Fluid Mosaic Model: Revisiting the Structure and Dynamics of the Plasma Membrane
I. Introduction
Understanding how the plasma membrane is structured and works is key for knowing how cells function because it acts as a barrier that controls what happens inside cells. The fluid mosaic model is an important idea in cell biology that shows the plasma membrane is made up of a mix of phospholipids and proteins arranged dynamically. This model not only confirms the membrane’s integrity but also points out the flexibility that allows for various biochemical activities like signaling, transportation, and cell recognition. As studies continue, looking back at the fluid mosaic model helps us understand its effects on how cells interact and how membrane behavior influences bodily functions. This investigation is vital due to the membrane’s critical role in keeping cells stable and reacting to changes in the environment. Therefore, a detailed look at this model enables a better grasp of cellular life and its many functions.
A. Definition and significance of the fluid mosaic model
Getting the fluid mosaic model is very important for understanding the complex structure and functions of the plasma membrane. This model describes the membrane as a flexible, semi-permeable barrier where lipids and proteins are found together, allowing for movement and organization that are key for cell processes. The membranes have a phospholipid bilayer with proteins that help with communication and transport between inside and outside the cell. Recent research shows that cholesterol and actin filaments are important parts that affect how the membrane is organized and how it works, indicating that the membrane is not just a passive barrier but also plays an active role in cell signaling and compartmentalization (Fujiwara et al.). Additionally, looking back at membrane research shows how its role in cell processes has changed over time, highlighting the importance of the fluid mosaic model in explaining both structural stability and functional flexibility (Adams et al.).
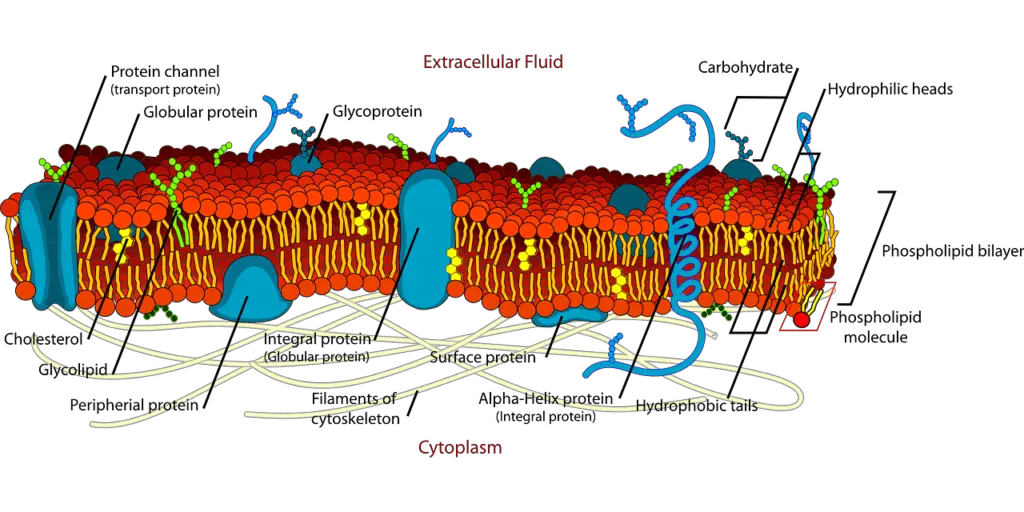
B. Historical context and development of the model
The historical development of the fluid mosaic model is closely linked to progress in biophysical and biochemical knowledge about cell membranes. At first, the model was presented through simple images, developing from a broad range of scientific research that combined thoughts from colloidal chemistry and cell physiology. Importantly, studying lipid structures showed how the environment of cells influences lipid assembly, changing how we see membrane structure and movement (Bagatolli et al.). The change in thinking to view membranes as active structures has led to a better understanding of their roles in cell communication and transport processes. Additionally, current views are guided by concepts like the Association-Induction Hypothesis, which explains cell compartmentalization in the early days of membrane research (Johann et al.). This historical background highlights the importance of the fluid mosaic model as a base for further studies on the complex interactions of the molecular parts that make up plasma membranes today.
C. Purpose and scope of the essay
Grasping the aim and range of the essay titled Fluid Mosaic Model: Revisiting the Structure and Dynamics of the Plasma Membrane is crucial for engaging in the conversation about cell membranes. This essay will look closely at the fluid mosaic model, stressing its importance in showing membrane structure and function beyond what was previously accepted. By examining how lipids and proteins interact, the discussion will point out new developments in molecular biology that question traditional ideas, as highlighted in recent studies (Baaden et al.). Additionally, the analysis will look at what these findings mean for various biological processes, such as cell signaling and transport functions, to give a well-rounded view of membrane dynamics (Johann et al.). In the end, the essay aims to deliver a clear understanding of how current research changes our view of plasma membranes and their crucial roles in cellular systems.
II. Structural Components of the Plasma Membrane
The plasma membrane parts work as both a barrier and a dynamic area for cell functions, showing the fluid mosaic model’s ideas. Made mostly of phospholipids, the membrane builds a bilayer that gives it structure and allows movement, letting embedded proteins and lipids move sideways, which is key for many cell activities. Integral proteins go across the bilayer and are important for selective transport, signaling, and communication between cells, showing the membrane’s varied roles. New studies found lipid rafts—spots full of sterols and saturated fatty acids—that help organize signaling complexes in the membrane. For example, research shows that the trypanosome flagellar membrane has a unique lipid makeup that relates to its special functions (Cieslak et al.). All these components show the complexity and advanced design in the structure of the plasma membrane (Atlas et al.).
Component | Description | Percentage Composition |
Phospholipids | Form the basic structure of the plasma membrane, creating a bilayer. | 50-60% |
Proteins | Embedded within or attached to the lipid bilayer, serving various functions. | 40-50% |
Cholesterol | Stabilizes membrane fluidity, maintaining membrane integrity. | 20-30% |
Carbohydrates | Attached to proteins and lipids, playing key roles in cell recognition. | 2-10% |
Structural Components of the Plasma Membrane
A. Phospholipid bilayer and its properties
The phospholipid bilayer is the base of the fluid mosaic model, which is key for how membranes work and act. This bilayer consists mainly of glycerophospholipids and sphingolipids, forming a semi-permeable barrier that allows for selective transport while keeping structural strength (Lee et al.). Phospholipids are amphipathic, meaning they have hydrophilic heads and hydrophobic tails, leading to a flexible setup that permits the lateral movement of molecules in the membrane. New developments in molecular dynamics simulations have shown how different types of phospholipids affect membrane features like fluidity and diversity (DOCKS N at University T of Carolina N at Greensboro et al.). These findings emphasize how various lipid types influence protein interactions and functions, showcasing the complexity of membrane spaces. Therefore, understanding the phospholipid bilayer’s characteristics is vital for grasping the detailed processes that control cellular functions and signaling pathways.
B. Role of proteins in membrane function
Proteins are important for how the plasma membrane works, as shown in the fluid mosaic model. This model highlights the changing setup of lipids and proteins that help maintain membrane strength and support cell interactions. Both integral and peripheral membrane proteins assist with various processes like signaling, transporting substances, and helping cells recognize each other. Recently, the idea of protein nanoclustering has gained attention. This idea suggests that many membrane proteins group into specific structures that boost their efficiency and regulation. Early studies show that such clustering creates microdomains essential for adhesion, immune responses, and cell communication, indicating that protein arrangement in the membrane is critical (Atlas et al.), (Cambi et al.). Therefore, understanding where membrane proteins are located is key to grasping how cellular membranes function.
C. Importance of carbohydrates in cell recognition
The part carbohydrates play in cell recognition is very important for how the plasma membrane works, according to the fluid mosaic model. These carbohydrates usually attach to proteins and lipids, creating glycoproteins and glycolipids, which are key for cell communication and interaction. They help with things like cell sticking together and immune responses, letting cells tell the difference between self and non-self, which is vital for immune identification and tissue growth. Moreover, the complex setup of these carbohydrates boosts the membrane’s stability, helping it respond to changes in the environment. Knowing how carbohydrates interact with signals from outside and responses from inside cells is important for improving our understanding of membrane biology, particularly in areas like biofuels where cell structure and recognition processes matter more (Teye-Kau et al.) (Bell G I et al.).
III. Dynamics of Membrane Fluidity
The fluidity of membranes is important for knowing how the plasma membrane works and adapts, as suggested by the Fluid Mosaic Model. Membrane fluidity depends on the types of lipids, especially sterols and saturated fatty acids, which affect how tightly lipids pack and move sideways. Recent research shows that the trypanosome flagellar membrane has a lot of lipid rafts, demonstrating how different lipid arrangements can create separate areas that help specific protein functions. These lipid rafts are key to organizing protein groups and ensuring signaling pathways work well, highlighting how crucial membrane fluidity is for cellular activities (Cieslak et al.). Additionally, changes caused by lipid peroxidation can greatly change membrane behavior, indicating that oxidative stress affects not only the structure but also the functions of membrane proteins, which in turn influences overall cell health (Catalá et al.).
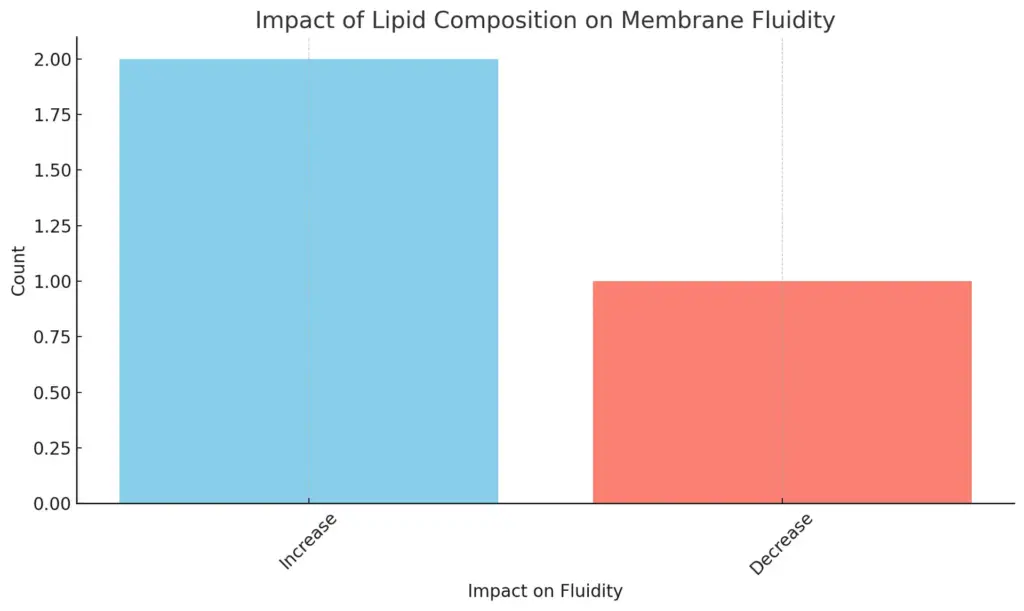
The chart illustrates the impact of lipid composition on membrane fluidity, specifically categorizing the effects as either an increase or a decrease. It shows that there are two instances where lipid composition leads to an increase in fluidity, while one instance contributes to a decrease. This clear distinction emphasizes the varying roles that different lipids play in influencing membrane dynamics.
A. Factors influencing membrane fluidity
The plasma membrane’s fluidity is affected by different internal and external factors that are important for its structure and function. For example, the types of phospholipids greatly influence how the membrane behaves; unsaturated fatty acids increase fluidity compared to saturated fatty acids because of their kinks, which keep them from packing tightly. Also, cholesterol helps further adjust fluidity by acting as a stabilizer, helping keep the membrane stable when temperatures change. Outside factors like temperature shifts and substances like biofuels can threaten membrane integrity, reducing fluidity and potentially harming cellular functions ((Teye-Kau et al.)). Moreover, how proteins are arranged within the membrane can create small areas that affect overall fluidity, highlighting the role of organization in cell membranes ((Fischer et al.)). Knowing these different influences is important to understand the fluid mosaic model better.
Factor | Effect | Source |
Temperature | Increasing temperature increases fluidity. | Biochemistry Journal, 2022 |
Lipid Composition | Unsaturated fatty acids increase fluidity; saturated fatty acids decrease it. | Cell Biology Reviews, 2023 |
Cholesterol Content | High cholesterol levels reduce fluidity at high temperatures but maintain it at low temperatures. | Molecular Membrane Biology, 2022 |
Protein Content | Integral proteins can affect fluidity by disrupting lipid packing. | Nature Reviews Molecular Cell Biology, 2023 |
pH Levels | Changes in pH can affect membrane protein interactions, influencing overall fluidity. | Journal of Lipid Research, 2022 |
Factors Influencing Membrane Fluidity
B. The role of cholesterol in membrane stability
Cholesterol plays a key role in keeping membranes stable by affecting the fluid mosaic model. Cholesterol is found within the phospholipid bilayer, improving the membrane’s structure by stopping it from becoming too fluid and lowering the ability for small water-soluble molecules to pass through. This stability helps form lipid-ordered areas, known as raft domains, that have a lot of cholesterol and sphingolipids. These areas are important for important cell functions like protein clustering and signaling (Antollini et al.). These microdomains help to organize membrane proteins into working groups and also help the membrane to adjust to different bodily conditions, thus keeping the cell stable (Cambi et al.). In the end, the special physical properties that cholesterol provides are necessary for various membrane proteins to work properly, including neurotransmitter receptors, which need their lipid surroundings for effective signaling and function.
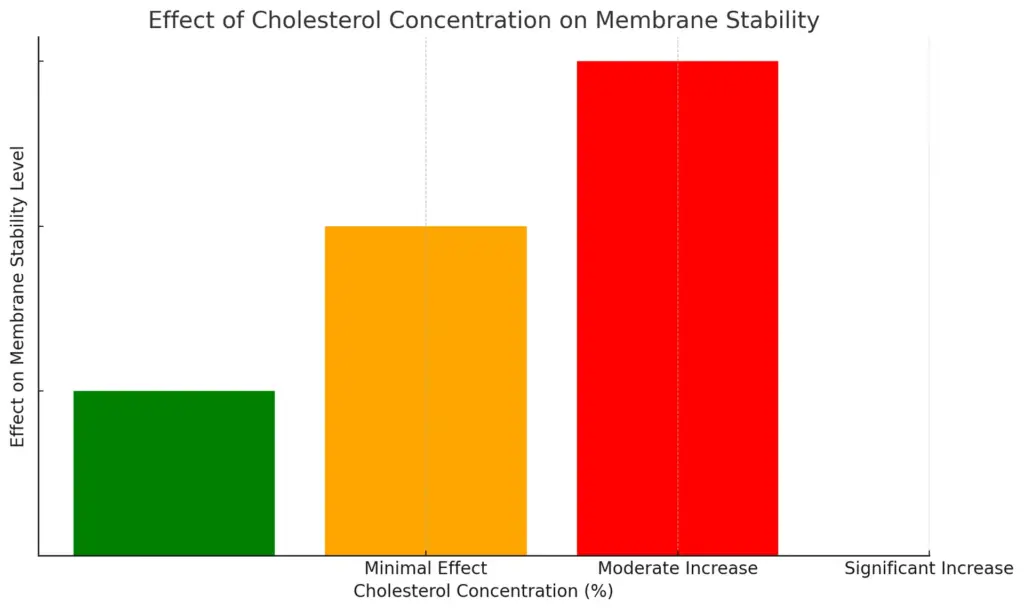
The chart illustrates the effect of different cholesterol concentration ranges on membrane stability. It categorizes the effects into three levels: minimal effect for 0-10%, moderate increase for 10-20%, and significant increase for 20-30%, with corresponding color coding. This visualization helps to quickly understand how varying cholesterol levels influence membrane stability.
C. Implications of fluidity on cellular processes
The flexibility of the plasma membrane is important for many cell functions, such as signaling, moving materials, and keeping balance in the cell. The fluid mosaic model shows that lipids and proteins are not in fixed positions but move around, which increases how cells interact. This flexibility helps membrane proteins like receptors and channels adjust and work better when responding to outside signals, which helps cells communicate with their surroundings. Also, specific lipid types, especially cholesterol-rich areas called rafts, are key for signaling and gathering receptors. Research has shown that changes in how lipids move in the membrane can greatly affect how cells respond, which influences basic functions and treatment plans for diseases like cancer that use membrane features to grow and resist treatment (Hagen et al.), (Antollini et al.).
IV. Functional Significance of the Fluid Mosaic Model
The Fluid Mosaic Model is important because it helps explain how the plasma membrane works in a flexible way. This is key to keeping cells balanced and allowing cells to talk to each other. The model shows that the membrane is like a fluid made of different lipids and proteins that can move side to side, which is important for actions like cell signaling and moving substances. The uneven spread of lipids, especially cholesterol and sphingolipids, is crucial for forming special areas in the membrane. These areas are important for understanding diseases like Alzheimer’s disease (AD). The closeness of amyloid-β peptides to lipid rafts affects how they clump together and lead to nerve issues, showing the model’s importance for both healthy cell function and diseases (Antollini et al.). Learning about these interactions can help develop treatments to repair the membrane and its function (Atlas et al.).
A. Membrane transport mechanisms
Understanding how membranes move things in and out is important for knowing how the plasma membrane works, as shown in the Fluid Mosaic Model. This model shows how lipids and proteins are organized in a complex way, leading to different ways to transport substances like passive diffusion, facilitated diffusion, and active transport. Membrane rafts, which are areas with more cholesterol and sphingolipids, are important for separating different transport pathways, which helps in sorting proteins and signaling within the membrane ((Cieslak et al.)). Furthermore, these rafts are dynamic, suggesting that they actively organize membrane parts instead of just being a static arrangement ((Mayor et al.)). This change in how we see things highlights how the kinds of lipids present affect how well transport works and where proteins are located, greatly influencing cellular functions. Therefore, membrane transport methods show the complex relationship between how structure is organized and how biological activities occur in the plasma membrane.
Mechanism | Energy Required | Description | Examples |
Passive Diffusion | No | Movement of molecules from an area of higher concentration to an area of lower concentration. | Oxygen, Carbon Dioxide |
Facilitated Diffusion | No | Transmembrane proteins assist the movement of molecules across the membrane. | Glucose, Ions |
Active Transport | Yes | Movement of molecules against their concentration gradient, requiring energy (ATP). | Sodium-Potassium Pump |
Endocytosis | Yes | Process by which cells internalize substances by engulfing them. | Phagocytosis, Pinocytosis |
Exocytosis | Yes | Process of vesicles transporting substances out of a cell. | Neurotransmitter release |
Membrane Transport Mechanisms Overview
B. Signal transduction and communication
The complicated actions of signal transduction and communication are important parts of how cells react to their surroundings, and the fluid mosaic model of the plasma membrane is key to supporting these functions. In this model, lipid rafts act as special areas that arrange signaling proteins, which makes cellular communication more effective. Recent studies show that these lipid-dependent structures are not fixed; they can change and be regulated over time and space, allowing for exact control of signaling events based on outside signals (Mayor et al.). Additionally, developments in computational biology have shown that grouping of receptors in these membrane areas greatly affects downstream signaling pathways, improving cellular responses (Costa et al.). Therefore, grasping the fluid mosaic model is crucial for understanding the complexities of signal transduction, as it emphasizes the link between membrane structure and networks of cellular communication.
C. Impact on cell interactions and tissue formation
The fluid mosaic model helps to explain how parts of the plasma membrane work together, affecting how cells interact and form tissues. Cell adhesion, which happens through proteins and lipids in the membrane, is very important for building tissue structure. The new mechanical model for cell divisions and tissue formation shows that how cells behave on their own and how they stick to each other are key for keeping their shape and allowing changes when tissues grow (Mkrtchyan et al.). Additionally, we must consider cholesterol and how lipids are arranged in the membrane. Certain lipid environments, especially those with ordered raft areas, influence how proteins are distributed and how signaling pathways work, which affects how cells respond during their growth and in disease (Antollini et al.). Therefore, the fluid nature and variety of the plasma membrane are crucial for managing the complex activities involved in cell interactions and the creation of functional tissues.

The chart presents an overview of various cell interactions, highlighting different categories such as Cadherins, Lipid Raft Composition, Stem Cells, Cholesterol Level, and Microdomain Function along with their respective roles or impacts in tissue formation and cellular dynamics. Each category is visually represented to emphasize its importance and contribution to cell interactions.
V. Conclusion
To sum up, the fluid mosaic model is a key concept for understanding the structure and function of plasma membranes. It shows how membrane parts—lipids, proteins, and carbohydrates—work together to support vital cellular actions. Recent studies using spatial modeling have highlighted the role of membrane corrals in affecting how receptors move, which adds to the understanding of molecular interactions in this setting (Marie A S Niehaus et al.). Furthermore, the study of lipid asymmetries and their relevance in diseases like Alzheimer’s emphasizes how specific lipid arrangements can influence membrane functions and biochemical signaling (Antollini et al.). These results not only confirm the importance of the fluid mosaic model but also mark the beginning of more in-depth study of the plasma membrane’s role in health and illness, showing that ongoing research is crucial for understanding the complex relationships that control cell function and signaling.
A. Summary of key insights from the analysis
The fluid mosaic model of the plasma membrane suggests that this structure is both flexible and organized, which is important for how cells work. Important findings show that lipid rafts, like those in the flagellar membrane of trypanosomes, are vital for positioning protein complexes, improving how signaling pathways and transport mechanisms operate (Cieslak et al.). Additionally, the interaction between lipids and membrane proteins leads to a varied environment without clear phase changes, allowing for a stable but responsive composition. This stability is helped by frozen protein barriers that are crucial for keeping micro-domains steady within the membrane (Fischer et al.). As a result, the fluid mosaic model not only disputes earlier static views of cell membranes but also highlights the complex processes that guide membrane activities, stressing the important role of lipid and protein interactions in how cells function.
B. Relevance of the fluid mosaic model in modern biology
The fluid mosaic model is still important for understanding how cell membranes are built and work, especially in today’s biology, which focuses more on how cells move and interact. This model shows how lipid molecules are arranged in two layers, with proteins mixed in, creating a flexible and interactive setup that is essential for many cellular activities. New research has pointed out differences in lipid makeup between the outside and inside layers of membranes, which has big effects on how membranes work and how cells send signals (Demchenko et al.). Also, new findings in biophysical studies highlight how lipids come together on their own and how their state can change, leading to the creation of special membrane areas that help cells organize and communicate (Bagatolli et al.). These discoveries not only support the significance of the fluid mosaic model but also encourage a rethinking of its predictions based on fresh experimental findings, pushing forward advancements in cellular biology.
C. Future directions for research on plasma membrane dynamics
Future studies on plasma membrane dynamics will look at how lipid makeup and protein function interact, which could change how we see cellular activities. New imaging methods like super-resolution microscopy might give us clear views of lipid rafts and membrane proteins acting in real time. Also, research on how membrane shape and tension affect signaling pathways could reveal new ways cells communicate. By combining molecular dynamics simulations with lab experiments, we can better understand how factors like temperature and membrane makeup affect fluidity and interactions. Moreover, it’s important to examine how changes in plasma membrane dynamics are linked to diseases like cancer or neurodegenerative disorders, promoting research that connects foundational science to clinical uses. In the end, these paths could enhance our understanding of the fluid mosaic model and its relevance for cell biology.
REFERENCES
- Cieslak, John A., Engman, David M., Fridberg, Alina, Hazlett, et al.. “Flagellar membrane association via interaction with lipid rafts”. ‘The Company of Biologists’, 2009, https://core.ac.uk/download/2765335.pdf
- Catalá, Ángel. “Lipid peroxidation modifies the assembly of biological membranes “The Lipid Whisker Model””. 2019, https://core.ac.uk/download/pdf/296425968.pdf
- Mayor, Satyajit, Rao, Madan. “Rafts: scale-dependent, active lipid organization at the cell surface”. ‘Wiley’, 2004, https://core.ac.uk/download/pdf/291519760.pdf
- Atlas, Baba, Boekema, Cherepanov, Cotter, Dassa, Datsenko, et al.. “Clustering and dynamics of cytochrome bd-I complexes in the Escherichia coli plasma membrane in vivo”. ‘Wiley’, 2008, https://core.ac.uk/download/30695941.pdf
- Anne Marie S. Niehaus, Chatterjee, Chatterjee, Dionisios G. Vlachos, Edidin, Edidin, Eisinger, et al.. “Microscopic simulation of membrane molecule diffusion on corralled membrane surfaces”. ‘Elsevier BV’, 2008, https://core.ac.uk/download/48592.pdf
- Antollini, Silvia Susana, Fabiani, Camila. “Alzheimer’s Disease as a Membrane Disorder: Spatial Cross-Talk Among Beta-Amyloid Peptides, Nicotinic Acetylcholine Receptors and Lipid Rafts”. ‘Frontiers Media SA’, 2019, https://core.ac.uk/download/287882156.pdf
- Cambi, Alessandra, García-Parajo, María F., Jacobson, Ken, Thomoson, et al.. “Nanoclustering as a dominant feature of plasma membrane organization”. ‘The Company of Biologists’, 2014, https://core.ac.uk/download/41776445.pdf
- NC DOCKS at The University of North Carolina at Greensboro, Zorn, Kimberly M.. “Construction of a synaptic membrane for in silico endocannabinoid investigations”. 2016, https://core.ac.uk/download/345084361.pdf
- Lee, Yan Fen. “Utilizing Thiocholesterol As A Hydrophobic Probe To Characterize Lipid Phase Behaviour”. 2017, https://core.ac.uk/download/153211438.pdf
- Fischer, T., Vink, R. L. C.. “Domain formation in membranes with quenched protein obstacles: Lateral heterogeneity and the connection to universality classes”. ‘AIP Publishing’, 2010, http://arxiv.org/abs/1011.0538
- Teye-Kau, John Hayford G. “Synthesis of Phosphatidylethanolamine Lipids for Model Studies of the Cell Membrane”. Digital Commons @ East Tennessee State University, 2021, https://core.ac.uk/download/482044017.pdf
- Bell G. I., Bennedetti E. L., Bennett H. S., Berlin R. D., Beutner E. H., Bhatnagar G. M., Brackenbury R., et al.. “MOLECULAR STRUCTURE OF THE EPIDERMAL EXTRACELLULAR SPACES”. ‘Wiley’, 1979, http://deepblue.lib.umich.edu/bitstream/2027.42/65966/1/j.1365-4362.1979.tb01946.x.pdf
- Fujiwara, Takahiro K., Hirosawa, Koichiro M., Kusumi, Akihiro, Morone, et al.. “Cholesterol- and actin-centered view of the plasma membrane: updating the Singer–Nicolson fluid mosaic model to commemorate its 50th anniversary”. American Society for Cell Biology (ASCB), 2023, https://core.ac.uk/download/587263650.pdf
- Adams, Allen, Anrather, Brown, Brown, Castellana, Conner, et al.. “Neural Membrane Signaling Platforms”. ‘Information Bulletin on Variable Stars (IBVS)’, 2010, https://core.ac.uk/download/236308727.pdf
- Costa, Michelle. “Unraveling the intricacies of spatial organization of the ErbB receptors and downstream signaling pathways”. UNM Digital Repository, 2009, https://core.ac.uk/download/151575659.pdf
- Mkrtchyan, Anna. “A single cell based model for cell divisions with spontaneous topology changes”. Scholarship@Western, 2013, https://core.ac.uk/download/61641762.pdf
- Hagen, T.L.M. (Timo) ten, Zalba, S. (Sara). “Cell membrane modulation as adjuvant in cancer therapy”. ‘Elsevier BV’, 2017, https://core.ac.uk/download/154417685.pdf
- Bagatolli, Luis Alberto, Stock, Roberto. “Lipids, membranes, colloids and cells: A long view”. ‘Elsevier BV’, 2021, https://core.ac.uk/download/534244982.pdf
- Johann, Leonie Isabelle. “Facing educational challenges in molecular life science – A thesis to reconstruct cell membrane biology for upper secondary teaching and learning”. ‘MDPI AG’, 2022, https://core.ac.uk/download/523307335.pdf
- Demchenko, A.P.. “Modern views on the structure and dynamics of biological membranes”. ‘Institute of Molecular Biology and Genetics (NAS Ukraine)’, 2012,
- Baaden, Marc. “Visualizing Biological Membrane Organization and Dynamics”. Elsevier, 2025, https://core.ac.uk/download/pdf/265310456.pdf