Extremophiles: How Bacteria Survive in Hot Springs, Deep Oceans, and Outer Space
Table of Contents
I. Introduction to Extremophiles
Extremophiles, a unique group of microorganisms, exemplify the incredible resilience and remarkable adaptability of life in some of the harshest environments on Earth and beyond. These extraordinary organisms have evolved over millions of years to thrive in conditions that would be inhospitable to the vast majority of life forms, including extreme temperatures that can reach scorching highs or frigid lows, salinity levels that rival the saltiest bodies of water, acidity that can corrode metals, and intense pressures found in inaccessible locations such as hot springs, deep-sea hydrothermal vents, and polar ice caps. The significance of extremophiles extends far beyond their specific ecological niches; they provide critical insights into the limits of life and raise fascinating questions about the potential for extraterrestrial organisms existing in similar extreme conditions elsewhere in the universe. The diversity of extremophiles is illustrated in several studies and classifications, which categorize the various environments where these resilient organisms can be found, thus highlighting their remarkable adaptability and the evolutionary strategies they employ to survive. As scientists continue to study extremophiles, they not only enhance our understanding of the incredible variety of life on Earth but also broaden the horizons for astrobiology, suggesting compellingly that life could potentially thrive in the most unexpected and inhospitable places, such as the surface of Mars or beneath the icy crust of Europa. These discoveries fuel our curiosity about the universe and challenge our preconceived notions of what constitutes a habitable environment, opening up thrilling possibilities for the existence of life beyond our planet.
Category | Example Organism | Habitat | Temperature Range (°C) | Notable Feature |
Type of Extremophile | Thermophiles | Hot Springs | 50-80 | Survive at high temperatures |
Type of Extremophile | Psychrophiles | Deep Oceans | -20-10 | Thrive in cold environments |
Type of Extremophile | Halophiles | Salt Flats | 20-50 | Adapted to high salinity |
Type of Extremophile | Acidophiles | Acidic Hot Springs | 25-80 | Flourish in acidic conditions |
Type of Extremophile | Radiophiles | Nuclear Reactors | 20-60 | Resistant to high radiation levels |
Type of Extremophile | Xerophiles | Deserts | 10-80 | Survive in arid conditions |
Type of Extremophile | Endoliths | Rock Structures | 5-70 | Live within rock or in mineral structures |
Extremophiles Overview
A. What Are Extremophiles and Why Are They Unique?
Extremophiles are truly remarkable microorganisms that are remarkably well-adapted to thrive in environments that are often deemed inhospitable for most other life forms, including those characterized by extreme temperatures, salinity, acidity, and pressure. They can be found in a surprising range of locations, such as scalding hot springs, the depths of deep-sea hydrothermal vents, and even in the frigid, icy expanses of polar regions, illustrating their astounding versatility. These extraordinary organisms possess unique biochemical adaptations, such as specialized proteins and cellular structures, that enable them to survive and function effectively where standard biological processes would typically fail. Notably, some extremophiles can withstand temperatures exceeding 100 degrees Celsius or salinities that would profoundly dehydrate ordinary living cells, showcasing an impressive and diverse array of metabolic pathways that remain largely unexplored by scientists. The diversity of ecological niches that extremophiles occupy is a vivid testament to their resilience and adaptability, as highlighted in various studies, and it underscores their crucial role in numerous biogeochemical cycles. Furthermore, the potential applications of extremophiles in burgeoning fields like biotechnology and astrobiology are immense and hold promise for advances in areas such as medicine and environmental sustainability. Their extraordinary ability to thrive in extreme conditions also raises compelling questions about the potential for extraterrestrial life, sparking scientific curiosity and research into environments beyond Earth. This exploration reveals why these organisms are not only unique in their characteristics but also critical to our understanding of the resilience and adaptability of life itself, both on our planet and potentially on others in the universe.
Type | Temperature Range (°C) | Habitat | Examples |
Thermophiles | 45 – 122 | Hot springs, hydrothermal vents | Thermus aquaticus |
Halophiles | 0 – 100 | Salt flats, salt mines | Halobacterium salinarum |
Acidophiles | 15 – 80 | Sulfuric hot springs, acidic mines | Ferroplasma acidarmanus |
Psychrophiles | -20 – 10 | Polar ice, deep-sea environments | Psychrobacter cryohalolentis |
Radiophiles | 20 – 60 | Radioactive waste sites | Deinococcus radiodurans |
Extremophiles Characteristics and Environments
B. The Importance of Studying Life in Extreme Environments
Studying life in extreme environments is crucial not only for understanding the resilience and adaptability of organisms but also for expanding our knowledge of life’s potential on other planets. Extremophiles, such as certain bacteria and archaea, thrive under conditions that would be lethal to most forms of life—such as high temperatures in geothermal hot springs, immense pressure in the deep oceans, and intense radiation in outer space. These remarkable organisms provide valuable insights into the biochemical processes that allow life to endure in such harsh conditions, firmly demonstrating the incredible versatility of life itself. Furthermore, understanding extremophiles can have practical applications in biotechnology, where their unique enzymes and metabolic pathways are harnessed for various industrial purposes, ranging from environmental cleanup to the development of novel pharmaceuticals. Their capacity to survive extreme temperatures, salinity, and pH levels makes them invaluable for enhancing industrial efficiency and developing innovative technologies. Research on these remarkable organisms also significantly informs astrobiology, as scientists investigate the possibility of finding life beyond Earth in similar extreme environments. By examining the ways extremophiles adapt to severe conditions, researchers can formulate hypotheses about how life might exist on planets with harsh climates or in extraterrestrial environments such as icy moons or other rocky bodies. Thus, studying extremophiles not only enriches our understanding of biology but also opens exciting avenues for future exploration and innovation, both on our planet and beyond, reinforcing the interconnectedness of life in the universe.
The Importance of Studying Life in Extreme Environments
- Expands Our Understanding of Life – Helps us learn how organisms survive in harsh conditions.
- Provides Insights into Evolution – Reveals adaptations that allow life to thrive in extreme habitats.
- Aids in the Search for Extraterrestrial Life – Offers clues about possible life on Mars and other planets.
- Advances Biotechnology and Medicine – Leads to discoveries of new enzymes, antibiotics, and biomaterials.
- Enhances Climate Change Research – Helps scientists understand how life adapts to extreme temperature shifts.
- Supports Astrobiology Studies – Shows how microbes can survive in space-like conditions.
- Improves Industrial Applications – Extremophiles produce heat- and pressure-resistant enzymes useful in industry.
- Aids Environmental Cleanup – Some extremophiles break down pollutants in extreme environments.
- Expands Our Knowledge of Microbial Diversity – Introduces new species with unique biological properties.
- Helps in Developing Sustainable Energy – Some extremophiles are used in biofuel production.
- Improves Food Processing Techniques – Thermophilic bacteria are used in high-temperature food fermentation.
- Supports Human Space Exploration – Research on extremophiles helps develop life-support systems for space travel.
- Contributes to Agriculture – Some extremophiles enhance soil fertility in arid or salty conditions.
- Reveals Earth’s Biological Limits – Helps define the boundaries of where life can exist.
- Protects Ecosystems – Understanding extremophiles aids in conserving fragile environments.
- Advances Synthetic Biology – Genetic engineering of extremophiles leads to innovative solutions.
- Provides Clues to Early Life on Earth – Studying extremophiles helps reconstruct ancient evolutionary pathways.
- Helps Discover Novel Bioactive Compounds – Leads to new antibiotics, antifungals, and anti-cancer drugs.
- Enhances Water Purification Technologies – Some extremophiles assist in treating contaminated water.
- Strengthens Interdisciplinary Scientific Research – Combines microbiology, chemistry, physics, and planetary science for groundbreaking discoveries.
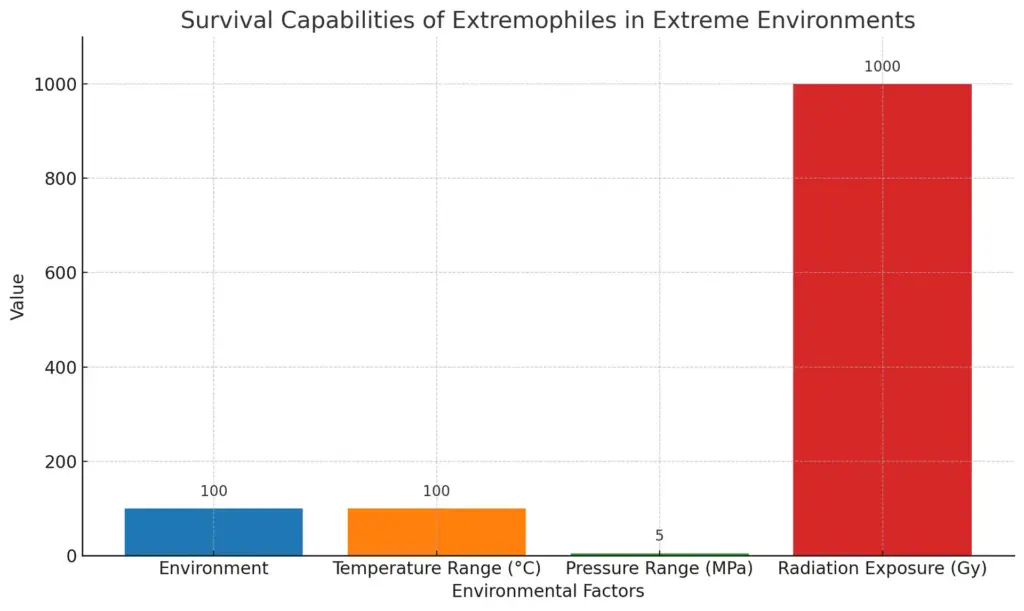
This bar chart illustrates the survival capabilities of extremophiles in various extreme environments, detailing their endurance in terms of temperature, pressure, and radiation exposure. The chart highlights that extremophiles can withstand high temperatures and pressures, with exceptional resilience to intense radiation levels, emphasizing their significance in the study of life in extreme conditions.
II. Types of Extremophilic Bacteria
Extremophilic bacteria showcase an astonishing array of adaptations that enable them to thrive in some of the most inhospitable environments on Earth. These remarkable organisms have evolved to survive conditions that would be lethal for most life forms, and among the diverse types are thermophiles, which flourish in extreme heat. These bacteria are often discovered in natural hot springs and geothermal areas, where temperatures can exceed 70 degrees Celsius, demonstrating their unparalleled ability to withstand high thermal stress. Acidophiles, on the other hand, prefer environments with low pH levels, such as acid mine drainage and acidic hot springs, where they can efficiently metabolize and thrive despite the harsh conditions. Additionally, halophiles are adapted to hypersaline environments, including salt flats and salt ponds, where they utilize specialized mechanisms to maintain osmotic balance. This adaptation is crucial for their survival, allowing them to extract water from highly saline surroundings. Each of these bacteria contributes significantly to their respective ecosystems by mediating essential biogeochemical cycles, which in turn supports diverse life forms. Furthermore, the study of extremophilic bacteria is not just limited to Earth; these organisms offer valuable insights into the potential for life on other celestial bodies, such as Mars, where radiation-contaminated sites may harbor organisms exhibiting similar resilience. The complexity and variety of these extremophiles are visually summarized in the diagram depicting their habitats, which enhances understanding of their ecological significance and intricate interactions within their environments. By studying these unique life forms with such hardy characteristics, researchers can uncover the fundamental principles of biological resilience and adaptability, yielding insights that could revolutionize our understanding of life beyond our planet.
Type | Ecosystem | Example Species | Optimal Temperature (°C) | Application |
Thermophiles | Hot Springs | Thermus aquaticus | 75 | PCR Enzyme (Taq polymerase) |
Psychrophiles | Polar Regions and Deep Oceans | Psychrobacter spp. | -12 | Cold-stable enzymes for food preservation |
Halophiles | Salt Lakes | Halobacterium salinarum | 20 | Biotechnology in saline conditions |
Acidophiles | Acidic Hot Springs | Ferroplasma acidarmanus | 1 | Bioremediation of metal-contaminated environments |
Alkaliphiles | Soda Lakes | Natronobacterium gregoryi | 10.5 | Biotechnology in alkaline conditions |
Radiophiles | Radiation-rich environments | Deinococcus radiodurans | 5000 | DNA repair in extreme radiation |
Xerophiles | Deserts | Bacillus spp. | 0.2 | Survival in arid conditions |
Types of Extremophilic Bacteria
A. Thermophiles: Bacteria That Thrive in Extreme Heat
Thermophiles are a remarkable group of microorganisms that have adapted to thrive in environments characterized by elevated temperatures, such as hot springs and hydrothermal vents. These fascinating organisms have evolved specialized cellular structures and metabolic pathways that enable them to withstand extreme heat, allowing them to perform biochemical processes that would be impossible for most organisms, which typically cannot survive beyond a certain temperature threshold. These bacteria not only contribute to the ecological balance in their native habitats by playing crucial roles in nutrient cycling and energy flow but also offer significant insights into biotechnology and industrial applications. For instance, enzymes derived from thermophiles are increasingly being utilized in industrial applications, including biofuels and pharmaceuticals, due to their remarkable stability and efficiency at high temperatures; this makes them invaluable in processes that require durable catalysts. The diversity of thermophilic species showcases their ecological niches and the unique conditions they endure, demonstrating various adaptations such as heat-stable proteins and robust cellular membranes. Understanding thermophiles thus provides critical knowledge not only about life in extreme environments on Earth but also hints at the potential for life in similar conditions elsewhere in the universe, such as the subsurface of other planets and moons where similar heat and chemical gradients may exist. Research into these hardy microbes continues to shed light on their evolutionary strategies, revealing secrets that could improve our industrial practices and deepen our understanding of the limits of life.
Species | Optimal Temperature (°C) | Habitat | Notable Feature |
Thermus aquaticus | 75 | Hot springs | Source of Taq polymerase used in PCR |
Thermococcus gammatolerans | 80 | Deep-sea hydrothermal vents | Highly resistant to gamma radiation |
Pyrococcus furiosus | 100 | Hydrothermal vents | Ferments carbohydrates at high temperatures |
Geobacillus stearothermophilus | 60 | Soil and compost heaps | Used as a biological indicator for sterilization processes |
Methanocaldococcus jannaschii | 85 | Deep-sea vents | Produces methane at high temperatures |
Thermophiles Data Overview
B. Halophiles: Salt-Loving Microbes in High-Salinity Environments
In high-salinity environments, such as salt flats and hypersaline lakes, halophiles demonstrate remarkable adaptations that allow them to thrive in ecological niches where most life forms cannot survive. These salt-loving microbes have developed an array of unique cellular mechanisms, including notable modifications to their proteins and enzymes, which enable their metabolic processes to function optimally despite the challenges posed by extreme salinity levels. For instance, halophiles often accumulate compatible solutes, such as potassium ions, within their cells to maintain osmotic balance, thereby preventing dehydration and ensuring cellular health. This process is essential for sustaining their life functions in environments that would be detrimental to most organisms. Additionally, their membrane structures are specifically adapted to withstand the high salt concentrations found in these habitats, thus ensuring cellular integrity and stability. Furthermore, the incredible diversity of habitats occupied by halophiles, exemplified in environments ranging from solar salt pans to sub-surface brines, highlights their ecological significance and remarkable evolutionary resilience. This adaptability not only illustrates the potential for life in extreme conditions but also prompts further investigation into their biochemical pathways that could inspire novel biotechnological applications. A diagram depicting various extremophilic environments, including salt flats, further enhances this understanding by visually connecting halophiles’ unique adaptations to their respective habitats. This visual representation allows researchers and enthusiasts alike to appreciate the intricate relationship between these microbes and their sometimes-harsh surroundings, deepening our understanding of biodiversity in extreme conditions.
Category | Details |
---|---|
Definition | Halophiles are microorganisms that thrive in environments with high salt concentrations (above 3% NaCl). They are found in salt flats, hypersaline lakes, salt mines, and marine environments. |
Types of Halophiles | – Slight Halophiles (3–5% salt) – Found in seawater. – Moderate Halophiles (5–20% salt) – Found in saline lakes. – Extreme Halophiles (20–30% salt) – Found in salt flats and brine pools. |
Examples of Halophiles | – Halobacterium salinarum – Archaea found in salt ponds. – Dunaliella salina – Algae that produce carotenoids. – Salinibacter ruber – Bacteria found in salt flats. |
Cellular Adaptations | – Produce osmoprotectants (e.g., glycine betaine, ectoine) to balance salt. – Modify cell membrane lipids to remain stable in high salinity. – Use specialized proteins and enzymes resistant to denaturation by salt. |
Metabolism | – Some halophiles are photoheterotrophs, using light and organic molecules. – Others are chemoheterotrophs, obtaining energy from organic matter. – Some halophilic archaea use bacteriorhodopsin for light-driven ATP production. |
Habitats | – Great Salt Lake, USA – Dead Sea, Middle East – Solar evaporation ponds – Hypersaline soils and deep-sea brines |
Ecological Role | – Contribute to nutrient cycling in saline ecosystems. – Produce pigments that help regulate light penetration in salt lakes. – Form microbial mats that support diverse microbial communities. |
Industrial & Biotechnological Uses | – Enzyme production – Salt-tolerant enzymes for detergents and biotechnology. – Bioplastics – Halophilic bacteria produce biodegradable plastics (PHA). – Bioremediation – Used for cleaning up saline industrial waste. – Food industry – Dunaliella salina produces β-carotene, used as a supplement. |
Medical & Pharmaceutical Applications | – Produce antimicrobial compounds effective against pathogens. – Source of extremozymes (enzymes stable in extreme conditions). – Potential use in cancer research for unique bioactive compounds. |
Importance in Astrobiology | – Halophiles can survive in Martian-like conditions, offering clues about extraterrestrial life. – Studying halophiles helps understand how life could exist on planets with saline environments. |
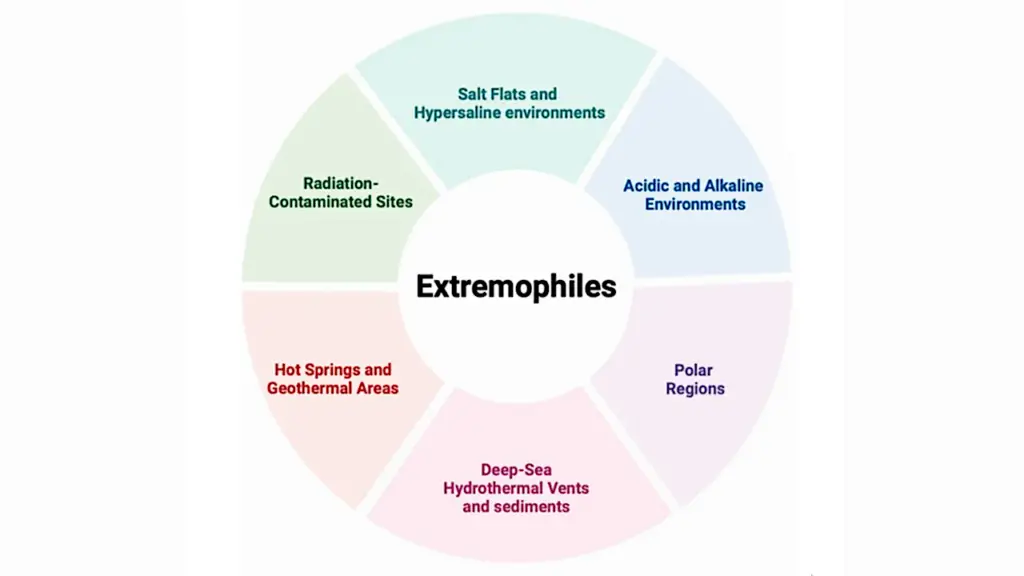
IMAGE – Categorization of Extremophiles and Their Habitats (The image is a diagram illustrating the various environments where extremophiles can be found. At the center is the term ‘Extremophiles,’ surrounded by six categories: ‘Radiation-Contaminated Sites,’ ‘Hot Springs and Geothermal Areas,’ ‘Deep-Sea Hydrothermal Vents and Sediments,’ ‘Polar Regions,’ ‘Acidic and Alkaline Environments,’ and ‘Salt Flats and Hypersaline Environments.’ Each category highlights the unique ecological niches that extremophiles inhabit, underscoring their adaptability and resilience to extreme conditions, which could be relevant for studies in microbiology, environmental science, and astrobiology.)
Microbe Name | Habitat | Optimal Salinity (%) | Temperature Range (°C) | Significance |
Halobacterium salinarum | Salt ponds, salt flats | 25 | 30-50 | Used in biotechnology, essential for certain biogeochemical processes |
Salinibacter ruber | Salt evaporation ponds | 30 | 20-40 | Provides insights into evolutionary processes of extremophiles |
Natronomonas pharaonis | Alkaline soda lakes | 16 | 30-45 | Explored for potential applications in astrobiology |
Halophiles Characteristics and Locations
C. Acidophiles and Alkaliphiles: Bacteria That Live in Extreme pH Levels
Acidophiles and alkaliphiles represent a fascinating subset of extremophiles, thriving in environments characterized by extreme pH levels, either acidic or alkaline. Acidophiles, such as those found in acid mine drainage and highly acidic hot springs, have adapted their cellular machinery and metabolic processes to function optimally in pH levels often below 3. This impressive resilience not only sheds light on the biochemical pathways that enable them to maintain cellular integrity and energy production in such hostile conditions but also underscores the incredible evolutionary adaptations that have taken place over time. These microorganisms have developed intricate mechanisms to combat the challenges posed by high concentrations of hydrogen ions, such as incorporating stable structural components in their cell membranes and utilizing specialized proteins that operate effectively under acidic conditions. Conversely, alkaliphiles inhabit environments with pH levels soaring above 9, such as soda lakes, where they employ unique adaptations, like specialized ion transport systems and unique enzyme functions, to survive extreme alkalinity. These adaptations include the production of enzymes that retain functionality in high pH, thus facilitating essential biochemical reactions that would otherwise be hindered in such conditions. The diversity of habitats occupied by these organisms not only highlights their extraordinary evolutionary strategies but also emphasizes the crucial ecological roles they play in nutrient cycling within various ecosystems. The interaction between acidophiles and alkaliphiles with their environments can drive significant biogeochemical processes, making their study increasingly important. Illustrating these adaptations and their habitats is essential for understanding their significance; thus, the diagram showing the sources of extremophiles is particularly relevant and acts as a valuable resource in this exploration of life at the extremes.
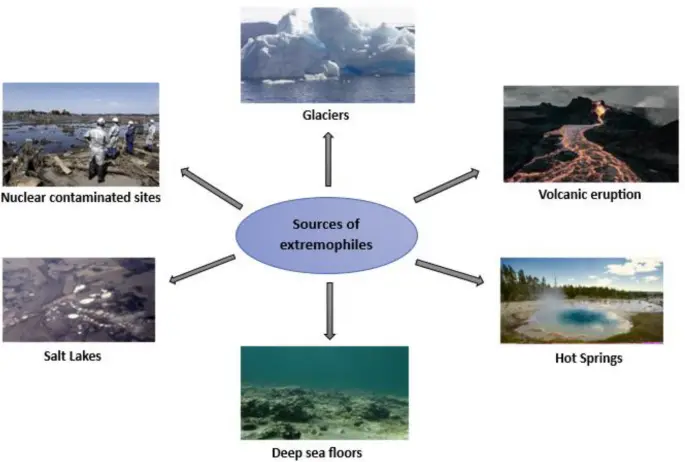
Image : Diagram of extremophile habitats and sources (The image depicts a diagram illustrating various sources of extremophiles, which are organisms that thrive in extreme environmental conditions. Central to the diagram is the phrase ‘Sources of extremophiles’, with arrows pointing to various images representing specific locations: glaciers, volcanic eruptions, hot springs, deep sea floors, salt lakes, and nuclear contaminated sites. Each image provides visual examples of the diverse habitats where extremophiles can be found, emphasizing their adaptation to extreme conditions. This diagram is relevant for academic discussions in the fields of biology, environmental science, and extremophiles research.)
Type | Optimal pH | Example species | Habitat | Temperature range Celsius |
Acidophile | 1.0 – 5.0 | Ferroplasma acidarmanus | Acid mine drainage | 25 – 50 |
Alkaliphile | 9.0 – 11.5 | Natronomonas pharaonis | Soda lakes | 30 – 45 |
Acidophile | 3.0 | Acidithiobacillus ferrooxidans | Mine environments | 15 – 45 |
Alkaliphile | 10.5 | Bacillus alcalophilus | Alkaline soils | 25 – 40 |
Characteristics of Acidophiles and Alkaliphiles
III. How Extremophiles Survive Harsh Conditions
Extremophiles, organisms that thrive in environments deemed inhospitable to most life forms, have evolved an astonishing array of sophisticated mechanisms to endure extreme conditions that would be lethal to other organisms. These remarkable adaptations can be categorized primarily into biochemical and structural modifications, which enable them to withstand intense heat, extreme pressure, and high levels of radiation. For instance, many thermophiles possess heat-stable proteins that not only maintain their functionality but also promote effective biochemical reactions even at elevated temperatures, allowing these organisms to flourish in places like hydrothermal vents. Moreover, their cell membranes are often enriched with saturated lipids, which bolster structural integrity under thermal stress, preventing the membranes from becoming too fluid and losing their functionality. Similarly, halophiles utilize specialized proteins that function optimally in environments with high salt concentrations, enabling them to effectively retain cellular integrity and maintain metabolic activity despite the challenges posed by osmosis. Additionally, other extremophiles employ unique survival strategies, such as the synthesis of protective compounds, including the sugar polymer trehalose, which plays a vital role in safeguarding cellular structures against desiccation and oxidative damage. By adapting to these hostile environments, extremophiles not only manage to survive but also play crucial roles in their ecosystems, contributing significantly to biogeochemical cycles and providing valuable insights into potential extraterrestrial life forms. Furthermore, the image depicting the various adaptation strategies of extremophiles can vividly illustrate these mechanisms, enhancing comprehension and appreciation of their remarkable survival tactics and the resilience of life in extreme conditions. This understanding may also pave the way for innovative applications in biotechnology and environmental science.
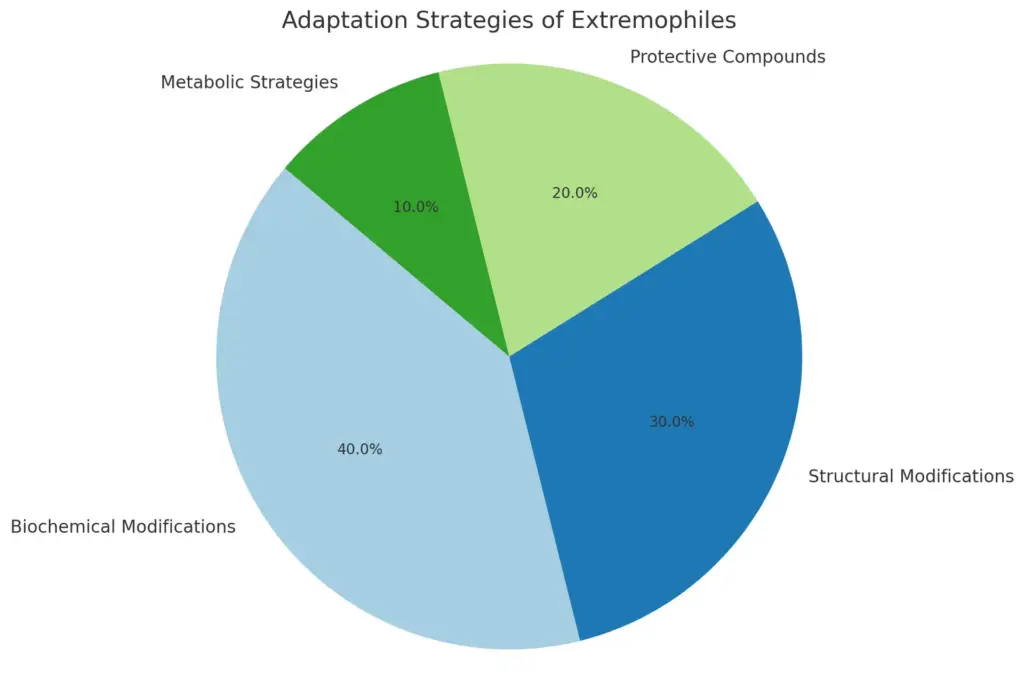
This pie chart illustrates the proportional distribution of various adaptation strategies used by extremophiles to survive harsh conditions. It highlights the significance of biochemical modifications, structural integrity, protective compounds, and metabolic strategies in their survival mechanisms.
Type | Survival Mechanism | Examples | Temperature Range (°C) | Habitat |
Thermophiles | Proteins and enzymes that remain stable at high temperatures | Thermus aquaticus, Pyrococcus furiosus | 60-121 | Hot springs and geothermal vents |
Halophiles | High concentrations of potassium ions to stabilize proteins | Halobacterium salinarum, Dunaliella salina | 0-70 | Salt flats and salt mines |
Acidophiles | Use of proton pumps to maintain internal pH | Ferrovum, Ferroplasma | 20-80 | Acidic hot springs and mining sites |
Psychrophiles | Enzymatic adaptations for cold activity | Psychrobacter, Colwellia | -20 to 10 | Antarctic ice and deep ocean |
Radiophiles | DNA repair mechanisms to withstand radiation | Deinococcus radiodurans | 0-50 | Nuclear waste sites and high radiation areas |
Xerophiles | Water-retaining mechanisms and biofilm formation | Noctiluca scintillans | 20-50 | Deserts and arid regions |
Extremophile Survival Mechanisms and Examples
A. Unique Adaptations in Their Cell Membranes
Extremophiles demonstrate remarkable adaptations in their cell membranes, enabling them to thrive in environments that would be inhospitable to most life forms. For instance, the phospholipid bilayers of bacteria found in hot springs are enriched with saturated fatty acids, which significantly enhance membrane stability against the high temperatures typically present in such extreme habitats. This adaptation is crucial for maintaining the functionality of cellular processes at elevated temperatures where most other organisms would simply cease to exist. In contrast, halophilic organisms that inhabit hypersaline conditions possess unique membrane structures that are packed with specific proteins designed to prevent dehydration, which is a constant risk in such desiccating environments. These specialized adaptations ensure that their cellular integrity is preserved despite the extreme osmotic pressures they face daily. Moreover, some extremophiles have not only developed additional protective layers or membranes but have also modified their lipid composition to resist the damaging effects of radiation or the corrosive influences of extreme pH levels. Such biochemical resilience is not merely a survival mechanism; it reflects a profound evolutionary response to the myriad of diverse ecological niches that these organisms occupy. The various environments where these extremophiles exist, underscore the extent of their adaptation and highlight the intricate complexity of life in extreme conditions. This reinforces the overarching theme of survival strategies that have evolved in response to the challenges posed by our planet’s most challenging habitats, showcasing nature’s incredible ingenuity in fostering life under seemingly impossible circumstances.
Extremophile Type | Adaptation | Temperature Stability (°C) | Source |
Thermophiles | High saturated fatty acid content in membranes | 70-121 | National Center for Biotechnology Information (NCBI) |
Halophiles | High levels of potassium ions to stabilize proteins | Up to 30 | Microbiology and Molecular Biology Reviews (MMBR) |
Psychrophiles | Unsaturated fatty acids in membranes to maintain fluidity | -12 to 10 | Frontiers in Microbiology |
Acidophiles | Highly acidic phospholipids that resist protonation | 0-5 | Journal of Bacteriology |
Radiophiles | Proteins and membranes resistant to radiation damage | Up to 15,000 | Environmental Microbiology |
Unique Adaptations in Cell Membranes of Extremophiles
B. DNA and Protein Stability Under Extreme Conditions
Extremophiles exhibit remarkable adaptations that enable their DNA and proteins to maintain stability under extreme conditions, such as high temperatures, intense radiation, and extreme salinity. In high-temperature environments like hot springs, these unique organisms possess specialized enzymes known as thermostable proteins, which maintain their structural integrity and function despite significant thermal stress that would typically denature proteins found in more temperate organisms. Additionally, some extremophiles employ unique DNA-binding proteins that help protect genomic material from denaturation, ensuring that their genetic code remains intact even under extreme environmental challenges. Interestingly, the evolutionary mechanisms driving these adaptations provide crucial insights into molecular stability; through studying these pathways, researchers can reveal potential applications in biotechnology and medicine, particularly in developing robust enzymes for industrial processes and therapies that exploit these stable biological systems. Understanding how these remarkable organisms withstand such harsh conditions not only advances our knowledge of microbiology but also informs fields like astrobiology, where the viability of life in extraterrestrial environments is a central question with profound implications for our understanding of life’s limits. This complexity is further illustrated by the diverse habitats occupied by extremophiles, as depicted in research studies, which effectively contextualizes their resilience and adaptability in extreme ecological niches. Their ability to thrive in conditions ranging from acidic hot springs to the salty depths of hypersaline lakes serves as an inspiration for innovative approaches to studying life, potentially leading to breakthroughs that could revolutionize various scientific disciplines.
Extremophile | Environment | DNA Stability | Protein Stability | Example species |
Thermophiles | Hot Springs | High | High | Thermus aquaticus |
Psychrophiles | Deep Oceans | Moderate | High | Psychrobacter cryohalolentis |
Halophiles | Salt Flats | Moderate | High | Halobacterium salinarum |
Acidophiles | Sulfuric Springs | Moderate | Moderate | Ferroplasma acidarmanus |
Radiophiles | High Radiation Areas | High | Low | Deinococcus radiodurans |
Xerophiles | Arid Regions | High | High | Mojavia monocytogenes |
Stability of DNA and Proteins in Extremophiles
IV. The Role of Extremophiles in Biotechnology
Extremophiles are increasingly pivotal in the field of biotechnology, primarily due to their unique cellular mechanisms and adaptations to extreme environments. These remarkable organisms have evolved to thrive under extreme conditions, such as high temperature, acidity, salinity, and even high pressure, where conventional organisms simply cannot survive. As a result of these extreme adaptations, they possess specialized enzymes known as extremozymes, which are highly stable and remarkably active even in harsh environments. This feature proves invaluable in a variety of industrial applications, including but not limited to biofuel production and bioremediation. For instance, enzymes extracted from thermophilic bacteria are widely utilized in laundry detergents and textile production because they can function effectively in hot water, leading to significant reductions in energy costs associated with heating water. Furthermore, the potential for extremophiles to produce bioactive compounds has spurred considerable interest, particularly in the fields of pharmaceuticals and agriculture, where their unique properties can lead to breakthroughs in drug development and crop resilience. The versatility of extremophiles not only highlights their essential contributions to the biotechnological sector, but also emphasizes their critical role in addressing pressing ecological and industrial challenges while fostering sustainable practices that benefit society as a whole. This relationship is beautifully illustrated in various studies, which showcase the diverse habitats of extremophiles and underscore their adaptability, a key advantage that underpins their many biotechnological applications. Whether as a source of novel enzymes or as a component of innovative processes, extremophiles are at the forefront of advancing biotechnology and enhancing our ability to meet future demands.
Extremophile Type | Environment | Biotechnological Application | Example Organism | Key Benefit |
Thermophiles | Hot Springs | Enzyme production for industrial processes | Thermus aquaticus | High heat tolerance |
Halophiles | Salt Lakes | Bioremediation | Halobacterium salinarum | Salt tolerance for bioremediation in saline environments |
Psychrophiles | Deep Oceans | Cold-active enzymes for food and textile industries | Psychrobacter cryohalolentis | Function at low temperatures, reducing energy costs |
Acidophiles | Acidic hot springs | Mining and bioleaching | Acidithiobacillus ferrooxidans | Efficiency in metal recovery from ores |
Radiophiles | Radioactive environments | Bioremediation of radioactive waste | Deinococcus radiodurans | High resistance to ionizing radiation |
Extremophiles in Biotechnology Applications
A. Enzymes from Extremophiles Used in Industry (PCR, Detergents)
Enzymes derived from extremophiles have truly revolutionized various industrial processes, particularly in the critical realms of biomedicine and detergent formulation, where traditional enzyme methods often fall short. These remarkable proteins exhibit exceptional stability and activity under conditions that would typically denature conventional enzymes, such as extremely high temperatures, extreme pH levels, and high salinity. For instance, DNA polymerases isolated from thermophilic bacteria are essential in polymerase chain reaction (PCR) methods, which facilitate the amplification of DNA, an important process for genetic studies, forensic investigations, and even medical diagnostics. Without these extremophilic enzymes, many modern molecular biology techniques would be significantly hampered. Additionally, proteases extracted from halophilic microorganisms have markedly enhanced detergent formulations, enabling efficient stain removal in laundry processes under varied water conditions, including those observed in hard or saline environments. The profound adaptability of these enzymes not only optimizes industrial applications but also highlights the vast biochemical potential of extremophiles residing in harsh environments, where survival often hinges on their unique enzymatic capabilities. Thus, the exploration and harnessing of extremophile enzymes signify not just a leap forward in biotechnology but also serve as a profound affirmation of nature’s ingenuity in developing innovative solutions for extreme conditions. This relevance extends to numerous scientific and commercial enterprises, shedding light on the ongoing need for industrial applications that can thrive in challenging environments while adopting sustainable practices. The ongoing research into extremophiles continues to uncover more about these extraordinary enzymes and their myriad potential applications across industries.
Application | Enzyme | Source Extremophile | Optimal Temperature (°C) | Market Demand (2022, Billion USD) | Key Industries |
Polymerase Chain Reaction (PCR) | Taq Polymerase | Thermus aquaticus | 75 | 1.2 | Biotechnology, Healthcare |
Detergents | Proteases | Bacillus licheniformis | 60 | 1.5 | Household Products, Industrial Cleaning |
Biosensors | Lipases | Thermococcus spp. | 85 | 0.8 | Food Industry, Environmental Monitoring |
Biotechnology | Amylases | Thermophilic bacteria | 70 | 1 | Food Processing, Brewing |
Industrial Applications of Extremophile Enzymes
B. How Extremophiles Are Used in Space Research
Extremophiles have become pivotal in advancing our understanding of potential life forms beyond Earth, making them indispensable in space research. By meticulously analyzing the resilience of these remarkable microorganisms, scientists can draw meaningful parallels between their extreme habitats—such as the astonishing ecosystems found in deep-sea hydrothermal vents and the incredibly hostile environments of volcanic hot springs—and the harsh conditions that can be found on other celestial bodies, including Mars or Europa. These comprehensive studies significantly aid in designing innovative experiments for various space missions that are fundamentally aimed at discovering life or bio-signatures on other planets. Furthermore, they provide invaluable insights into how life can originate and thrive in adverse or extreme environments that might initially seem uninhabitable. Moreover, extremophiles, such as the intriguing tardigrade, which is renowned for its exceptional ability to survive extreme radiation and severe desiccation, offer valuable models that can be utilized in the development of robust life-support systems intended for long-duration space travel. Such meticulous research not only enhances our comprehension of life’s remarkable adaptability but also profoundly informs the fields of astrobiology and planetary protection protocols, ensuring the safe exploration of other worlds. This ongoing inquiry into extremophiles is crucial for future explorations of extraterrestrial environments where life may exist or may have existed in the past, thereby broadening our understanding of life’s potential and the conditions required for its emergence beyond our planet. As we deepen our studies into these extraordinary organisms, we inch closer to answering the age-old question of whether we are alone in the universe.
Extremophile Type | Application | Source | Year |
D. radiodurans | Studying radiation resistance | NASA | 2022 |
Thermococcus gammatolerans | Heat resistance and biochemistry | JPL | 2023 |
Deinococcus spp. | Astrobiology research on Mars | Space.com | 2023 |
Halococcus hamelinensis | Studying potential for life in saline environments | Astrobiology Magazine | 2021 |
Desulforudis audaxviator | Microbial life in extreme conditions analogs | Frontiers in Microbiology | 2020 |
Applications of Extremophiles in Space Research
V. Conclusion – What Studying Extremophiles Can Teach Us About Life on Other Planets
The study of extremophiles provides critical insights into the possibilities of life beyond Earth, particularly in environments previously thought to be inhospitable. By understanding how these remarkable organisms thrive under extreme conditions—such as the high radiation levels experienced in outer space, or the extreme temperatures found at hydrothermal vents and in the frigid polar regions—scientists can formulate educated speculations on the potential existence of life on other planets like Mars or Europa. For instance, the extraordinary adaptability of extremophiles to harsh environments characterized by high salinity, intense acidity, or even complete lack of sunlight suggests that similar microorganisms could conceivably exist in extraterrestrial habitats where conditions mirror those they endure. The diagram illustrating various extremophilic environments reinforces the astounding diversity of niches occupied by these resilient organisms, highlighting their remarkable ability to survive despite severe challenges. Such knowledge not only expands our comprehension of life’s tenacity and versatility but also significantly informs astrobiological exploration strategies. It effectively guides missions to celestial bodies, directing the search towards those environments where conditions may harbor unknown life forms waiting to be discovered. Ultimately, studying extremophiles is a key step in unraveling the complex puzzle of life’s universality across the cosmos, offering valuable insights that broaden our understanding of biology as a phenomenon that might be prevalent not only on Earth but also throughout the universe. As we continue to explore the frontiers of space, the lessons learned from extremophiles will be indispensable in shaping our approach to the search for extraterrestrial life.
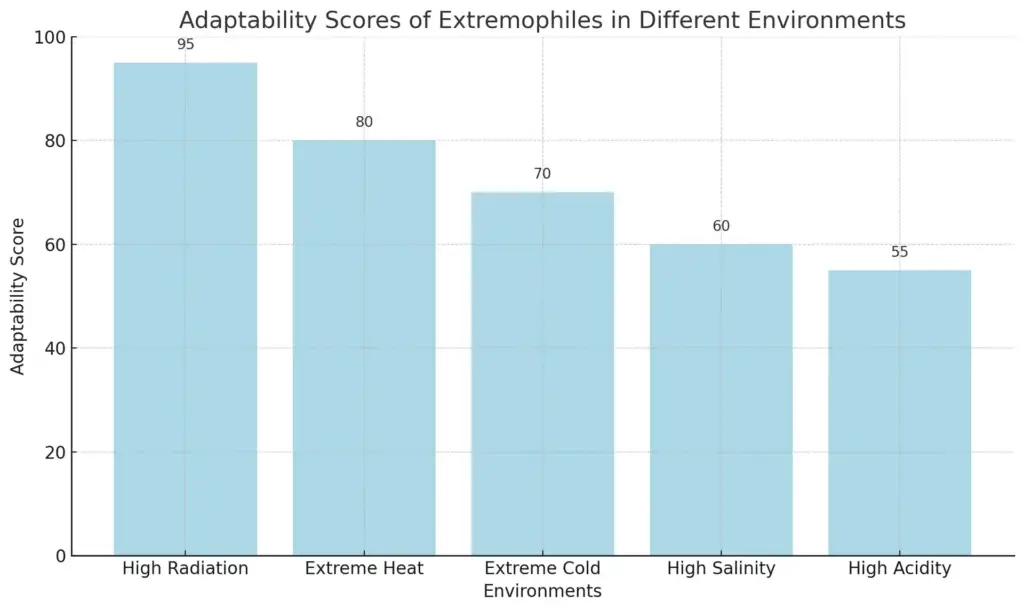
This bar chart illustrates the adaptability scores of extremophiles across various environments. It highlights their survival capabilities against extreme conditions such as high radiation, extreme temperatures, high salinity, and acidity. The scores suggest the potential for similar microorganisms to exist on other planets, aligning with research on extremophiles and extraterrestrial life.
What Studying Extremophiles Can Teach Us About Life on Other Planets
- Expands Our Definition of Habitability – Shows that life can exist in extreme conditions beyond Earth.
- Reveals Adaptations to Harsh Environments – Helps us understand how organisms survive extreme heat, cold, radiation, and pressure.
- Provides Clues About Potential Alien Life – Suggests that microbes could thrive on planets with extreme conditions like Mars or Europa.
- Helps Identify Biosignatures – Guides the search for chemical and molecular signs of life on other worlds.
- Aids in the Design of Life-Detection Missions – Informs space probe instrumentation for detecting microbial life.
- Demonstrates Survival in Space-Like Conditions – Some extremophiles can withstand vacuum, radiation, and extreme temperatures.
- Suggests Possibilities for Subsurface Life – Supports the idea that microbial life may exist underground on Mars or in icy moons.
- Guides Astrobiology Research – Provides real-world examples of how life may function in alien ecosystems.
- Improves Understanding of Early Earth Life – Helps reconstruct how life emerged and evolved under extreme conditions.
- Supports Terraforming and Colonization Efforts – Extremophiles may play a role in creating habitable environments on other planets.
- Explains How Life Can Use Alternative Energy Sources – Some extremophiles survive without sunlight, hinting at life in dark alien oceans.
- Prepares Us for Contamination Risks – Helps develop protocols to avoid spreading Earth microbes to other planets.
- Shows the Role of Extremophiles in Ecosystem Formation – Provides insights into how life could shape planetary environments.
- Aids in Understanding Chemical Evolution – Demonstrates how life may utilize unique biochemical pathways in different planetary conditions.
- Explores the Potential for Alien Microbial Symbiosis – Suggests that life forms on other planets might have complex interactions.
- Helps Predict Habitability Zones Beyond Our Solar System – Assists in determining which exoplanets could support life.
- Enhances Our Ability to Recognize Non-Carbon-Based Life – Encourages looking beyond Earth-like chemistry for extraterrestrial life.
- Develops New Technologies for Space Exploration – Research on extremophiles inspires innovations in robotics, materials, and life support.
- Strengthens Interdisciplinary Scientific Collaboration – Combines microbiology, planetary science, and chemistry to study astrobiology.
- Inspires the Search for Life Beyond Earth – Drives curiosity and exploration in the quest to answer whether we are alone in the universe.
References:
- Alistair McCleery. ‘An Introduction to Book History.’ David Finkelstein, Routledge, 3/13/2006
- Thombre, Rebecca. ‘Physiology, Genomics, and Biotechnological Applications of Extremophiles.’ Gunjal, Aparna B., IGI Global, 12/10/2021
- Constance M. Bertka. ‘Exploring the Origin, Extent, and Future of Life.’ Philosophical, Ethical and Theological Perspectives, Cambridge University Press, 9/3/2009
- Daniel Deudney. ‘Dark Skies.’ Space Expansionism, Planetary Geopolitics, and the Ends of Humanity, Oxford University Press, 3/2/2020
- Helga Stan-Lotter. ‘Extremophiles as Astrobiological Models.’ Joseph Seckbach, John Wiley & Sons, 1/13/2021
- D. V. Subba Rao. ‘Extremophiles.’ From Biology to Biotechnology, Ravi V. Durvasula, CRC Press, 1/9/2018
- Ralph Williams. ‘Thermus Species.’ Richard Sharp, Springer Science & Business Media, 12/6/2012
- K. Tsujii. ‘Extremophiles in Deep-Sea Environments.’ K. Horikoshi, Springer Science & Business Media, 11/1/1999
- K. Tsujii. ‘Extremophiles in Deep-Sea Environments.’ K. Horikoshi, Springer Science & Business Media, 12/6/2012
- Mohammed Kuddus. ‘Microbial Extremozymes.’ Novel Sources and Industrial Applications, Academic Press, 8/20/2021
- Pierre Genevaux. ‘Functions and Mechanisms of Bacterial Protein Homeostasis and Stress Responses.’ Axel Mogk, Frontiers Media SA, 2/1/2022
- RW Fitzpatrick. ‘Clays: Controlling the Environment.’ GJ Churchman, Csiro Publishing, 1/1/1995
- T.D. Brock. ‘Thermophilic Microorganisms and Life at High Temperatures.’ Springer Science & Business Media, 12/6/2012
- Ali Asghar Rastegari. ‘Microbiomes of Extreme Environments.’ Biodiversity and Biotechnological Applications, Ajar Nath Yadav, CRC Press, 3/21/2021
- Pabulo H. Rampelotto. ‘Extremophiles and Extreme Environments.’ Mdpi AG, 10/1/2018