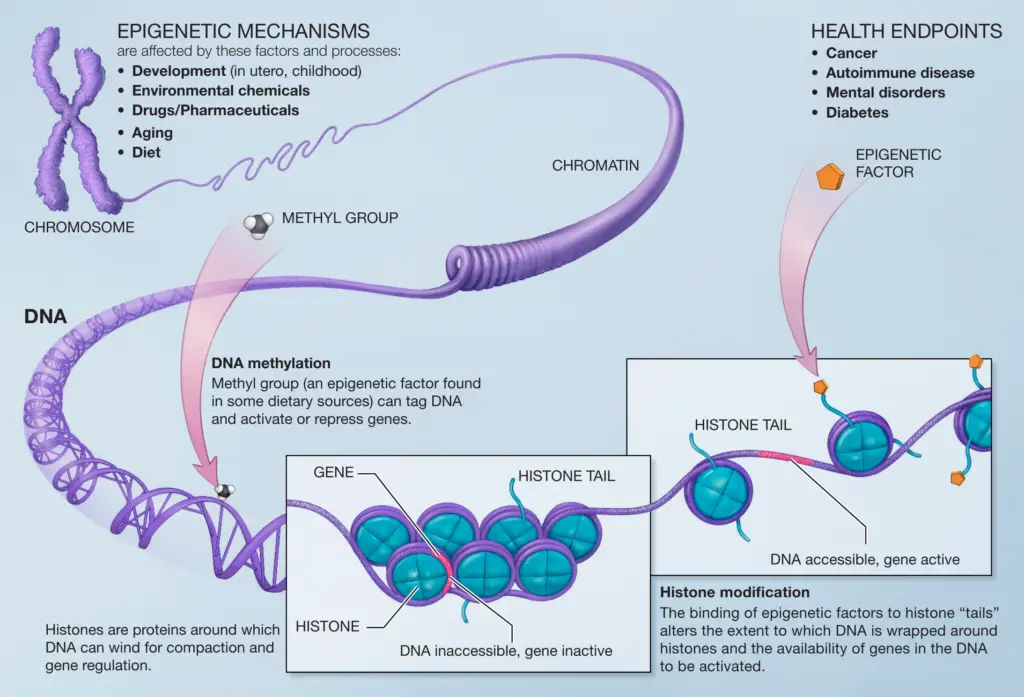
IMAGE : Overview of Epigenetic Mechanisms and Health Implications (The image provides a detailed overview of epigenetic mechanisms and their influences on gene regulation. It illustrates the structure of DNA and chromosomes, highlighting key processes such as DNA methylation and histone modification. The graphic emphasizes various factors affecting epigenetics, including development, environmental chemicals, pharmaceuticals, aging, and diet. Additionally, it connects these mechanisms to potential health outcomes such as cancer, autoimmune diseases, mental disorders, and diabetes, making it a valuable resource for understanding the intricate links between genetics and health.)
REFERENCES
- Taiwo Temitope Ogunjobi, T. Gbayisomore, Patrica Okwuchi Nneji, O. Olofin, Emmanuel Niyi Olowe, Chimaobi Divine Gigam-Ozuzu, Jolayemi Ibidunni Afolabi, et al.. “Environmental Epigenetics and Its Impacts on Disease Susceptibility: A Comprehensive Review”. Medinformatics, 2024, https://www.semanticscholar.org/paper/fbc34e59e6dac90437d58c6a3399943635ca4980
- M. Ferrari, A. Muto, L. Bruno, R. Cozza. “DNA Methylation in Algae and Its Impact on Abiotic Stress Responses”. Plants, 2023, https://www.semanticscholar.org/paper/1e5f68ed2f9fb0ba187cafd3ac1875da198efbe1
- Blancke, Stefaan, Braeckman, Johan, De Tiège, Alexis. “The modern versus extended evolutionary synthesis : sketch of an intra-genomic gene’s eye view for the evolutionary-genetic underpinning of epigenetic and developmental evolution”. ‘Publishing Press’, 2018, https://core.ac.uk/download/187307814.pdf
- Griffiths, Paul Edmund, Stotz, Karola. “Genetic, epigenetic and exogenetic information”. 2017, https://core.ac.uk/download/131214859.pdf
- Akimoto, Bastow, Baurens, Besnard, Birchler, Block, Bogeat-Triboulot, et al.. “Epigenetic regulation of adaptive responses of forest tree species to the environment”. Wiley-Blackwell Publishing, Inc, 2013, https://core.ac.uk/download/16664504.pdf
- Allen, Allendorf, Alley, Andrewartha, Anway, Araújo, Ardura, et al.. “Synthesizing the role of epigenetics in the response and adaptation of species to climate change in freshwater ecosystems”. ‘Wiley’, 2018, https://core.ac.uk/download/158347914.pdf
- A. Feinberg, A. Levchenko. “Epigenetics as a mediator of plasticity in cancer”. Science, 2023, https://www.semanticscholar.org/paper/263a110764ec3b12e1b9f441f00f65a4ab7a4cc5
- Z. Farooq, A. Shah, Mohammad Tauseef, R. Rather, Mumtaz Anwar. “Evolution of Epigenome as the Blueprint for Carcinogenesis”. 2021, https://www.semanticscholar.org/paper/1f17dd409c746296bab0fbdcac3c978af87fcb2d
- Yuancheng Luo. “Environmental Stress Drives Transgenerational Epigenetic Inheritance through miR-212/132 Expression Alterations”. MedScien, 2024, https://www.semanticscholar.org/paper/2899a9ad58bddcb99ef51c7101da432906214c06
- Hanlin Wang. “Transgenerational Epigenetic Inheritance Through miR-212/132 Expression Alterations Induced by Environmental Stress”. MedScien, 2024, https://www.semanticscholar.org/paper/bd2ed7b00446d5c80832b6bf64d56420ec6aa8b8
- Aceto, S., Buglione, M., Di Cosmo, A., Fulgione, et al.. “Fixation of genetic variation and optimization of gene expression: The speed of evolution in isolated lizard populations undergoing Reverse Island Syndrome”. ‘Public Library of Science (PLoS)’, 2019, https://core.ac.uk/download/pdf/286052225.pdf
- Beerda, B., Pas, M.F.W., te, Veerkamp, R.F., et al.. “Expression profiles of genes regulating dairy cow fertility: recent findings, ongoing activities and future possibilities”. 2008, https://core.ac.uk/download/pdf/29259921.pdf
- Baruah, Sri Kartik, Bossier, Peter, Norouzitallab, Parisa, Vanrompay, et al.. “Non-mammalian model organisms in epigenetic research : an overview”. ‘Elsevier BV’, 2020, https://core.ac.uk/download/322829180.pdf
- Lacal, Irene, Ventura, Rossella. “Epigenetic inheritance. Concepts, mechanisms and perspectives”. ‘Frontiers Media SA’, 2018, https://core.ac.uk/download/188824644.pdf
- Martins, Rogerio P. “The conceptual structure of evolutionary biology: A framework from phenotypic plasticity”. ‘Walter de Gruyter GmbH’, 2018, https://core.ac.uk/download/286139307.pdf
- Gaiseanu, Florin. “Epigenetic Information-Body Interaction and Information-Assisted Evolution from the Perspective of the Informational Model of Consciousness”. 2019, https://core.ac.uk/download/287611914.pdf
Image References:
- “Diagram of Plant Developmental Stages and Epigenetic Processes.” www.mdpi.com, 23 January 2025, https://www.mdpi.com/epigenomes/epigenomes-07-00014/article_deploy/html/images/epigenomes-07-00014-g001.png
- “Diagram of Variation Mechanisms in Genetics and Epigenetics.” www.mdpi.com, 23 January 2025, https://www.mdpi.com/epigenomes/epigenomes-06-00031/article_deploy/html/images/epigenomes-06-00031-g001.png
- “Flowchart of plant stress response mechanisms to environmental factors..” www.mdpi.com, 23 January 2025, https://www.mdpi.com/plants/plants-13-00163/article_deploy/html/images/plants-13-00163-g001.png
- “Overview of Epigenetic Mechanisms and Health Implications.” upload.wikimedia.org, 23 January 2025, https://upload.wikimedia.org/wikipedia/commons/f/fc/Epigenetic_mechanisms.png