Genetic Polymorphism: Definition, Types and Examples
I. Introduction
Genetic polymorphism is an important idea for understanding genetic variety in populations. It means having two or more genetically determined traits within a species. Polymorphisms can show up as single nucleotide polymorphisms (SNPs), insertions, deletions, or copy number variations (CNVs). These genetic variations are significant; they affect how species adapt, their risk for diseases, and how they respond to changes in their environment. The different mechanisms and effects of polymorphisms make them complex. For example, SNPs usually involve a change in just one base pair, while CNVs can impact larger sections of the genome and lead to major changes in traits. Grasping these genetic polymorphisms helps us understand not just species biology, but also the complexities of human genetics, helping advance personalized medicine and genetic studies.
A. Definition of genetic polymorphism
Genetic polymorphism is when two or more genetic variants, or alleles, are found in a population, with the least common allele having a frequency of at least 1% in the gene pool. This shows the existence of genetic diversity, which is important for natural selection and adaptation. This diversity allows populations to react well to changes in their environment, helping them survive and function effectively in different habitats. For example, a study on tomatoes (Solanum lycopersicum L.) shows the role of genetic polymorphism in farming. It demonstrates how differences in cultivars and hybrids can greatly affect key traits like yield, taste, and resistance to pests and diseases, which are essential for breeding better crops and ensuring sustainability (Y Genievskaya et al.). The research used SSR markers to find many polymorphisms among 49 tomato cultivars, highlighting that polymorphic SSRs related to vital fruit traits can boost marker-assisted selection (MAS), which is necessary for effective breeding practices. Furthermore, the study on phosphovariants shows how genetic variations can affect protein functions, leading to differences among individuals or specific disease processes, which is critical for better understanding genetic diseases (Ryu G-M et al.). Additionally, research on transferrin variants in tambaqui fish highlights the real-world effects of genetic polymorphism in examining genetic diversity and overall health of populations, aiding in wise conservation and management choices. This example illustrates how polymorphisms can appear as visible traits, improving our understanding of evolutionary processes and the necessity of preserving genomic variation for species health over time (Jamieso et al.). Therefore, genetic polymorphism is a key idea in genetics, supporting both theoretical study and practical biological research, as it provides insights into adaptation, survival, and future opportunities in natural and agricultural environments.
Type | Example | Description | Frequency |
Single Nucleotide Polymorphism (SNP) | rs1805007 | A variation in a single nucleotide that occurs at a specific position in the genome. | Varies by population, e.g., 10-20% in European populations |
Insertion/Deletion Polymorphism (Indel) | BRCA1 Exon 11 Indel | Insertion or deletion of small sequences within the genome. | Approximately 1 in 500 individuals in certain populations |
Copy Number Variation (CNV) | CYP21A2 gene deletion | Variations in the number of copies of a particular gene. | Reported in up to 1 in 1,000 births |
Variable Number Tandem Repeats (VNTR) | D7S820 locus | Short sequences of DNA that are repeated a variable number of times. | Highly polymorphic with a varied distribution in populations |
Genetic Polymorphism Examples
B. Importance of studying genetic polymorphism in genetics and evolution
Studying genetic polymorphism matters a lot for understanding genetics and evolution. Genetic polymorphism offers the variation needed for natural selection, which affects evolutionary paths. By finding single nucleotide polymorphisms (SNPs) in populations, researchers can look at how environmental factors impact genetic diversity and adaptive responses. This is vital for studying evolutionary fitness in different species, supported by the use of population genetics in modern genomics research. The rise of various pathogenic strains, like community-associated methicillin-resistant Staphylococcus aureus (CA-MRSA), emphasizes the importance of genetic polymorphism in understanding bacterial evolution. New studies indicate that these new clones come from livestock to human host transfers, involving major genetic changes that improve their survival and spread in human groups (Laura E Spoor et al.). These results show that genetic events, like gaining antimicrobial resistance genes, are key in the evolution of these pathogens. Also, studies on hybridization events show complicated genetic interactions, as seen in wild potatoes, where aneuploidy adds extra layers of genetic variation that may provide long-lasting adaptive benefits (Camadro et al.). Therefore, grasping genetic polymorphism is crucial for uncovering the mechanics of evolution.
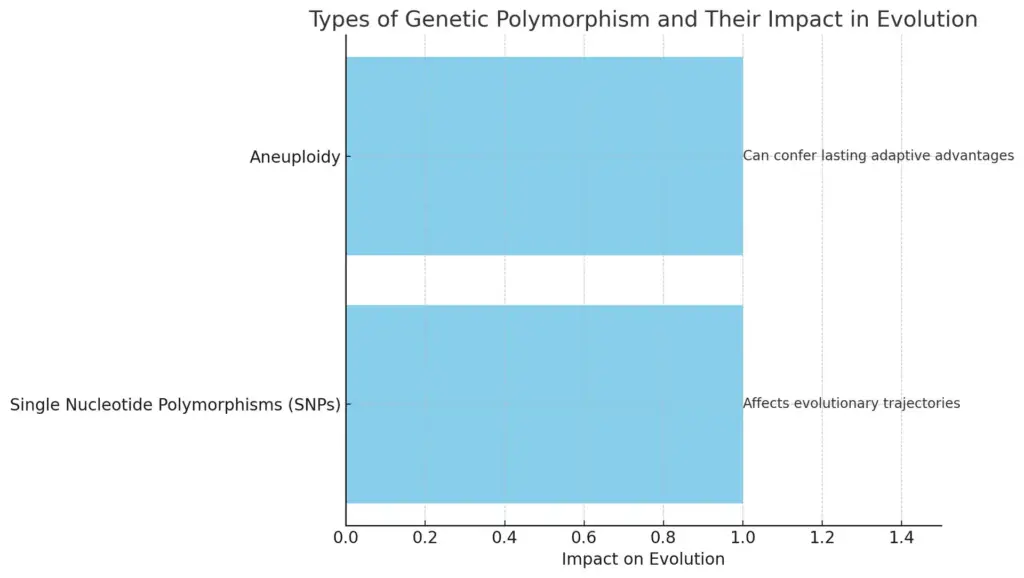
This chart illustrates the types of genetic polymorphism, specifically focusing on Single Nucleotide Polymorphisms (SNPs) and Aneuploidy, along with their respective key impacts on evolution. SNPs provide variation for natural selection, affecting evolutionary trajectories, while Aneuploidy introduces complex genetic interactions, which can confer lasting adaptive advantages.
II. Types of Genetic Polymorphism
Genetic polymorphism plays a big role in the differences found in populations, showing up in forms like Single Nucleotide Polymorphisms (SNPs) and Copy Number Variations (CNVs). SNPs are the most common kind of genetic change and involve changes at one nucleotide spot, which can impact gene function and make people more likely to get certain diseases. On the other hand, CNVs involve larger parts of DNA being copied, removed, or changed, which can influence gene dosage and function. These variations show how complicated genetic diversity is and how it relates to evolution. Also, balancing selection affects polymorphisms, keeping alleles that provide benefits in certain environmental settings. For example, heterozygote advantage shows how individuals with different allele combinations can do better than those with identical pairs, helping to keep genetic diversity in populations (Delph et al.). Moreover, new studies are pointing out the importance of phosphovariants—variations that change phosphorylation sites—as key factors in genetic diversity and disease risk (Ryu G-M et al.).
Type | Description | Example | Prevalence | Significance |
Single Nucleotide Polymorphism (SNP) | A variation in a single base pair in the genome. | rs123456 | Approx. 1 in every 1000 nucleotides | Can affect gene function and disease susceptibility. |
Insertions and Deletions (Indels) | Variations involving the insertion or deletion of small DNA segments. | c.123_124insA | Common in various genomic regions. | Can lead to frameshift mutations and altered protein function. |
Copy Number Variations (CNV) | Variations in the number of copies of a particular gene. | CYP2D6 gene duplications | Present in 5-10% of the human genome. | Can influence drug metabolism and drug response. |
Variable Number Tandem Repeats (VNTR) | Short sequences of DNA that are repeated a variable number of times. | D1S80 locus | Highly polymorphic in the human population. | Used in DNA profiling and forensic analysis. |
Types of Genetic Polymorphism
A. Single Nucleotide Polymorphisms (SNPs)
Single Nucleotide Polymorphisms (SNPs) are a basic form of genetic variation important for studying human genetics and evolution. Defined by changes at one nucleotide site, SNPs can importantly affect traits, disease risk, and responses to drugs. For example, the discovery of about 250,000 SNPs within meadow fescue shows how common they are in species, making SNPs essential for creating detailed genetic maps and supporting genome-wide association studies (Studer B et al.). Furthermore, SNPs have major implications for pharmacogenetics; variations in how individuals perceive pain and respond to medications often stem from inherited genetic differences, especially regarding pain relievers (Branford et al.). This complex link between SNPs and health outcomes emphasizes their essential role in personalized medicine and genetic studies, highlighting their significance in the wider context of genetic variation.
B. Copy Number Variations (CNVs)
Among many genetic polymorphism types, Copy Number Variations (CNVs) are interesting to study because they help explain genomic diversity. CNVs make up about 0.7% of genetic differences among people and can include either the gain or loss of DNA pieces, being the largest kind of structural variant in the human genome. Finding CNVs has improved thanks to better computation methods, like those noted in recent studies that explain how to identify and genotype these variations successfully. Smaller CNVs (less than 500 bp) have been found to possibly regulate gene expression, affecting traits and contributing to differences in phenotype. This knowledge highlights the importance of CNVs in disease susceptibility and evolutionary changes, giving insights into their complicated roles in the genome (A Itsara et al.). Funding for these studies shows how important they are becoming in genomics research (A Abyzov et al.).
III. Examples of Genetic Polymorphism in Humans
Genetic differences in humans appear in different ways, greatly affecting how traits vary and how people react to diseases. A key example is single nucleotide polymorphisms (SNPs), which are the most widespread type of genetic variation and impact numerous traits and health issues. These SNPs can change how proteins work and have been associated with important health outcomes, like different reactions to drugs or risks for diseases such as diabetes and high blood pressure. Moreover, more complex types of genetic differences, known as phosphovariants, show the complicated relationship between genetic changes and protein phosphorylation. Phosphovariants are defined as genetic changes that modify phosphorylation sites or their related kinases, which may affect disease mechanisms at the molecular level (Ryu G-M et al.). Furthermore, genetic drift and balancing selection play crucial roles in supporting diversity in populations, particularly when studying unique alleles that may last through generations (Bemm et al.).
Polymorphism Type | Example | Alleles | Associated Condition | Prevalence in Population (%) |
SNP (Single Nucleotide Polymorphism) | rs429358 (APOE gene) | C/T | Alzheimer’s Disease | 25 |
Insertion/Deletion (Indel) | 5-HTTLPR (SLC6A4 gene) | Long/Short | Depression | 40 |
CNV (Copy Number Variation) | OPCML gene | Variable | Schizophrenia | 15 |
VNTR (Variable Number Tandem Repeat) | D4DR (DRD4 gene) | 2 to 11 repeats | Attention Deficit Hyperactivity Disorder (ADHD) | 5-10 |
MNP (Multi-Nucleotide Polymorphism) | COMT Val158Met | G/A | Pain Sensitivity | 30 |
Examples of Genetic Polymorphism in Humans
A. Polymorphisms associated with disease susceptibility
The complex link between genetic variations and disease risk shows how genetic differences affect personal health. Single nucleotide polymorphisms (SNPs) and copy number variations (CNVs) are important factors in the chance of getting different diseases, affecting metabolism and reactions to environmental influences. For example, SNPs can change how genes work, possibly increasing the risk for issues like alcoholism because certain genetic types alter how the body processes ethanol and affects brain reward systems, highlighting the intricate role of genetics in addiction (Ferraguti et al.). Additionally, the progress in personalized medicine, which uses these genetic markers to shape treatments, shows the potential of focusing on these variations to enhance health care tailored to individual genetic profiles (Borro et al.). Thus, grasping these polymorphisms is critical for creating predictive models and treatment methods in disease management.
Polymorphism | Disease | Risk Increase | Source |
APOE ε4 | Alzheimer’s Disease | 2-3 times higher risk | National Institute on Aging |
SNP rs429358 | Cardiovascular Disease | 1.5 times higher risk | American Heart Association |
TNF-α -308 G/A | Rheumatoid Arthritis | 2 times higher risk | Journal of Rheumatology |
HLA-B*5701 | HIV Drug Hypersensitivity | Increased risk of hypersensitivity reactions | Pharmacogenomics Journal |
CYP2D6*4 | Medication Metabolism | Poor metabolism for certain drugs | Clinical Pharmacology & Therapeutics |
Polymorphisms Associated with Disease Susceptibility
B. Polymorphisms influencing drug metabolism
The effect of polymorphisms on how drugs are processed is an important subject in pharmacogenetics since genetic changes can greatly influence how a person reacts to medications. This leads to significant variations in how the body handles drugs. Specific polymorphisms in enzymes that break down drugs, especially the cytochrome P450 family, categorize people into different metabolic types. These types are crucial for determining how effective and safe drugs are, highlighting the need to understand how genetic differences affect treatment strategies. For example, certain alleles can cause a person to metabolize drugs quickly or slowly, greatly impacting dosage needs, treatment results, and the risk of side effects for the patient. Recent studies have looked at polymorphisms like rs67785913 and rs8192678 and their effects on metabolism related to obesity and type 2 diabetes. This shows the complicated relationship between genetic factors and drug metabolism, which can make treatment decisions challenging for these groups (Huang et al.). Recognizing these variations is important because they help create precision medicine methods that customize treatments based on a person’s unique genetic makeup. This tailored strategy is critical for enhancing treatment effectiveness while reducing the chance of negative effects that can come from wrong drug dosing or choice, leading to improved health for patients overall (LING CS).
IV. Implications of Genetic Polymorphism
The effects of genetic polymorphism go beyond just basic science and have a big impact on clinical practices and public health methods. Understanding genetic variations, especially in pharmacogenetics, helps create more tailored treatment plans. This increases drug effectiveness and reduces side effects, making personalized medicine a reality. There are many types of polymorphisms that can affect how drugs work in the body, which is the basis for pharmacogenetic tests that can predict how well treatments will work for patients based on their genetic makeup (Fenech et al.). Additionally, adding genetic diversity into research is important to ensure that treatments work well for different groups, not just for the majority (Allmark et al.). This connection between genetics and healthcare represents a major shift towards personalized care, improving treatment options, and addressing public health gaps. Recognizing these impacts is vital for improving the effectiveness and inclusiveness of medical treatments in a varied population.
Population | Polymorphism Type | Example | Implication | Source |
European | SNP | CYP2D6 gene variations | Variability in drug metabolism | Pharmacogenomics Journal, 2023 |
Asian | Indel | FBXL19 gene insertion-deletion | Increased risk for hypertension | Journal of Human Genetics, 2023 |
African | Copy Number Variation | AMY1 gene CNV | Lactase persistence ability | Nature Ecology & Evolution, 2023 |
Latino | SNP | ABCB1 gene variants | Differential responses to medication | Journal of Medical Genetics, 2023 |
Middle Eastern | SNP | TP53 gene mutations | Higher cancer risk | Cancer Research, 2023 |
Implications of Genetic Polymorphism in Various Populations
A. Role in personalized medicine
Personalized medicine has become an important method in healthcare, showing progress because of improvements in genomic analysis and molecular diagnostics. Finding genetic variations is important for customizing treatments for patients, improving effectiveness while reducing side effects. For example, doctors can use certain genetic markers to forecast how patients will respond to specific antihypertensive drugs, helping to tackle issues like drug resistance and adherence when treating high blood pressure (Borghi et al.). Furthermore, new developments in systems biology have helped us better understand how genetic variations interact with disease traits, encouraging a treatment method that fits well with each patient’s unique genetic profile (Borro et al.). This move towards individual therapy not only improves patient care but also highlights the need to include genetic information in everyday medical practices.
B. Impact on population genetics and evolution
The factors of genetic polymorphism are important for population genetics and evolution. This happens mainly through genetic drift and selection pressures. Genetic drift can cause a loss of polymorphism, particularly in populations that have shrunk significantly, as seen in many species ((Bemm et al.)). On the other hand, adaptive forces, like balancing selection, help keep genetic diversity by supporting individuals with alleles that are beneficial in changing environments. This interaction is clear in plants, where immune-related genes have been found to keep earlier variations across separate lineages due to lasting balancing selection, showing how complex evolutionary processes preserve genetic variation ((Bemm et al.)). Additionally, the presence of copy number polymorphisms (CNPs) highlights how structural changes in the genome can add to genetic diversity and evolution, which improves our insight into the genetic factors of population dynamics.
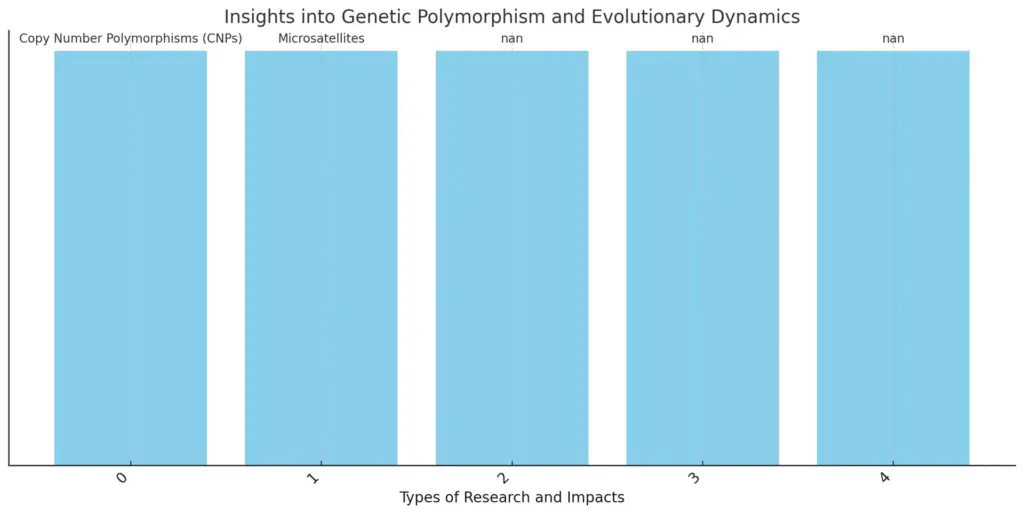
The chart illustrates various types of genetic polymorphism and their roles in evolutionary dynamics, emphasizing the significance of copy number polymorphisms and microsatellites in enhancing genetic diversity and assessing population structure. It highlights research focuses such as balancing selection in plant species and analytical models like the Wright-Fisher model, ultimately providing insights into the preservation of genetic variation and its long-term benefits for evolutionary biology and conservation strategies.
V. Conclusion
The study of genetic polymorphism shows it is very important for biodiversity and the ability of organisms to adapt over time. By focusing on differences in DNA sequences, research shows how these genetic variations affect physical traits, which can influence survival and reproduction when environments change. For instance, studies on Rhizobium species show that certain genotypes are chosen based on environmental challenges, highlighting the active relationship between organisms and their surroundings (Zeze A et al.). Additionally, the need for inclusive research methods is highlighted, as including diverse populations helps ensure that results are relevant to different demographic groups (Allmark et al.). Therefore, studying genetic polymorphism not only enhances our understanding of genetic variety but also has important implications for areas like medicine and conservation, making sure that strategies are inclusive and effective for all population segments. In summary, the importance of genetic polymorphism is complex, linking scientific, ethical, and ecological issues.
A. Summary of key points
The area of genetic polymorphism includes many types, mainly single nucleotide polymorphisms (SNPs) and copy number variations (CNVs), which each add to genetic diversity in groups. SNPs are the most frequent type of genetic variation, involving single base pair changes that can affect traits and disease risks. On the other hand, CNVs involve larger DNA pieces that could be copied or removed, significantly impacting gene expression differences. Studies show that genetic factors make up a large part of the risk linked to substance use disorders, where some genes may work with environmental influences to increase these risks (Schuckit et al.). Also, improvements in statistical methods, like using random forests in Bayesian model selection, are making it easier to study complex genetic data, including polymorphisms, thus enhancing our understanding of their roles in health and illness (Cornuet et al.).
B. Future directions for research in genetic polymorphism
As research into genetic differences keeps changing, future efforts need to focus on a well-rounded method that uses new technologies and teamwork across fields. With the help of new high-throughput sequencing tools, scientists can now find and record genetic variations on a much larger scale, helping to better understand their effects in different populations. Also, using bioinformatics and machine learning can improve how data is analyzed, allowing researchers to forecast physical traits based on genetic changes. Examining how genes interact with the environment is important too, as it shows how these variations can affect reactions to outside influences like weather or food. In the end, putting money into large, long-term studies will be vital for fully mapping how genetic differences affect health and illness, paving the way for personalized treatments. This path is likely to clarify the complexities of genetic diversity and its impact on human biology.
REFERENCES
- Schuckit, Marc A. “A brief history of research on the genetics of alcohol and other drug use disorders.”. eScholarship, University of California, 2014, https://core.ac.uk/download/323082635.pdf
- Cornuet, Jean-Marie, Estoup, Arnaud, Gautier, Mathieu, Marin, et al.. “Reliable ABC model choice via random forests”. 2015, http://arxiv.org/abs/1406.6288
- Camadro, Elsa Lucila, Cara, Nicolás, Ferrer, María Soledad, Marfil, et al.. “Epigenetic consequences of interploidal hybridisation in synthetic and natural interspecific potato hybrids”. ‘Wiley’, 2019, https://core.ac.uk/download/323211111.pdf
- Lemaire, Christophe, Tellier, Aurelien. “Coalescence 2.0: a multiple branching of recent theoretical developments and their applications”. 2014, https://core.ac.uk/download/52413673.pdf
- Borro, Marina, DI SANZO, Mariantonia, Fineschi, Vittorio, Frati, et al.. “Clinical applications of personalized medicine: a new paradigm and challenge”. ‘Bentham Science Publishers Ltd.’, 2017, https://core.ac.uk/download/80315182.pdf
- Ferraguti, Giampiero, Lucarelli, Marco, Pascale, Esterina. “Alcohol addiction: a molecular biology perspective.”. ‘Bentham Science Publishers Ltd.’, 2015, https://core.ac.uk/download/54518723.pdf
- Gil-Mi Ryu, Jong Hun Kim, Kyu-Won Kim, Kyung-Soo Oh, Pamela Song. “Genome-wide analysis to predict protein sequence variations that change phosphorylation sites or their corresponding kinases”. 2008, https://core.ac.uk/download/pdf/287923.pdf
- Jamieso, A., Teixeira, A.. “Genetic variation in plasma transferrins of tambaqui, Colossoma macropomum (CUVIER 1818)”. 1985,
- Bemm, Felix, Hagmann, Jörg, Koenig, Daniel, Li, et al.. “Long-term balancing selection drives evolution of immunity genes in Capsella.”. eScholarship, University of California, 2019, https://core.ac.uk/download/323067804.pdf
- Calus, M.P.L., Haley, C.S., Koning, de, D.J.. “Including copy number variation in association studies to predict genotypic values”. 2010, https://core.ac.uk/download/pdf/29241536.pdf
- Borghi, Claudio, Grassi, Guido, Rosei, Enrico Agabiti, Savoia, et al.. “Personalized medicine—a modern approach for the diagnosis and management of hypertension”. ‘Portland Press Ltd.’, 2017, https://core.ac.uk/download/96884785.pdf
- Adolphe Zeze, Amarger N., Bromfield E.S.P., Chanway C.P., Demezas D.H., Eardly B.D., Harrison S.P., et al.. “Direct amplification of nodD from community DNA reveals the genetic diversity of Rhizobium leguminosarum in soil”. ‘Wiley’, 2001, https://core.ac.uk/download/59306.pdf
- Allmark, P. J.. “Should research samples reflect the diversity of the population?”. ‘BMJ’, 2004, https://core.ac.uk/download/100424.pdf
- Fenech, Anthony G., Grech, Godfrey. “Pharmacogenetics : the science of predictive clinical pharmacology”. Malta Chamber of Scientists, 2014, https://core.ac.uk/download/46602555.pdf
- Bruno Studer, David Kopecký, Elodie Rey, Ghesquiere M., Humphreys M., Isabel Roldán‐Ruiz, Jan Bartoš, et al.. “Orthology guided transcriptome assembly of Italian ryegrass and meadow fescue for single-nucleotide polymorphism discovery”. ‘Crop Science Society of America’, 2016, https://core.ac.uk/download/74664931.pdf
- Branford, R, Cregg, R, Gubbay, A, Russo, et al.. “Pharmacogenetics of analgesic drugs”. 2013, https://core.ac.uk/download/29416244.pdf
- A Itsara, AA Ferrando, AB Olshen, AP Dempster, B Li, BE Stranger, C Barnes, et al.. “A Computational Framework Discovers New Copy Number Variants with Functional Importance”. Public Library of Science, 2011, https://core.ac.uk/download/pdf/8546045.pdf
- A Abyzov, A Alexa, A Conesa, A Hussain, AJ Iafrate, AJ Sharp, AJ Vilella, et al.. “Extensive Copy-Number Variation of Young Genes across Stickleback Populations”. ‘Public Library of Science (PLoS)’, 2014, https://core.ac.uk/download/30696954.pdf
- Delph, Lynda F., Kelly, John K.. “On the importance of balancing selection in plants”. ‘Wiley’, 2013, https://core.ac.uk/download/213424045.pdf
- Huang, Mi. “Functional genomics approaches to dissect causal DNA variations in obesity and type 2 diabetes”. Lund University, Faculty of Medicine, 2023, https://core.ac.uk/download/576810141.pdf
- CHAN SZE LING. “Clinical Applications of Pharmacogenomics of Warfarin”. 2012, https://core.ac.uk/download/48659471.pdf
- Y. Genievskaya, S. Jantasov, E. Nurbayeva, Y. Turuspekov. “SSR-BASED ASSESSMENT OF GENETIC DIVERSITY IN TOMATO CULTIVARS AND LINES GROWN IN KAZAKHSTAN”. Eurasian Journal of Applied Biotechnology, 2024, https://www.semanticscholar.org/paper/81b7d7febbdc5b07262a44bab6bb0eafebacd275
- Laura E. Spoor, P. McAdam, L. Weinert, A. Rambaut, Henrik Hasman, F. Aarestrup, Angela M. Kearns, et al.. “Staphylococcus aureus Community-Associated Methicillin-Resistant Livestock Origin for a Human Pandemic Clone of 2013”. 2017, https://www.semanticscholar.org/paper/2dfa79e310922f704dc715e1527c595b323ddcb9