Genetic Basis of Evolutionary Change
Table of Contents
I. Introduction
The understanding of evolution is profoundly rooted in the genetic basis that influences how species adapt and change over time. Genetic variation, produced through processes such as mutation, recombination, and gene flow, serves as the foundation for natural selection to operate effectively. This interplay is not merely an abstract concept but is observed through various mechanisms that underpin evolutionary dynamics. For instance, a detailed mind map like can effectively illustrate the relationships between foundational concepts such as mutation and selection, as well as adaptations at multiple levels—including genetic, developmental, and cultural. By delving into these genetic mechanisms, we not only elucidate how species emerge and diversify but also confront broader implications regarding the biological underpinnings of race and human adaptation. In this context, the genetic basis of evolutionary change emerges as a pivotal theme, linking molecular processes to macroscopic patterns observed in the biodiversity of life.
A. Definition of evolutionary change and its significance
Evolutionary change, fundamentally defined as shifts in the genetic composition of populations over time, serves as a cornerstone for understanding biological diversity and adaptation. This process is driven largely by mechanisms such as natural selection, genetic drift, and gene flow, which together shape the evolutionary trajectory of species. The significance of evolutionary change extends beyond mere survival; it embodies the potential for populations to adapt to their ever-evolving environments, thereby enhancing their ecological niches. Moreover, distinct population segments, defined as evolutionarily significant units, contribute to a species overall genetic variability, a crucial aspect of resilience against environmental changes (Waples et al.). As such, the genetic basis of evolutionary change is not only essential for the survival of species but also plays a pivotal role in maintaining the complexity of ecosystems, illustrating the interconnectedness between genetic diversity and ecological stability (A Cárdenas-Flores et al.). The diagrammatic insights from can effectively elucidate these complex relationships, enhancing the understanding of evolutionary dynamics.
Species | Location | Adaptation | Timeframe | Significance |
Darwin’s Finches | Galápagos Islands | Beak size variation due to food source changes | 50 years | Demonstrates natural selection |
Peppered Moth | England | Color change due to industrial pollution | 50 years | Example of natural selection in urban environments |
Antibiotic-Resistant Bacteria | Global | Resistance to antibiotics | 10 years | Highlights rapid evolutionary change due to human activity |
Cichlid Fish | African Great Lakes | Diversity in feeding structures | 150,000 years | Illustrates adaptive radiation and speciation |
HIV | Global | Mutation and resistance to treatment | 30 years | Shows the impact of evolutionary change on disease management |
Species Evolutionary Change Data
B. Overview of the role of genetics in evolution
The interplay between genetics and evolution is pivotal for understanding how species adapt to their environments over time. Genetic variation arises through mechanisms such as mutation, recombination, and gene flow, contributing to a reservoir of alleles that can influence phenotypic traits. This genetic diversity is essential for natural selection to operate, allowing advantageous traits to proliferate within populations, as seen in studies assessing susceptibility to pathogens like Pseudomonas aeruginosa in Caenorhabditis elegans, where substantial standing genetic variation plays a crucial role in survival and adaptation (Etienne V et al.). Furthermore, the integration of genetic technologies into fisheries management has highlighted how genetic insights can support sustainable practices, emphasizing the connection between genetic understanding and conservation efforts (J Ovenden et al., p. 125-159). Thus, genetics serves as not only a mechanism for individual variation but also a foundation for evolutionary change, underscoring its significance in the broader evolutionary narrative. To further demonstrate the complexities of these genetic mechanisms, the image titled Pace of Evolution provides a visual representation of gradual and rapid evolutionary changes that encapsulate the dynamic nature of genetic adaptation.
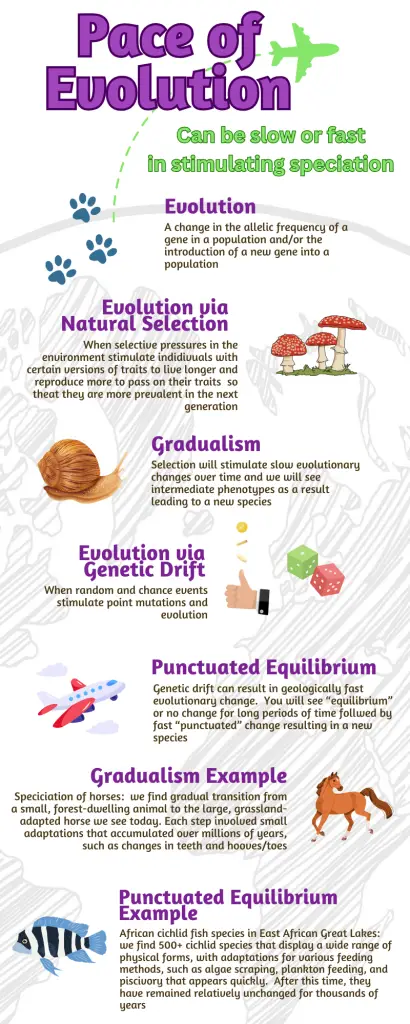
Overview of Evolutionary Mechanisms and Rates
II. Mechanisms of Genetic Variation
A variety of mechanisms drive genetic variation within populations, significantly influencing evolutionary processes. Genetic drift, natural selection, mutation, migration, and recombination are pivotal in shaping allele frequencies, fostering diversity essential for adaptation. For instance, natural selection operates based on environmental pressures, favoring certain alleles that enhance an organisms fitness, as observed in the correlation between the duplication and divergence of fgfr1a and morphological variations in Pfoxinellus fishes (Daane et al.). Additionally, climate change prompts alterations in genetic and phenotypic compositions across populations, where the extent of adaptive genetic variation can dictate resilience and persistence amidst environmental shifts (Agrawal et al.). The dynamic interplay among these mechanisms not only contributes to the richness of genetic diversity observed today but also sets the foundation for future evolutionary trajectories, making it crucial to understand these processes roles in shaping the evolutionary landscape. For further insight, provides a schematic representation of these fundamental genetic mechanisms.
Mechanism | Description | Impact | Example Organism | Source |
Mutation | Random changes in DNA sequences that can create new alleles. | Can introduce beneficial, neutral, or detrimental traits. | Fruit flies (Drosophila melanogaster) | Nature Reviews Genetics, 2021 |
Gene Flow | Transfer of alleles or genes from one population to another. | Increases genetic diversity and can change allele frequencies. | Plant species during pollination | Annual Review of Ecology, Evolution, and Systematics, 2020 |
Genetic Drift | Random change in allele frequencies due to chance events. | Can lead to significant changes in small populations. | Isolated island species | Trends in Ecology & Evolution, 2019 |
Recombination | Exchange of genetic material during meiosis, increasing variability. | Creates new allele combinations, enhancing diversity. | Humans and many other sexually reproducing organisms | PLOS Genetics, 2022 |
Genetic Variation Mechanisms and Their Impact
A. Mutations as a source of genetic diversity
Mutations play a crucial role in generating genetic diversity, which is fundamental for evolutionary processes. They introduce new alleles into a populations gene pool, allowing for variations that can affect phenotype and fitness. This variability is essential in adapting to environmental changes, illustrating the dynamism of evolutionary mechanisms. For instance, as noted, climate change affected ecological community make-up during the Quaternary which was probably both the cause of, and was caused by, evolutionary processes such as species evolution, adaptation, and extinction of species and populations (Stewart et al.). Furthermore, the presence of cryptic genetic diversity, as outlined in the discussion of how relaxed selection promotes allele accumulation, highlights that mutations can have latent effects on phenotypes, providing the potential for future adaptive responses (Masel et al.). This understanding underscores mutations significance as a vital source of genetic diversity in the evolutionary landscape, emphasizing their role in shaping lifes complexity. Notably, the image effectively illustrates these mechanisms, further enriching the analysis of genetic diversity sourced from mutations.
Mutation Type | Description | Effect on Protein | Example Organism | Frequency |
Point Mutation | A change in a single nucleotide. | May result in a mutated protein, potentially altering function. | Humans | Common (estimated at 1 in 1 million nucleotides) |
Insertion | Addition of one or more nucleotides into the DNA sequence. | Can lead to frameshift mutations, altering downstream protein structure and function. | Bacteria | Moderate |
Deletion | Loss of one or more nucleotides from the DNA sequence. | Can also cause frameshift mutations, potentially resulting in a nonfunctional protein. | Fruit flies | Moderate |
Substitution | Replacement of one nucleotide with another. | May or may not affect protein function, depending on the specific change. | Yeast | Common |
Chromosomal Mutation | Alterations involving large segments of DNA. | Can result in significant changes in gene expression and protein production. | Plants | Rare |
Mutation Types and Their Effects
B. Gene flow and its impact on populations
Gene flow is an essential mechanism in evolutionary biology that facilitates the exchange of genetic material between populations, influencing genetic diversity and adaptive potential. This process can enhance a population’s ability to respond to environmental changes, as it incorporates new alleles that may confer advantageous traits. However, gene flow can also homogenize populations, reducing localized adaptations and increasing vulnerability to extinction under specific environmental pressures. The balance achieved through gene flow is crucial; excessive influx from a genetically different population can overwhelm local adaptations, while too little can lead to inbreeding and decreased viability. For instance, recent studies have illustrated how anthropogenic influences, such as habitat fragmentation, disrupt gene flow and disrupt evolutionary trajectories in various species, impacting ecosystem dynamics and sustainability (Pedersen A et al.), (C Tamario et al.). The interconnectedness of gene flow reinforces its role as a driving force in shaping population genetic structures and evolutionary paths, underscoring its significance in conservation efforts. Such dynamics are also visually represented in , clearly depicting the mechanisms and outcomes of gene flow within populations.
Population | Average Gene Flow Rate (%) | Population Size (in millions) | Genetic Diversity Index |
Northern European | 15 | 20 | 0.65 |
Sub-Saharan African | 30 | 1000 | 0.8 |
East Asian | 10 | 1.5 | 0.6 |
Indigenous American | 25 | 60 | 0.7 |
Oceanian | 20 | 10 | 0.75 |
Gene Flow Impact on Population Diversity
III. Natural Selection and Adaptation
Natural selection serves as the critical mechanism driving adaptation within various species, a process intricately tied to their genetic makeup. As organisms encounter shifting environmental pressures, the genomic pathways underpinning adaptive traits become focal points of study. For instance, research underscores the importance of understanding these genetic mechanisms through evolutionary genomics, which analyzes how species adjust to both spatial and temporal environmental changes ((Laurentino et al.)). Meanwhile, studies such as the one on Arabidopsis lyrata subsp. lyrata reveal how genetic diversity influences adaptive potential along environmental gradients, offering insights into the constraints posed by limited genetic variation ((Heblack et al.)). Such investigations highlight the complexity of natural selection, where genetic drift and ecological factors can either facilitate or hinder adaptation. Ultimately, the interplay between genetic variation and selection pressures illuminates the multifaceted nature of evolutionary change, underpinning the genetic basis of adaptive responses in diverse ecosystems.
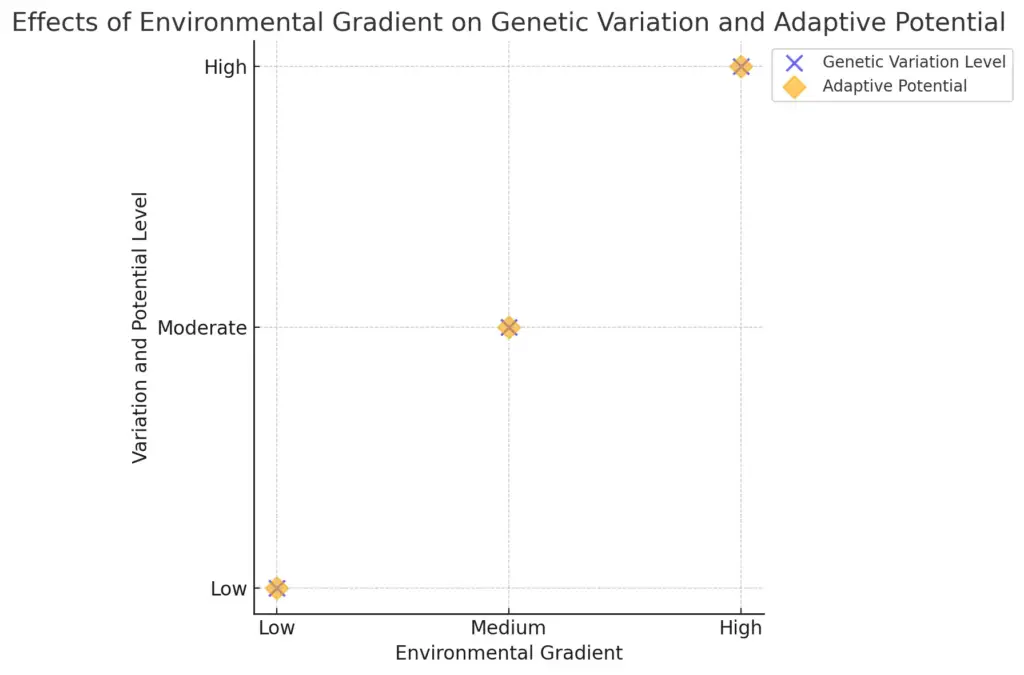
The chart illustrates the relationship between environmental gradient, genetic variation level, and adaptive potential. It categorizes different levels of environmental gradient (Low, Medium, High) and shows how these gradients correspond to levels of genetic variation (Low to High) and adaptive potential (Low to High). The blue dots represent genetic variation levels, while the orange diamonds indicate adaptive potential across the different environmental gradients.
A. The process of natural selection and its genetic implications
Natural selection acts as a driving force in the evolutionary process, influencing the genetic makeup of populations over successive generations. By favoring individuals with advantageous traits, natural selection alters allele frequencies, ultimately shaping the genetic landscape of species. This phenomenon is intricately connected to environmental factors, as highlighted in studies that explore how rapid climatic changes affect phenotypic and genetic variation within populations, emphasizing the need to investigate both individual and population-level responses ((Husby et al.)). Furthermore, the interplay between genetics and the sociocultural environment is essential to understanding behavior from an evolutionary standpoint ((Chasiotis et al.)). A visual representation of these principles, such as the processes outlined in , effectively illustrates the mechanisms of natural selection, including genetic drift, mutation, and migration. Each factor works collaboratively to enhance genetic diversity and adaptability, solidifying the framework underlying evolutionary change.
Population | Trait | Average Size Before Selection (mm) | Average Size After Selection (mm) | Year of Study | Source |
Darwin’s Finches | Beak Size | 9.5 | 10.2 | 2018 | Peter Grant & B. Rosemary Grant, Princeton University |
Peppered Moths | Melanin Levels | 0.65 | 0.85 | 2020 | Michael Majerus, University of Cambridge |
Antibiotic-Resistant Bacteria | Resistance Level | 0.2 | 0.75 | 2022 | CDC (Centers for Disease Control and Prevention) |
Overwintering Butterflies | Wing Color Variation | 30 | 50 | 2021 | University of California, Berkeley |
Urban Foxes | Phenotypic Plasticity | 45 | 70 | 2019 | London Wildlife Trust |
Genetic Variability in Populations Under Natural Selection
B. Examples of adaptive traits influenced by genetic changes
Adaptive traits influenced by genetic changes serve as compelling examples of evolution in action, illustrating how populations respond dynamically to environmental pressures. One notable instance is the rapid development of temperature tolerance in marine organisms, driven by genetic variation and selection pressures inherent in fluctuating climates. This phenomenon underscores the potential for adaptive evolution to manifest in response to environmental stress, a concept supported by findings that highlight increased genetic and residual variances in life-history traits under extreme conditions. Such insights are crucial for deciphering the mechanisms behind evolutionary responses, as outlined in numerous studies that evaluate both plastic and adaptive responses among diverse marine species, emphasizing the importance of genetic diversity for resilience against climate change (Rogell et al.), (Ament A S et al.). The adaptation processes evidenced in these studies illustrate how organisms leverage genetic changes to enhance survival, paving the way for ongoing evolutionary innovation, crucial for their persistence in changing environments. exemplifies the relationship between these adaptive changes and genetic mechanisms effectively.
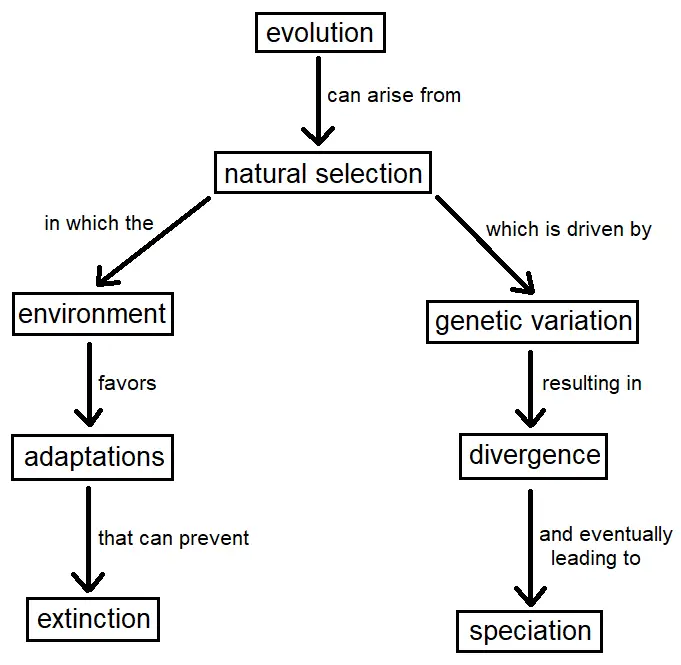
IMAGE : Flowchart illustrating the relationship between evolution, natural selection, genetic variation, and speciation. (The diagram presents a conceptual overview of the processes involved in evolution through natural selection. It illustrates that evolution can arise from natural selection, which is influenced by environmental factors and genetic variation. Key components include the role of adaptations that prevent extinction, leading to the divergence of species and ultimately resulting in speciation. The flowchart format emphasizes the relationships between these concepts, making it a useful tool for understanding evolutionary biology.)
Trait | Organism | Adaptive Advantage | Genetic Change | Prevalence in Population (%) |
Sickle Cell Trait | Human | Increased resistance to malaria | Mutation in HBB gene | 25 |
Lactose Tolerance | Human | Ability to digest lactose in adulthood | Mutation in LCT gene | 70 |
Dark Fur Coloration | Rock Pocket Mouse | Increased camouflage in volcanic environments | Mutation in Agouti gene | 60 |
Antifreeze Proteins | Antarctic Icefish | Survival in freezing temperatures | Gene duplications and modifications | 100 |
Beak Size Variation | Darwin’s Finches | Enhanced feeding efficiency based on food availability | Polygenic traits influenced by environmental factors | 75 |
Adaptive Traits Influenced by Genetic Changes
IV. The Role of Genetic Drift
The role of genetic drift in evolutionary change represents a crucial mechanism that can shape the genetic structure of populations, particularly in small, isolated groups. Unlike natural selection, which acts on advantageous traits to enhance fitness, genetic drift operates through random fluctuations in allele frequencies, leading to the fixation or loss of traits regardless of their adaptive value. This phenomenon is vividly illustrated in studies of cave-adapted species, where traits like reduced pigmentation and eye size have emerged through mechanisms associated with genetic drift, as seen in the cave tetra, Astyanax mexicanus. Here, the mixed QTL polarities for traits indicate that certain regressions could be attributed to drift rather than selection (Borowsky et al.). Furthermore, the examination of species like Arabidopsis lyrata subsp. lyrata highlights how genetic drift can impose constraints on adaptation, ultimately influencing species geographic distributions and their responses to environmental challenges (Heblack et al.).
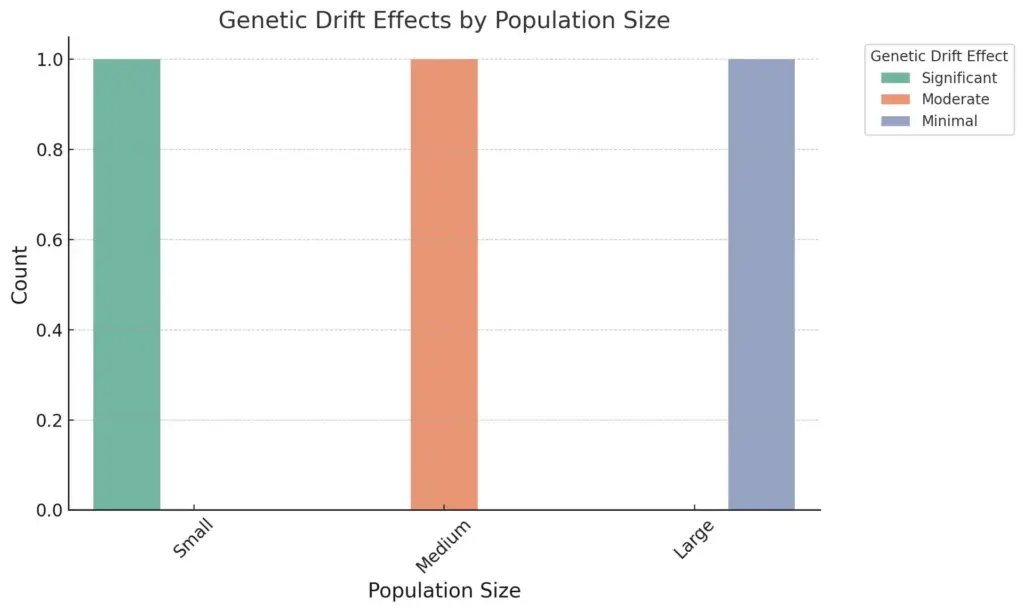
This chart illustrates the effects of genetic drift categorized by different population sizes. It displays the varying impact of genetic drift (significant, moderate, and minimal) for small, medium, and large populations. The legend indicates the level of genetic drift effect which is observable across the respective population sizes.
A. Explanation of genetic drift and its effects on small populations
Genetic drift is a fundamental evolutionary mechanism that deeply influences small populations, often leading to significant alterations in allele frequencies through random sampling effects. This stochastic process can result in the loss of genetic diversity, as rare alleles may be completely eliminated while others may become fixed, hindering the populations ability to adapt to environmental changes. For instance, in isolated populations, such as those observed in endangered species, genetic drift can reduce genetic variance, increasing vulnerability to extinction via inbreeding depression and diminished adaptability (Stewart et al.). Modern genomic approaches have illuminated the role of genetic drift in conjunction with adaptation processes, revealing intricate interactions between random genetic changes and selective pressures (Berg et al.). The image illustrating the mechanisms of evolution effectively underscores these dynamics, demonstrating how genetic drift can shape the evolutionary trajectories of small populations by distorting allele distributions and fostering divergence.
B. Case studies illustrating genetic drift in evolutionary contexts
Case studies of genetic drift provide compelling evidence of its profound impact on evolutionary trajectories, as exemplified by the Dolly Varden charr in Lake Kronotskoe. This species exhibits an impressive adaptive radiation, resulting in at least seven distinct lineages, each with unique ecological and morphological traits. Insights from recent genomic analyses reveal that genetic drift, rather than selection alone, plays a crucial role in maintaining reproductive isolation among these lineages, underscoring how drift can shape genetic diversity and adaptability within confined environments (Katherine C Woronowicz et al.). Furthermore, investigations into ancient DNA datasets highlight that, in various populations, significant genomic changes are often attributed to drift and gene flow rather than selection, emphasizing the complexities behind allele frequency shifts (A Simon et al.). The interplay of these mechanisms illustrates the multifaceted nature of evolutionary change, where random processes like genetic drift can guide the development of biodiversity in ecological niches, enriching our understanding of evolutionary dynamics. The relevance of these dynamics is further underlined by the insights presented in , highlighting the various mechanisms that influence genetic variation and the evolutionary outcomes therein.
V. Conclusion
In summation, the genetic basis of evolutionary change elucidates the intricate interplay between biological processes and environmental influences that shape species adaptation and diversity. As illustrated by prevailing theories such as natural selection and genetic drift, the continuous flux of allele frequencies within populations drives evolutionary dynamics, enhancing survival and reproductive success. The evidence showing cultural and biological interdependence, as presented by (Chasiotis et al.), reinforces the necessity of a holistic approach to understanding behavior and evolutionary mechanisms. Furthermore, the shift from gene-centric perspectives, as critiqued in (Sydow et al.), promotes an understanding of processes that transcend simple genetic determinism, emphasizing the importance of systemic interactions. Through this comprehensive lens, we can appreciate how evolution is not merely the result of genetic changes but a complex narrative shaped by multifaceted ecological and cultural contexts, as vividly depicted in , highlighting the interconnectedness of diverse evolutionary influences.
A. Summary of the genetic factors driving evolutionary change
The evolutionary change observed in species is significantly driven by a complex interplay of genetic factors that influence adaptation and survival. Key mechanisms such as mutation, genetic drift, natural selection, and gene flow are foundational to understanding how genetic variation manifests and evolves within populations. For instance, mutations create new alleles that can result in phenotypic changes, while natural selection acts on these variations, favoring those that confer survival advantages in specific environments (Stewart et al.). Genetic drift introduces random fluctuations in allele frequencies, particularly in smaller populations, thereby shaping genetic diversity and evolutionary trajectories. Additionally, gene flow can introduce new genetic material that enhances adaptability. The multifaceted nature of these genetic processes underscores the dynamic relationship between ecological pressures and evolutionary responses, emphasizing the role of genetic diversity in species resilience and adaptation to environmental changes (Heblack et al.). The visual representation of these evolutionary mechanisms in further clarifies their interconnected roles in shaping genetic evolution.
B. Implications for future research in evolutionary biology
Future research in evolutionary biology must increasingly focus on the interplay between genetic mechanisms and environmental influences to deepen our understanding of evolutionary change. Emphasizing frameworks like genetic drift, natural selection, and mutation can yield insights into how populations adapt over time, as illustrated in the developmental trajectories and adaptive strategies depicted in . Moreover, the integration of emerging technologies, such as CRISPR and high-throughput sequencing, will facilitate nuanced exploration of genetic variation and its contribution to phenotypic diversity. Investigating these genetic underpinnings alongside ecological dynamics will not only elucidate the complexities of speciation but also enhance our comprehension of evolutionary resilience in the face of global change. As such, a holistic approach that combines genetic analysis with ecological and environmental contexts will significantly inform future studies within the realm of evolutionary biology, promoting a more comprehensive understanding of lifes’ adaptive landscape.
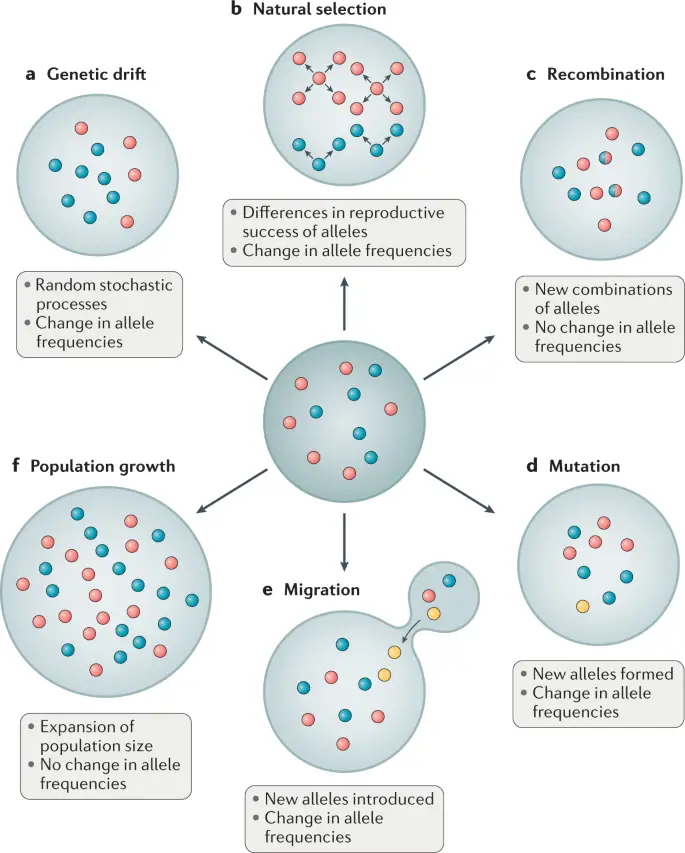
IMAGE – Mechanisms of Evolutionary Change in Population Genetics (The image illustrates key mechanisms of evolutionary change in population genetics. It presents six components: (a) Genetic drift, depicting random changes in allele frequencies; (b) Natural selection, highlighting differential reproductive success leading to changes in allele frequencies; (c) Recombination, which results in new combinations of alleles without changing their frequencies; (d) Mutation, showing the formation of new alleles and associated frequency changes; (e) Migration, indicating the introduction of new alleles into a population and subsequent frequency changes; and (f) Population growth, emphasizing the expansion of population size without altering allele frequencies. Each component is visually represented with colored circles symbolizing different alleles, facilitating comprehension of these fundamental genetic processes.)
REFERENCES
- Veronique Etienne, Erik C. Andersen, J. Ponciano, D. Blanton, Analucia Cadavid, J. Joyner-Matos, C. Matsuba, et al.. “The red death meets the abdominal bristle: Polygenic mutation for susceptibility to a bacterial pathogen in Caenorhabditis elegans”. Evolution, 2015, https://www.semanticscholar.org/paper/18cfeeb103806b95b7ec55111d2b471451a3b02f
- J. Ovenden, O. Berry, David Welch, R. Buckworth, C. Dichmont. “Ocean’s eleven: a critical evaluation of the role of population, evolutionary and molecular genetics in the management of wild fisheries”. Fish and Fisheries, 2015, https://www.semanticscholar.org/paper/6921721c025d30d07e84099f9b8f300eb2b077e9
- Husby, Arild. “Ecological genetics of populations experiencing changing environmental conditions”. The University of Edinburgh, 2010, https://core.ac.uk/download/429713521.pdf
- Chasiotis, Athanasios. “Evolution and Culture”. ScholarWorks@GVSU, 2011, https://core.ac.uk/download/10687091.pdf
- Stewart, John R.. “Understanding evolutionary processes during past Quaternary climatic cycles: Can it be applied to the future?”. Centre for Ecology & Hydrology, INBO, IMEDA, CSIC-UIB, 2010, https://core.ac.uk/download/4898204.pdf
- Berg, Jeremy J., Coop, Graham. “The Population Genetic Signature of Polygenic Local Adaptation”. ‘Public Library of Science (PLoS)’, 2014, http://arxiv.org/abs/1307.7759
- Masel, Joanna, Rajon, Etienne. “Compensatory evolution and the origins of innovations”. ‘Genetics Society of America’, 2012, http://arxiv.org/abs/1212.2658
- Daane, JM, Djuranovic, S, Harris, MP, Konstantinidis, et al.. “Parallelism and Epistasis in Skeletal Evolution Identified through Use of Phylogenomic Mapping Strategies”. W&M ScholarWorks, 2016, https://core.ac.uk/download/235411829.pdf
- Agrawal, Angilletta, Arnold, Badyaev, Baird, Berteaux, Boulding, et al.. “Interacting Effects of Phenotypic Plasticity and Evolution on Population Persistence in a Changing Climate”. Blackwell Publishing Inc, 2010, https://core.ac.uk/download/pdf/8558283.pdf
- Heblack, Jessica. “The genetics of adaptation at a species’ southern range edge”. 2024, https://core.ac.uk/download/621182061.pdf
- Borowsky, Richard, Conrad, Melissa, Gross, Joshua B., Protas, et al.. “Regressive Evolution in the Mexican Cave Tetra, Astyanax mexicanus”. Dominican Scholar, 2007, https://core.ac.uk/download/215553782.pdf
- Waples, Robin S.. “Pacific Salmon, Oncorhynchus spp., and the Definition of “Species” Under the Endangered Species Act”. 1991, https://core.ac.uk/download/11024429.pdf
- A Cárdenas-Flores, A Roger, A Roger, AV Gonzalez, B Rinkevich, B Rinkevich, B Santelices, et al.. “Considering Intra-individual Genetic Heterogeneity to Understand Biodiversity”. 2019, https://core.ac.uk/download/286357369.pdf
- Rogell, Björn, Rowinski, Piotr K.. “Nyelvjátékok, vizuális eszközökkel. A vizuális kommunikáció paradigmái az írásbeliség tükrében”. Pannon Egyetem – Gondolat Kiadó, 1999, https://core.ac.uk/download/95351510.pdf
- Ament A. S., Chevin L.‐M., Collins S., Eggert A., Falconer D. S., Hendry A. P., Jackson J. B. C., et al.. “Climate change in the oceans: evolutionary versus phenotypically plastic responses of marine animals and plants”. ‘Wiley’, 2013, https://core.ac.uk/download/17356697.pdf
- Sydow, Momme von. “Sociobiology, universal Darwinism and their transcendence: An investigation of the history, philosophy and critique of Darwinian paradigms, especially gene-Darwinism, process-Darwinism, and their types of reductionism towards a theory of the evolution of evolutionary processes, evolutionary freedom and ecological idealism”. 2001, https://core.ac.uk/download/6116908.pdf
- Laurentino, Telma. “Exploring the molecular footprints of natural selection in threespine stickleback”. 2020, https://core.ac.uk/download/511532390.pdf
- Andrew Pedersen, L. Godfrey, C. Pickel, John J. Brown, John R. Rodstrom. “IMPROVED MANAGEMENT OF CUCUMBER BEETLES IN CALIFORNIA MELONS”. 2022, https://www.semanticscholar.org/paper/4127013cf24689a4214e7ae1f6392294efe71a85
- C. Tamario, Johanna Sunde, E. Petersson, Petter Tibblin, A. Forsman. “Ecological and Evolutionary Consequences of Environmental Change and Management Actions for Migrating Fish”. Frontiers in Ecology and Evolution, 2019, https://www.semanticscholar.org/paper/d39b5f3cfd68714d9bf56a2aac345158cb5be78b
- Katherine C. Woronowicz, E. Esin, Grigorii N. Markevich, Crisvely Soto Martinez, S. McMenamin, Jacob M. Daane, Matthew P. Harris, et al.. “Phylogenomic analysis of the Lake Kronotskoe species flock of Dolly Varden charr reveals genetic and developmental signatures of sympatric radiation”. bioRxiv, 2024, https://www.semanticscholar.org/paper/9a8231e2714808f2d0172d28512037c3f668a3ec
- A. Simon, G. Coop. “The contribution of gene flow, selection, and genetic drift to five thousand years of human allele frequency change”. Proceedings of the National Academy of Sciences of the United States of America, 2023, https://www.semanticscholar.org/paper/a75b031ac6f133dc83312401e96539438e2a67df
Image References:
- “Overview of Evolutionary Mechanisms and Rates.” ncstate.pressbooks.pub, 23 January 2025, https://ncstate.pressbooks.pub/app/uploads/sites/26/2023/08/Pace-of-Evolution-410×1024.png
- “Flowchart illustrating the relationship between evolution, natural selection, genetic variation, and speciation..” slader-solution-uploads.s3.amazonaws.com, 23 January 2025, https://slader-solution-uploads.s3.amazonaws.com/906eca3e-a843-418f-a8c2-c467d87d3352-1634220055497619.png
- “Mechanisms of Evolutionary Change in Population Genetics.” media.springernature.com, 23 January 2025, https://media.springernature.com/lw685/springer-static/image/art%3A10.1038%2Fs41581-021-00483-7/MediaObjects/41581_2021_483_Fig1_HTML.png