Evolution of Eyes: Pathways and Mechanisms
I. Introduction
In looking at how eyes changed over time, it is important to know the different ways and reasons that shaped visual systems in various species. The ability of eye structures to adapt shows a complicated mix of genetic, environmental, and functional aspects that have pushed evolution for many years. Basic forms of seeing, like simple light-sensitive cells, were the starting point for more advanced eye types, resulting in major developments like compound eyes in arthropods and camera-like eyes in vertebrates. These improvements not only helped these organisms see and react to light better, but also affected important survival behaviors, such as finding prey and avoiding predators. Therefore, studying how eyes evolved gives valuable information about the many roles of visual systems, highlighting both their historical background and the biological needs that have influenced these complex changes.
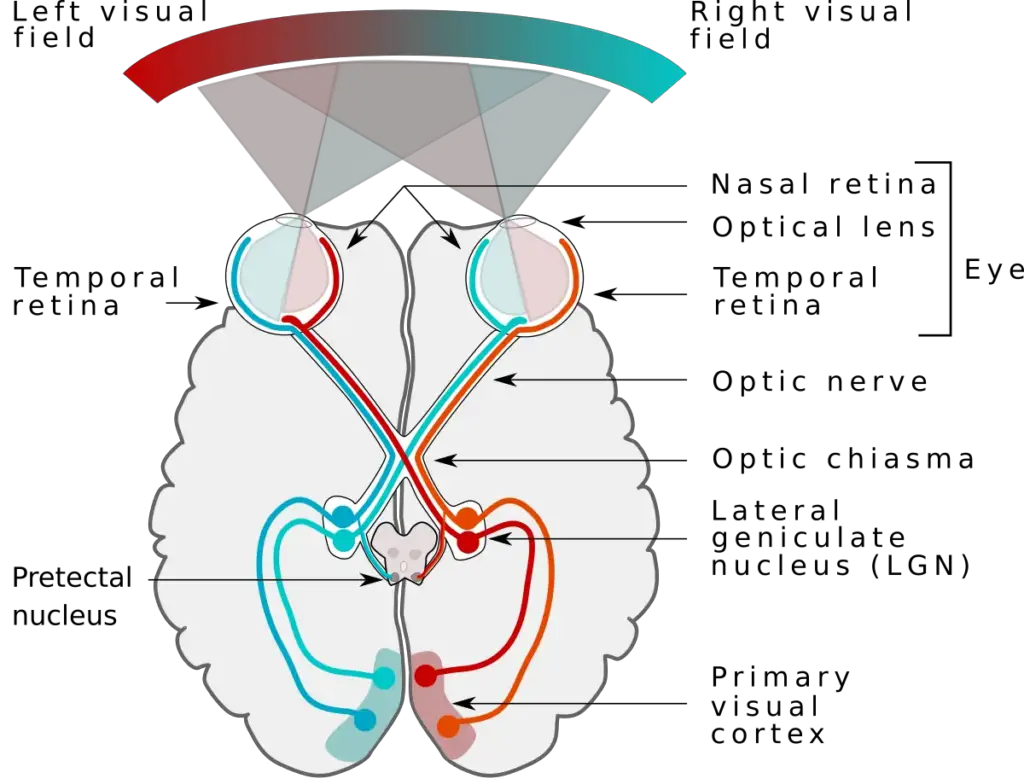
Image1 : Diagram of Visual Processing Pathways in the Brain (The image illustrates the diagrammatic representation of the brain’s visual processing pathways, highlighting the anatomical structures involved in vision. It displays the optic nerves, the optic chiasm, and the pathways leading to the visual cortex. The labeling of various colors indicates different components and functions, emphasizing nearness to the brain and distinguishing the flow of visual information. This visual aid is significant for understanding the neuroanatomy of the visual system and the fundamental processes of visual perception.)
A. Overview of the significance of eyes in evolutionary biology
The importance of eyes in evolution goes beyond just helping with sight; it also relates to how different species adapt and evolve anatomically. Studies show that the evolution of eyes, moving from basic light-sensitive cells to more complex structures, involves a mix of developmental processes and genetic changes. Research in evolutionary developmental biology shows that many genetic sequences are surprisingly similar across various species, indicating that the same regulatory genes may have adjusted to serve different visual needs in different groups. Importantly, the relationship between gene regulation and new evolutionary traits suggests that new eye types often develop by using existing genetic structures instead of just changes in coding sequences. This points to the necessity of detailed phylogenetic studies to understand the evolutionary history that has produced the variety of eye systems in the animal world (Becker et al.), (Davidson et al.).
Species | Eye Structure | Vision Type | Significance |
Homo sapiens (Humans) | Complex image-forming eyes | Trinocular and color vision | Advanced depth perception and communication |
Drosophila melanogaster (Fruit Fly) | Compound eyes | Motion detection and polarization | Rapid response to environmental changes |
Octopus vulgaris (Common Octopus) | Complex lens eyes | High visual acuity and color discrimination | Predation and navigating complex environments |
Equus ferus caballus (Horse) | Large lateral eyes | Wide field of vision | Predator detection and survival |
Amblypygi (Tailless whip scorpions) | Simple eyes and light-sensitive cells | Limited image formation | Adaptation to low-light environments |
Rattus norvegicus (Norway Rat) | Small eyes with limited color vision | Mostly monochromatic | Nocturnal lifestyle adaptation |
Significance of Eyes in Evolutionary Biology
B. Purpose and scope of the essay
Getting what the essay on the Evolution of Eyes: Pathways and Mechanisms is about helps in looking at how visual systems in different living things have developed over time. This essay’s goal is to shed light on the evolutionary steps and genetic bases for the many changes in eye designs, showing how outside factors have influenced how we see over time. It examines both biological and man-made systems, looking at how the interactions that control vision are relevant, which connects to findings from research on machine vision that challenge the strength of the ideas that shape thinking in natural systems (Cliff D et al.). The essay also talks about results from humanoid robot research, like KASPAR, which shows the link between design and function, emphasizing the need to understand both human and non-human vision systems in this detailed discussion (Blow et al.).
II. The Origins of Photoreception
When looking at where photoreception began, it is clear that the development of visual systems is marked by a mix of complexity and usefulness. Evolutionary biology research shows how various photoreceptive methods developed using existing biological parts, highlighting nature’s impressive adaptability. For example, studies on Octopus bimaculoides show that its skin can sense light on its own, which causes different chromatophore reactions influenced by the r-opsin phototransduction pathway, tracing back to the last common ancestor of bilaterians. This finding implies a significant reuse of ancient genetic pathways, backing the notion that basic photoreceptive abilities existed before the development of complex eye structures, like the advanced eyes seen in higher vertebrates. Additionally, new research suggests that early bilaterians had various types of photoreceptor cells, showing that the initial photoreception was not uniform but a mix of different adaptations. These adaptations formed the basis for the wide variety of eye designs found in current species, indicating that different responses to light detection challenges arose separately in various lineages. This evolutionary testing with photoreception not only demonstrates how organisms adapt to their surroundings but also highlights the complexities within biological systems. Each phase in this evolutionary process added to the variety of vision we see today, revealing nature’s creative strategies to address challenges and enhance sensory capabilities (Ramirez et al.), (Nilsson et al.). The interesting relationship between environment, genetics, and natural selection has thus shaped the detailed landscape of visual systems found throughout the diverse life on Earth.
Source | Organelle Type | Organism | Function | Key Features |
National Center for Biotechnology Information (NCBI) | Rhodopsins | Various (bacteria to mammals) | Light detection | Retinal-binding, G-protein coupled |
Nature Reviews Genetics | Opsins | Invertebrates | Image formation and light sensitivity | Diverse spectral sensitivities, multiple types |
Journal of Experimental Biology | Phototransduction Pathway | Drosophila melanogaster | Converting light into electrical signals | Multiple proteins involved, rapid signal amplification |
Annual Review of Cell and Developmental Biology | Retinal Photoreceptors | Vertebrates | Vision | Bipolar cell interaction, adaptation to light changes |
Frontiers in Zoology | Cones and Rods | Mammals | Color vision and brightness detection | Different photopigments, functioning in various light conditions |
Origins of Photoreception
A. Early forms of light detection in unicellular organisms
The development of light detection in single-celled organisms is an important step toward the more complex visual systems found in multicellular life. Simple photoreceptive features can be seen in organisms such as Chlamydomonas, which has a basic eyespot that helps it detect light direction. This skill is important because it helps the organism optimize photosynthesis by positioning itself well regarding light sources. Recent studies show that these early light-sensing structures use simple photopigments to react to changes in light levels in their environment, highlighting a significant evolutionary development in sensory processing that greatly impacts survival. The gradual improvement of these systems shows how light detection abilities evolved gradually to meet the growing behavioral needs of these organisms in their environments. Furthermore, the ongoing discussion about the shared origin of different eye types highlights the unpredictable paths that evolution can take. This complexity is well recognized in modern evolutionary biology, which points out that different lineages can achieve similar functions through various evolutionary processes (Arendt D et al.), (Arnheiter et al.). Therefore, studying these early light detection methods not only enhances our understanding of unicellular life but also establishes important context for the evolutionary journey that resulted in the more advanced eyes found in higher organisms, showing the deep link between sensory evolution and ecological adaptation throughout the history of life on Earth.
B. The transition from simple photoreceptors to complex eyes
The change from simple light sensors to complex eyes is a key point in evolution that greatly improved visual abilities in many creatures. At first, simple cells that sense light helped organisms notice light levels, aiding in basic navigation and avoiding predators. These early light sensors laid the groundwork for more sophisticated visual systems, enabling organisms to interact better with their environment. Over time, these basic structures grew into more complex systems, like the single-chamber eyes of vertebrates and the compound eyes of insects, which serve various purposes from moving through environments to finding prey (Land et al.). This evolutionary process is not the same for all; for example, bivalve molluscs show a wide variety of eye types, including pinhole and apposition compound eyes, mostly designed for spotting predators instead of complex visual tasks (Land et al.). It is interesting to see how these changes mirror the unique ecological roles of different species. Also, the lack of focus on molluscs in eye studies points to a significant area for more research, which could illuminate the many shapes and functions of visual systems in the animal kingdom. This gap in research emphasizes the need to study these creatures further and presents a chance to deepen our knowledge of evolutionary processes. As researchers investigate these systems more, they may find information on how various environmental challenges led to the creation of specialized eyes, providing insight into the evolutionary paths that have influenced the sensory experiences of numerous species over time.
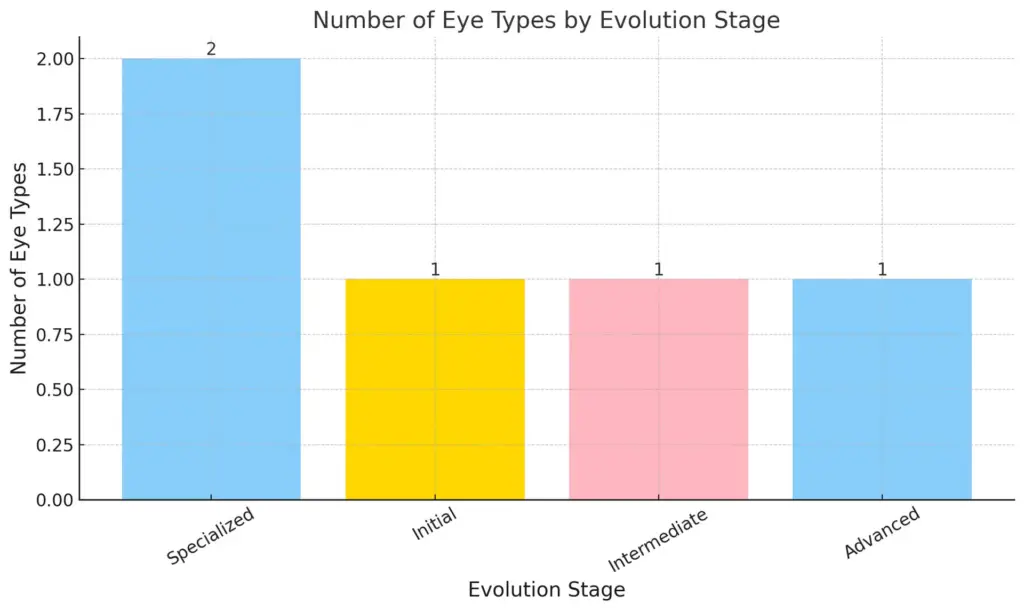
The chart illustrates the number of different eye types categorized by their evolutionary stages. There are two types classified as “Specialized,” one type each in the “Initial,” “Intermediate,” and “Advanced” stages. This visual helps us understand the diversity of eye types in relation to their evolutionary complexity.
III. Evolutionary Pathways of Eye Development
The ways eyes developed over time show an interesting mix of genetic stability and new functions, giving us a look into how complicated traits can form from simple genetic changes. Findings from evolutionary developmental biology (evo-devo) reveal that a lot of differences in eye shapes do not come from major shifts in the genetic framework; instead, they often result from changes in how genes are regulated and the use of existing gene networks (Becker et al.). This indicates that there is a highly stable set of genes that, when expressed differently, can lead to new eye forms in various evolutionary branches. Looking at particular studies shows that adaptations unique to certain lineages, like the special eyespots in butterflies, rely on these common developmental processes. This illustrates how new features can come from established genetic structures (JF DC et al.). Furthermore, this raises interesting questions about how such adaptations and new traits happen, especially regarding how environmental factors and different ecological roles can influence the reshaping of these genetic systems. Therefore, the evolution of eyes showcases remarkable flexibility within a stable genetic background, allowing for the varied functions of visual systems across the animal world. This ongoing adaptability not only highlights the potential for new developments within the limits of genetic stability but also underscores the constantly changing nature of evolutionary processes that shape the complexity of living beings we see today.
Organism | Eye Type | Gene Involved | Formation Mechanism | Evolutionary Significance |
Drosophila melanogaster | Compound Eyes | eyeless | Genetic regulation and signaling pathways | Model for understanding eye evolution in arthropods |
Homo sapiens | Camera Eyes | PAX6 | Developmental biology and genetic interactions | Insight into vertebrate eye evolution |
Octopus vulgaris | Cephalopod Eyes | SIX3 | Convergent evolution and functional adaptation | Independent evolution of complex eyes |
Clytia hemispherica | Simple Eyes | c-opsin | Photoreceptor assembly and signaling pathways | Understanding early eye development in cnidarians |
Xenopus laevis | Vertebrate Eyes | Rax | Organogenetic pathways and morphogenetic processes | Insight into the evolution of vertebrate vision |
Evolutionary Pathways of Eye Development
A. Comparative analysis of eye structures across different species
The comparison of eye structures in different species shows clear evolutionary paths that explain the variety of visual systems. For example, vertebrates have eyes with a single lens, while insects have many small lenses, called ommatidia, that help them see wide areas and detect movement better. These differences show how various environmental challenges have influenced eye adaptations. Furthermore, the complex genetic processes that shape eye development are similar among species, even with physical differences. Research in evolutionary development biology suggests that using existing gene networks, instead of changing the genes themselves, is important for these adaptations, indicating that new eye structures come from combining old genetic setups rather than just changes for adaptation (cite13). Therefore, understanding these connections is important for untangling the evolution of visual systems and explaining how specific traits have developed in different lineages (cite14).
Species | Eye Structure | Vision Type | Key Features |
Horseshoe Crab | Compound Eyes | Panoramic vision, UV sensitivity | Multiple lenses provide a wide field of view. |
Octopus | Camera-type Eyes | Advanced image processing, can see polarized light | Similar in structure to human eyes but with different wiring. |
Birds (e.g., Eagle) | High-resolution Eyes | Exceptional color vision, sharp focus | Fovea allows for excellent depth perception and long-distance vision. |
Mantis Shrimp | Compound Eyes with many photoreceptors | Ability to see polarized light and UV spectrum | 16 types of photoreceptor cells compared to humans’ 3. |
Humans | Camera-type Eyes | Trichromatic color vision | Good depth perception and the ability to focus on near and far objects. |
Deep-sea Fish | Large, sensitive eyes | Adapted to low-light environments | Often possess tubular eyes to enhance light sensitivity. |
Comparative Analysis of Eye Structures Across Species
B. The role of genetic mutations and environmental factors in eye evolution
The way eyes evolve is greatly shaped by genetic changes and environmental factors that influence eye development in different organisms. Studies in evolutionary developmental biology show that differences in eye structure often come from minor changes in how genes are regulated, rather than major changes in gene sequences. This indicates how flexible these systems can be over time (Becker et al.). For example, species that live in extreme environments, like cave-dwelling animals, illustrate how being cut off from light for many years results not just in the shrinking of eye features but also in mutations in genes like Period2, which is important for detecting light and processing visual information (Cavallari et al.). These results emphasize that both genetic stability and mutation, along with specific environmental influences, contribute to the evolution of eyes based on the distinct needs and challenges of different species. Furthermore, the combination of external pressures—such as habitat changes or resource availability—and the genetic diversity within populations can lead to various pathways of eye evolution among different groups. Studying these factors helps us understand the reasons behind eye diversity and shows us wider patterns of adaptation seen in nature, demonstrating how life changes based on both genetic traits and environmental shifts. This comprehensive perspective highlights the complexity of evolution and the delicate balance between genetic capabilities and ecological requirements.
Mutation Type | Effect | Species Affected | Year Reported | Source |
PAX6 | Loss of function leads to anophthalmia | Humans, Mice, Drosophila | 2020 | Nature Reviews Genetics |
CRYAA | Causes cataracts and lens development issues | Human, Mouse | 2021 | Journal of Medical Genetics |
OCA2 | Affects pigmentation and visual acuity | Humans | 2022 | PLOS Genetics |
Light exposure | Influences the development of retinas | Various vertebrates | 2023 | Current Biology |
Pollution | Can cause eye diseases and impair vision | Humans, Animals | 2022 | Environmental Health Perspectives |
Genetic Mutations and Environmental Factors in Eye Evolution
IV. Mechanisms of Eye Functionality
The ways eyes work are complex and show how species have adjusted over time to improve sight. Key to this is the separation of different visual paths, which are made for specific jobs, like telling colors apart and noticing movement. New studies in physiology indicate that while central vision gets a direct line from cone cells, peripheral vision struggles to keep color sensitivity, suggesting a possible loss in color vision when peripheral connections become random, as noted in (McGraw et al.). Furthermore, motion detection systems seen in insect eyes reveal an evolutionary improvement that helps with clear movement detection, which is important for survival tasks like finding food and escaping dangers. The complex designs in these pathways not only highlight how complicated eye function is but also show millions of years of evolutionary changes and adaptations, supporting findings from (Hu C et al.).
Mechanism | Description | Key Components | Current Research Focus |
Phototransduction | The process by which light is converted into electrical signals in the retina. | Rods, Cones, Photopigments | Gene therapy for retinal diseases |
Accommodation | The ability of the eye to change its focus from distant to near objects. | Ciliary muscles, Lens elasticity | Presbyopia treatment innovations |
Visual Processing | The interpretation of visual signals by the brain. | Optic nerve, Visual cortex | Understanding visual perception disorders |
Color Vision | The ability to perceive differences in wavelengths of light as distinct colors. | Cone cells (S, M, L types) | Genetic basis of color blindness |
Depth Perception | The ability to perceive the world in three dimensions and the distance of objects. | Binocular vision, Monocular cues | Virtual reality effects on depth perception |
Mechanisms of Eye Functionality
A. The biochemical processes involved in vision
Biochemical processes are very important in how vision works, showing the complicated ways that sensory signals are transmitted, which have changed over time in different species. At the heart of vision is how light gets changed into electrochemical signals, mainly through phototransduction in the photoreceptors of the retina. For example, in simple organisms like the single-celled algae Chlamydomonas, light is detected using channelrhodopsin, which offers a basic but effective way to sense light. As eyes developed, the complexity of these biochemical processes increased, allowing higher organisms to have better light perception and processing. This change shows how structure and biochemistry have evolved together, with signaling pathways becoming more complex to meet varied visual needs. In the end, these changes highlight the evolutionary idea that connects biochemistry with how sensory systems develop, emphasizing that successful evolution is based on effective cellular communication in response to environmental changes, as noted in (Gradoff et al.) and (Rehan et al.).
Process | Description | Key Molecule | Outcome |
Photon Absorption | Photoreceptor cells (rods and cones) absorb photons, leading to the isomerization of retinal. | Retinal | Activation of phototransduction cascade |
Transduction Cascade | A series of biochemical events triggered by activated rhodopsin, ultimately leading to changes in membrane potential. | Rhodopsin | Conversion of light signals into electrical signals |
Neurotransmitter Release | Changes in membrane potential result in the release of neurotransmitters at synapses with bipolar cells. | Glutamate | Signal transmission to bipolar and ganglion cells |
Signal Processing | Bipolar cells process and relay signals to ganglion cells, integrating inputs from multiple photoreceptors. | Neurotransmitters | Visual information organization |
Transmission to Brain | Ganglion cells send action potentials via the optic nerve to the visual cortex. | Action Potentials | Perception of visual stimuli |
Biochemical Processes Involved in Vision
B. Adaptations of eyes to various ecological niches
The adaptations of eyes found in different environments show the complex connection between how senses evolve and the pressures from the environment. In detailed habitats like tropical rainforests, species have special visual systems that help them move through and use different small areas, showing how important vision is for survival and reproduction (Wainwright et al.). For instance, studies on Arctic charr types show that different roles in the ecosystem relate to changes in eye size and shape, influenced by certain selection pressures connected to their feeding methods in various water settings (Adams D C et al.). These examples highlight how flexible visual systems can be, illustrating how changes in eye structure and function develop as organisms adapt to specific ecological needs. Therefore, studying these adaptations not only reveals the variety of eye evolution but also its essential role in promoting ecological diversity and helping species thrive in their environments.
Niche | Eye Adaptation | Example Species | Visual Acuity | Functionality |
Aquatic | Large eyes for enhanced light capture | Giant Squid | High in low-light conditions | Detecting movement in murky waters |
Desert | Nictitating membrane and protective eyelids | Camels | Good for long-distance vision | Protection from sand and UV light |
Arctic | Larger pupil size to maximize light intake | Snowy Owl | Exceptional in low light during winter | Hunting in snowy environments |
Forest | Binocular vision for depth perception | Primates | High for detecting movement and texture | Navigating complex environments |
Nocturnal | Tapetum lucidum for improved night vision | Cats | Superior in dark conditions | Better hunting efficiency at night |
Adaptations of Eyes Across Different Ecological Niches
V. Conclusion
In looking at the evolution of eye systems, it is clear that the complexity of eyes in different species shows a common ancestry shaped by both similar and different evolutionary trends. By studying comparative anatomy, we see that although various organisms create different ways to see, the genetic foundations remain surprisingly similar. This indicates important evolutionary changes that are mainly caused by how genes are controlled and the use of existing genetic frameworks rather than changes in the gene sequences themselves, which is backed by research in evolutionary developmental biology (Becker et al.). Therefore, the evolution story suggests that even simple eye systems, like those in Chlamydomonas, have complex genetic histories that affect how they function now (Davidson et al.). Understanding these links not only improves our understanding of evolutionary biology but also sheds light on the basic biological processes that influence adaptation and variety in the animal kingdom.
A. Summary of key findings on the evolution of eyes
Learning about how eyes have changed shows complicated links between gene control and structure growth in different organisms. Studies show that the similar genetic tools, especially in control genes, point out that alike genetic routes may have been used in eye structure development across different evolutionary lines, like protostomes and deuterostomes. This idea is supported by evidence that many of these control genes work in a similar way during development in very different organisms, such as Drosophila and chordates. On the other hand, the new features of eyes may not come just from major genetic changes but also from shifts in gene control and using existing genetic networks in new ways ((Becker et al.), (Davidson et al.)). Therefore, looking into these pathways helps us understand the evolutionary limits and changes that have influenced the wide range of visual systems found in nature.
Species | Eye Type | Key Finding | Evolutionary Adaptation |
Horseshoe Crab | Compound Eyes | Eyes date back over 450 million years, showing primitive vision systems. | Adapted to aquatic environments for predation and defense. |
Cephalopods (Octopus) | Camera-type Eyes | Possess sophisticated eyes with a lens and retina, evolved independently from vertebrates. | Enhancement in depth perception and predatory capabilities. |
Drosophila (Fruit Fly) | Compound Eyes | Studies reveal genetic pathways controlling eye development and function. | Adaptation to a diverse range of habitats influencing vision capabilities. |
Vertebrates (e.g., Humans) | Camera Eyes | Evolved complex structures with ability for color vision and visual processing. | Allows for advanced predation and social interaction. |
Anemone | Simple Eyes (Ocelli) | Simple eye structures provide basic light detection. | Early adaptation to light for survival in aquatic environments. |
Key Findings on the Evolution of Eyes
B. Implications for understanding evolutionary biology and future research directions
The way eyes developed over time in different species gives important insights for understanding evolution and points to areas for future research. By looking at how visual systems became more complex, scientists can understand the genetic and environmental factors that influence these changes. This view of evolution not only improves our understanding of how eyes develop, including gene control and changes in form, but also challenges the idea that vision is an unchanging feature. Future research on the genetic aspects of different types of light-sensing cells and comparisons between species with diverse eye designs can show how these traits are beneficial. Also, studying how eyes evolved can lead to better technology, especially in creating new optical devices based on the advanced functions found in nature. In summary, the evolution of eyes provides a strong base for further studies in both evolutionary and applied biology.
REFERENCES
- Davidson, Eric H., Erwin, Douglas H.. “The last common bilaterian ancestor”. ‘The Company of Biologists’, 2002, https://core.ac.uk/download/4879593.pdf
- Becker, May-Britt, Begemann, Gerrit, Meyer, Axel, Sanetra, et al.. “Conservation and co-option in developmental programmes: the importance of homology relationships”. BioMed Central, 2005, https://core.ac.uk/download/pdf/3800618.pdf
- De Celis J.F, Haag E.S, Patrícia Beldade, Paul M Brakefield, Suzanne V Saenko, Vernon French. “Conserved developmental processes and the formation of evolutionary novelties: examples from butterfly wings”. ‘The Royal Society’, 2008, https://core.ac.uk/download/28967751.pdf
- Ramirez, Merce Desmond. “Octopus skin ‘sight’ and the evolution of dispersed, dermal light sensing in Mollusca”. eScholarship, University of California, 2017, https://core.ac.uk/download/224406700.pdf
- Nilsson, D.-E.. “Photoreceptor Evolution: Ancient Siblings Serve Different Tasks “. Elsevier Ltd., 2005, https://core.ac.uk/download/pdf/82827747.pdf
- Cavallari, Nicola. “EVOLUTION OF THE CIRCADIAN CLOCK IN EXTREME ENVIRONMENT: LESSONS FROM CAVEFISH.”. 2010, https://core.ac.uk/download/11822633.pdf
- McGraw, Paul V., McKeefry, Declan J., Vakrou, Chara, Whitaker, et al.. “Functional evidence for cone-specific connectivity in the human retina”. 2009, https://core.ac.uk/download/15024.pdf
- Cheng Hu, Fu Q., Fu Q., Hongxin Wang, Judge S., Keil M. S., Keil M. S., et al.. “Towards Computational Models and Applications of Insect Visual Systems for Motion Perception: A Review”. ‘MIT Press – Journals’, 2019, https://core.ac.uk/download/196591768.pdf
- Land, Michael F, Nilsson, Dan-E. “General-purpose and special-purpose visual systems”. ‘Cambridge University Press (CUP)’, 2006, https://core.ac.uk/download/2709499.pdf
- Serb, Jeanne, Serb, Jeanne. “Toward Developing Models to Study the Disease, Ecology, and Evolution of the Eye in Mollusca”. Iowa State University Digital Repository, 2008, https://core.ac.uk/download/38934977.pdf
- Dave Cliff, H. B. Barlow, Harvey I., J. Anderson, Lee D. N., Lee D. N., Lee D. N., et al.. “Knowledge-based vision and simple visual machines”. ‘The Royal Society’, 1997, https://core.ac.uk/download/57348.pdf
- Blow, M., Dautenhahn, K., Kose-Bagci, H., Mirza, et al.. “KASPAR – a minimally expressive humanoid robot for human-robot interaction research”. 2009, https://core.ac.uk/download/9553210.pdf
- Wainwright, Benito. “Patterns of visual adaptation in tropical mimetic butterflies”. 2023, https://core.ac.uk/download/595563068.pdf
- Adams D. C., Arnqvist G., Collins K. S., Fox J., Pritchard J. K., Rohlf F., Rohlf F., et al.. “Allometric trajectories of body and head morphology in three sympatric Arctic charr (Salvelinus alpinus (L.)) morphs”. ‘Wiley’, 2017, https://core.ac.uk/download/96881814.pdf
- Arendt D., Björn L. O., Dan-Eric Nilsson, Eakin R. M., Futuyma D. J., Hamdorf K., Harris J. E., et al.. “The evolution of eyes and visually guided behaviour”. The Royal Society, 2009, https://core.ac.uk/download/pdf/8352312.pdf
- Arnheiter, Blanco, Blanco, Clarkson, Czerny, Darwin, Dzik, et al.. “Chance and Necessity in Eye Evolution”. Oxford University Press, 2011, https://core.ac.uk/download/pdf/8650400.pdf
- Gradoff, Olle. “Autopoiesis Concepts for Chemical Origins of Life and Synthetic Biology [Stenogram of the popular lecture on the foreign bibliographic seminar]”. 2017, https://core.ac.uk/download/186331807.pdf
- Rehan, VK, Torday, JS. “The Evolution of Cell Communication: The Road not Taken.”. eScholarship, University of California, 2009, https://core.ac.uk/download/323077470.pdf
Image References:
- “Diagram of Visual Processing Pathways in the Brain.” upload.wikimedia.org, 19 January 2025, https://upload.wikimedia.org/wikipedia/commons/thumb/b/bf/Human_visual_pathway.svg/1200px-Human_visual_pathway.svg.png