How Bacteria and Viruses Reproduce: Comparing Binary Fission and Viral Replication
Table of Contents
I. Introduction to Microbial Reproduction
Microbial reproduction is a critical aspect of microbiology, encompassing the diverse mechanisms by which microscopic organisms such as bacteria and viruses replicate. Understanding the differences in reproduction between these two groups is fundamental to grasping their respective roles in ecosystems and their impacts on human health. Bacteria primarily reproduce through binary fission, a process characterized by a singular cell dividing into two identical daughter cells, as illustrated in . This asexual reproduction allows for rapid population growth under favorable conditions, making bacteria highly adaptable and resilient. Conversely, viruses depend on host cells for replication, utilizing a complex process that can involve stages of attachment, entry, and assembly, culminating in the release of new viral particles. The implications of these reproductive strategies extend beyond individual organisms, influencing patterns of infection, the evolution of resistance, and the dynamics of microbial populations. Such distinctions in reproduction highlight the intricate balance of microbial life forms within their environments.
Method | Organism Type | Description | Reproduction Time | Examples |
Binary Fission | Bacteria | A form of asexual reproduction where a single cell divides into two identical cells. | Typically 20 minutes to several hours | Escherichia coli, Bacillus subtilis |
Viral Replication | Viruses | The process by which a virus replicates by hijacking the host cell’s machinery. | From several hours to days, varies by virus type | Influenza virus, HIV |
Budding | Yeasts (Eukaryotic Microbes) | A form of asexual reproduction where a new cell develops from a protrusion on the parent cell. | About 2 hours | Saccharomyces cerevisiae |
Microbial Reproduction Methods Comparison
A. Why Reproduction is Essential for Microbial Survival
Reproduction is a fundamental aspect of microbial survival, as it directly influences genetic diversity, population dynamics, and adaptability to environmental changes. In bacteria, the process of binary fission permits rapid multiplication, allowing populations to expand quickly under favorable conditions. This asexual reproduction not only ensures a continuous supply of genetically identical organisms, crucial for exploiting available resources, but also provides opportunities for genetic variation through mechanisms such as horizontal gene transfer. Conversely, viruses rely on host cells to replicate, often modifying their genetic material to adapt and overcome host defenses. This reliance on host interactions highlights a different, yet equally vital, reproductive strategy that underpins viral survival. The contrasting methods of reproduction in bacteria and viruses underscore the dynamic capabilities of these microorganisms in response to environmental pressures, as seen in , which depicts the binary fission process central to bacterial reproduction. Understanding these distinctions illuminates the crucial role that reproduction plays in microbial ecology and evolution.
Process | Organism Type | Reproduction Rate (per hour) | Environment | Duration Before Reproduction (minutes) |
Binary Fission | Bacteria | 1-3 | Favorable | 20-30 |
Viral Replication | Viruses | 1000-100,000 | Host-dependent | 1-24 |
Conjugation | Bacteria | Variable | Requires direct contact | 1-2 |
Transduction | Bacteria (via viruses) | Variable | Requires a bacteriophage | 1-2 |
Microbial Reproduction Data
B. Key Differences Between Bacterial and Viral Reproduction
The differences in reproduction between bacteria and viruses are fundamental to understanding their biological characteristics and implications for healthcare. Bacteria reproduce asexually primarily through a process known as binary fission, where a single cell copies its genetic material and divides into two identical daughter cells, a mechanism illustrated in . This process is efficient and allows for rapid population growth under favorable conditions. In contrast, viruses cannot replicate independently; they require a host cell to reproduce. Viral replication involves hijacking the host cell’s machinery, resulting in the production of new virions that eventually emerge to infect additional cells, as highlighted in the discussions about viral infections. Notably, this distinction underlines the complexities associated with treating bacterial versus viral infections, as antibiotics can target bacterial processes effectively, whereas antiviral treatments are required for viral diseases, making the understanding of these reproductive methods crucial in both medical research and practical applications in healthcare.
Reproduction Type | Organism Type | Process Description | Time Taken | DNA Replication | Cellular Structures |
Binary Fission | Bacteria | Asexual reproduction where a single cell divides into two identical daughter cells. | Typically 20 minutes to several hours, depending on the species and environmental conditions. | Occurs before the division process; genes are copied. | Bacteria are prokaryotic, lack membrane-bound organelles. |
Viral Replication | Viruses | Viruses hijack host cells to replicate; they require a living host for reproduction. | Varies widely based on the virus and host; generally ranges from a few hours to several days. | Viral genetic material is replicated using the host’s cellular machinery. | Viruses are acellular, lack cellular structures and metabolism. |
Comparison | Both | Both involve genetic material replication but differ in dependence on host cells. | Bacterial reproduction is self-sufficient; viral replication depends on host availability. | Bacteria replicate their own DNA; viruses rely on host cells to replicate. | Bacteria can function independently; viruses require a host cell. |
Key Differences Between Bacterial and Viral Reproduction
II. How Bacteria Reproduce
Bacterial reproduction primarily occurs through aprocess known as binary fission, a highly efficient mechanism that enables rapid population growth. During binary fission, a single bacterial cell undergoes a series of well-defined stages: it first replicates its circular DNA, followed by the elongation of the cell, and finally the formation of a septum that divides the cell into two genetically identical daughter cells. This process, clearly illustrated in , transforms one cell into two separate entities, each equipped with a complete copy of the original genetic material. The simplicity and speed of binary fission allow bacteria to thrive in diverse environments and adapt quickly to changing conditions, underscoring their evolutionary success. Understanding this reproductive strategy is crucial, as it not only informs microbial ecology but also has significant implications in areas such as medicine and biotechnology, where control of bacterial populations is often necessary to prevent disease and contamination.
A. Binary Fission: Step-by-Step Process
Binary fission is a fundamental and rapid method of reproduction in bacteria, characterized by a systematic and highly efficient step-by-step process. Initially, a single bacterial cell containing a circular chromosome undergoes DNA replication, starting at a specific origin of replication. As the cell elongates, the replicated chromosomes move toward opposite ends, ensuring that each future daughter cell will contain a complete set of genetic material. Following elongation, a septum, composed of cell membrane and peptidoglycan, forms in the middle of the cell, eventually leading to the physical separation of the two new cells. This bifurcation results in two genetically identical daughter cells, each equipped with the necessary cellular machinery to thrive. The detailed mechanisms involved in binary fission are effectively illustrated in the accompanying image , which visually depicts each stage of this process. Understanding binary fission is crucial when comparing it to viral replication, highlighting the differences in reproductive strategies among microorganisms.
Step | Description | Duration (minutes) | Key Feature |
Step 1 | DNA replication begins, where the bacterial chromosome is duplicated. | 5 | Replication forks form at the origin of replication. |
Step 2 | Cell elongation occurs as built-up materials drive the cell to grow. | 10 | Cell membrane and wall elongate. |
Step 3 | Formation of the septum begins at the middle of the cell. | 5 | New cell wall material is synthesized. |
Step 4 | The septum is completed, dividing the cell into two daughter cells. | 5 | Each daughter cell has identical genetic material. |
Step 5 | Daughter cells separate and can start the cycle anew. | 1 | Cells can now grow and prepare for further division. |
Binary Fission Process Steps
B. Conjugation, Transformation, and Transduction
The processes of conjugation, transformation, and transduction exemplify the mechanisms by which bacteria exchange genetic material, thereby enhancing their adaptability and evolution. Conjugation involves direct contact between bacterial cells, typically facilitated by a structure known as a pilus, allowing for the transfer of plasmids carrying advantageous genes, such as antibiotic resistance. Transformation, on the other hand, occurs when a bacterium incorporates free DNA from its surrounding environment into its own genome, a process that underscores the role of environmental DNA in genetic variation. Furthermore, transduction is mediated by bacteriophages, which can inadvertently transfer DNA between bacterial hosts during viral replication, thus introducing new genetic traits. Collectively, these processes contribute to the genetic diversity in bacterial populations, providing them with the tools to adapt to shifting environments and survive under selective pressures. The image illustrating these processes, , visually summarizes the intricate nature of horizontal gene transfer, reinforcing their significance in bacterial reproduction and evolution.
Mechanism | Description | Example Organisms | Key Features |
Conjugation | Transfer of genetic material between bacteria through direct contact. | Escherichia coli, Bacillus subtilis | Involves a pilus, typically transfers plasmids. |
Transformation | Uptake of free DNA from the environment by a bacterial cell. | Streptococcus pneumoniae, Neisseria gonorrhoeae | Can occur naturally or be induced; important for genetic engineering. |
Transduction | Transfer of DNA from one bacterium to another via bacteriophages. | Pseudomonas aeruginosa, Salmonella enterica | Can transfer large segments of DNA; involves viral infection. |
Mechanisms of Bacterial Genetic Exchange
C. Factors Influencing Bacterial Growth
Bacterial growth is a complex phenomenon influenced by various environmental and physiological factors. Primarily, nutrient availability plays a crucial role, as cells require specific macronutrients such as carbon, nitrogen, and phosphorus for optimal growth and reproduction through binary fission. Additionally, factors such as temperature, pH, and oxygen levels significantly impact bacterial cell division and metabolic function. For instance, most bacteria thrive in a neutral pH and moderate temperatures, which can be visualized in the growth performance metrics from experimental data, such as those exhibited in . Furthermore, the presence of inhibitory substances, including antibiotics or competing microorganisms, can restrict growth rates and affect population dynamics. Understanding these factors is crucial in microbiology, particularly when considering applications such as antibiotic resistance and industrial fermentation processes. Through the integration of these elements, researchers can gain deeper insights into bacterial reproduction and its implications in various biological systems.
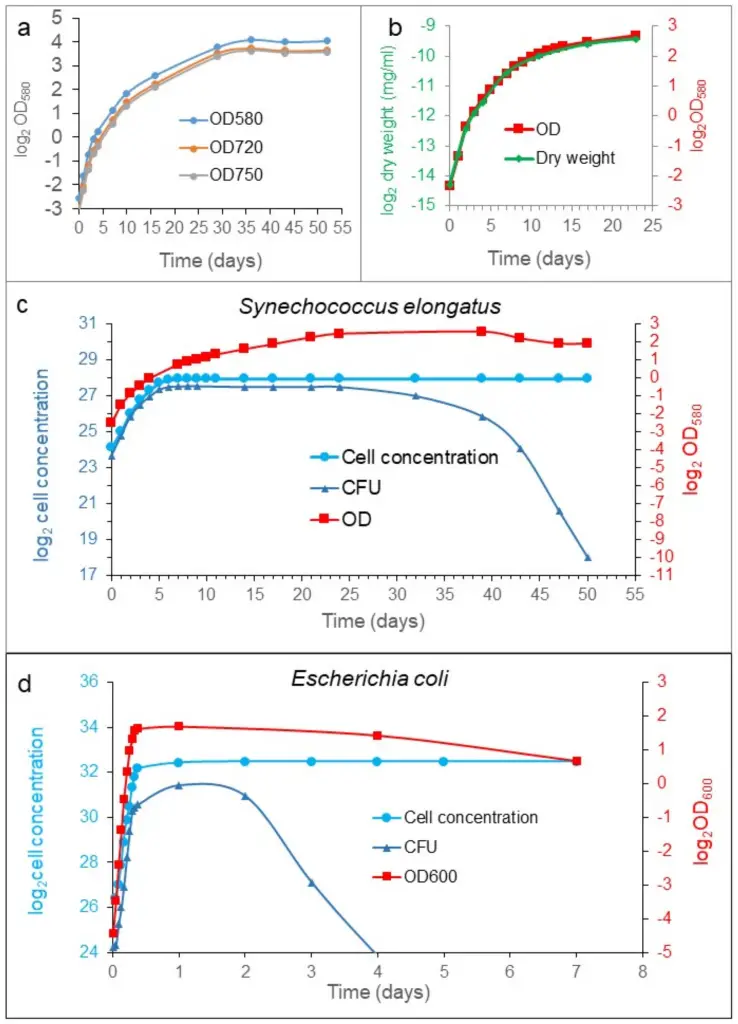
Image : Growth Metrics of Synechococcus elongatus and Escherichia coli Over Time (The image presents four panels (a, b, c, and d) displaying various experimental data related to the growth metrics of Synechococcus elongatus and Escherichia coli over time. Panel (a) illustrates changes in optical density (OD580, OD720, OD750) over 55 days, measuring the culture growth. Panel (b) correlates optical density with dry weight measurements, noting their progression over 24 days. Panel (c) focuses on Synechococcus elongatus, providing a comparative analysis of cell concentration, colony-forming units (CFU), and optical density (OD) over time. Lastly, Panel (d) presents similar metrics for Escherichia coli, tracking changes in cell concentration and OD (OD600) over 7 days. The graphs utilize logarithmic scales to enhance clarity in the representation of growth dynamics.)
Factor | Optimal Range | Impact on Growth |
Temperature (°C) | 30-37 | Inhibitory outside range |
pH Level | 6.5-7.5 | Significant growth at optimum pH |
Oxygen Availability | Aerobic, Anaerobic, Facultative | Depends on oxygen type requirement |
Nutrient Availability | Carbon, Nitrogen, Phosphorus | Growth limited by nutrient scarcity |
Moisture Level | High moisture | Dry conditions inhibit growth |
Salinity | 1-3% salt concentration | High salinity can be inhibitory |
Factors Influencing Bacterial Growth
III. How Viruses Reproduce
Viruses reproduce through a complex process known as viral replication, which fundamentally differs from the binary fission of bacteria. This process begins when a virus attaches to a specific host cell, utilizing receptor proteins to gain entry. Once inside, the viral genome is released and commandeers the host’s cellular machinery to synthesize new viral components, including proteins and nucleic acids. The host cell then assembles these components into new virions, which are subsequently released, often causing cell lysis or sometimes budding off to infect additional cells. This method allows viruses to produce numerous progeny rapidly, contributing to their virulence and ability to spread within a host organism. The differences in replication strategies between bacteria and viruses highlight the unique adaptability and pathogenicity of these microorganisms, a theme underscored by the comparative dynamics illustrated in the image on viral growth patterns and dynamics . Understanding these methods not only expands our comprehension of microbiology but also informs medical strategies to combat viral infections.
A. The Lytic Cycle: Rapid Viral Multiplication
The lytic cycle is a critical mechanism by which viruses reproduce, illustrating a stark contrast to the binary fission process of bacteria. This cycle begins when a virus attaches to a susceptible host cell and injects its genetic material, leading to the hosts cellular machinery being hijacked to produce viral components. Unlike bacteria, which undergo division through a simple process of binary fission, viruses like bacteriophages exhibit a more complex, rapid replication strategy. The biosynthesis phase sees the assembly of viral particles, culminating in cell lysis, where the host cell bursts, releasing newfound virions into the environment to infect adjacent cells. This lytic process is exemplified in the graphical representations of viral growth patterns, especially the differences between bacterial asexual reproduction and the dynamic stages of viral replication that unfold in the lytic cycle . Understanding these mechanisms not only highlights the ecological impact of viral infections but also underscores the evolutionary adaptations that distinguish bacterial and viral life forms.
Virus | Generation Time (minutes) | Infectious Particles Produced per Infection | Environment |
T4 Phage | 20 | 200 | Optimal |
Influenza Virus | 6 | 100 | Cell culture |
HIV | 1.5 | 1000 | Human host |
Bacteriophage λ | 30 | 150 | Bacterial culture |
Viral Replication Rates in Different Environments
B. The Lysogenic Cycle: Dormancy and Activation
The lysogenic cycle represents a sophisticated mechanism of viral replication that contrasts significantly with the binary fission observed in bacteria. During this cycle, a bacteriophage integrates its genetic material into the host bacteriums genome, establishing a dormant state known as the lysogenic state. In this dormant phase, the viral DNA, or prophage, replicates alongside the host’s DNA during cell division without causing immediate harm. This strategic dormancy grants the virus a considerable advantage, ensuring its propagation under favorable conditions while evading the hosts immune response. Activation of the lysogenic cycle can occur due to environmental stressors, prompting the virus to enter the lytic cycle, where it hijacks the hosts machinery to produce new virions. This dual nature of viral existence embodies a complex interaction between virus and host, drawing attention to the adaptive strategies of viruses compared to the direct reproduction via binary fission seen in bacteria. The visual representation of these cycles could be effectively enhanced by referencing , which articulates the differences in growth patterns between bacteria and viruses, shining light on the implications of these reproductive techniques.
Stage | Description | Duration (Hours) | Typical Hosts | Example Viruses |
Lysogenic Phase | Viral DNA integrates into the host genome, remaining dormant. | Indefinite | Bacteria such as Escherichia coli | Lambda phage, Epstein-Barr virus |
Trigger for Activation | Environmental factors such as UV radiation or stress induce the viral DNA to enter the lytic cycle. | Varies based on environmental factors | Bacteria and human cells | Herpes Simplex Virus, Varicella-Zoster Virus |
Lytic Cycle Activation | Viral DNA replicates and new virions are produced, leading to cell lysis. | Generally 20-30 hours | Bacteria and various animal cells | T4 bacteriophage, Influenza virus |
Lysogenic Cycle Data Comparison
C. How Viruses Hijack Host Cells
Viruses exhibit a unique reproductive strategy that fundamentally differs from the binary fission seen in bacteria. Unlike bacteria, which are free-living organisms that can replicate independently, viruses rely on hijacking host cells to reproduce. Upon infection, a virus attaches to a specific receptor on the host cells surface, facilitating the entry of its genetic material into the host. Once inside, the viral genome commandeers the hosts cellular machinery, redirecting it to synthesize viral components instead of normal cellular functions. This manipulation leads to the assembly of new viral particles, which eventually burst from the host cell, often destroying it in the process. This intricate process is crucial for understanding the pathogenicity of viruses, as it highlights their dependence on host organisms for replication. The comparative analysis of these reproductive mechanisms emphasizes the distinctive and adaptive strategies employed by viruses, a concept effectively illustrated in the contrasting growth patterns shown in the graph labeled .
Virus Type | Host Cell Type | Mechanism | Replication Speed | Impact on Host |
HIV | CD4+ T cells | Binding to CD4 receptors and co-receptors, entering via fusion | Rapid (within 24-48 hours) | Leads to immune deficiency and increased susceptibility to infections |
Influenza | Epithelial cells of the respiratory tract | Attach via hemagglutinin (HA) to sialic acid receptors | Moderate (hours to days) | Causes respiratory illness, can lead to severe complications |
Hepatitis B | Liver cells (hepatocytes) | Binding through a specific receptor and translocating genome into nucleus | Slow (weeks to months) | Can lead to chronic liver disease and liver cancer |
COVID-19 (SARS-CoV-2) | Human respiratory epithelial cells | Binding via ACE2 receptors, entry via endocytosis | Rapid (24-72 hours) | Severe respiratory illness, long-term health effects |
Comparison of Virus Hijacking Mechanisms
IV. Impact of Reproduction on Disease Spread
The reproductive mechanisms of bacteria and viruses play a pivotal role in the spread of diseases, influencing epidemiological patterns and public health responses. Bacteria reproduce through binary fission, a process wherein a single cell divides into two identical offspring, effectively doubling the population in optimal conditions. This method allows for rapid increases in bacterial numbers, leading to extensive outbreaks if left unchecked. In contrast, viruses rely on hijacking host cellular machinery to replicate, resulting in the production of numerous viral particles that can disseminate throughout the host and subsequently infect new cells. The speed and efficiency of these reproductive mechanisms directly correlate with the ease of transmission, as higher reproductive rates generally lead to quicker outbreaks. Understanding how these reproductive strategies contribute to the spread of infections is crucial for developing effective prevention and control measures, as visualized in image reference . As a result, comprehending the nuances of bacterial and viral reproduction is integral to strategizing interventions against infectious diseases.
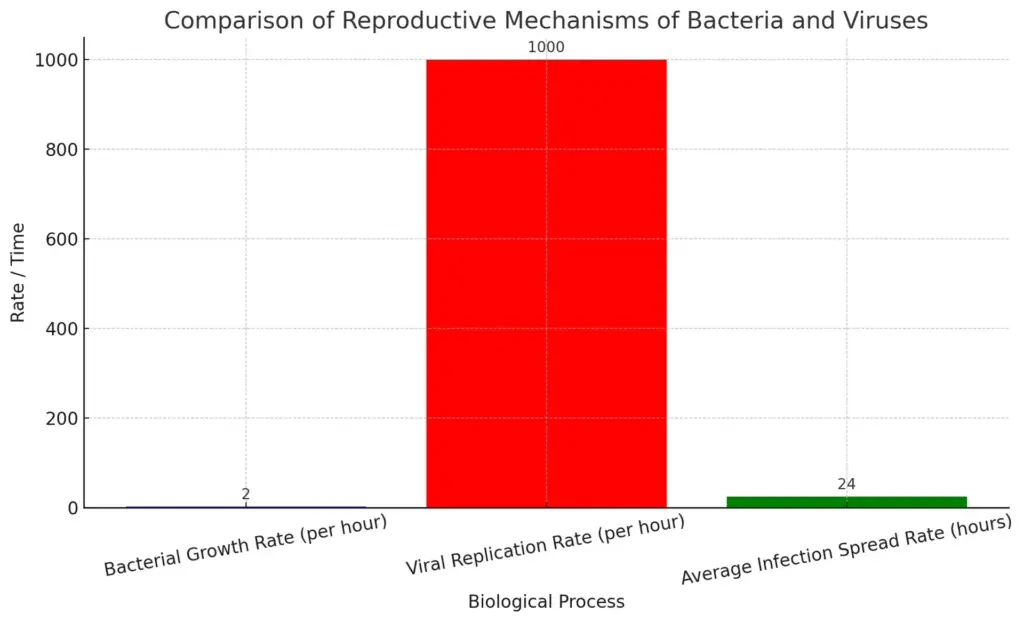
This bar chart compares the reproductive mechanisms of bacteria and viruses, illustrating the significant differences in their growth rates. The chart shows that bacteria can grow at a rate of 2 per hour through binary fission, while viruses can replicate at a much higher rate of up to 1000 per hour via host cell hijacking. Additionally, the average infection spread rate is noted as 24 hours. This visualization highlights the exponential nature of viral replication and the implications for disease transmission.
A. How Bacterial Growth Leads to Infections
The rapid growth of bacterial populations through processes such as binary fission is a significant factor in the emergence of infections. As bacteria proliferate, they can overwhelm host defenses, leading to a cascade of pathogenic events. When bacteria find a suitable environment, nutrients, and minimal competition, they can multiply exponentially, sometimes yielding trillions within a short period. This prolific increase in bacterial cells can result in the colonization of tissues, inflammation, and ultimately the disruption of normal cellular functions. The dynamics of bacterial growth are further complicated by gene transfer mechanisms, enabling the acquisition of virulence factors that enhance their pathogenicity. For a visual representation of how this binary fission occurs, effectively illustrates the stages of bacterial reproduction. Understanding these processes underscores the importance of timely interventions to control bacterial proliferation and prevent infections, illuminating the intricate relationship between growth dynamics and disease manifestation.
Year | Global Cases (Millions) | Global Deaths (Thousands) | Common Infections |
2020 | 4 | 5 | Staphylococcal infections, Streptococcal infections |
2021 | 4.5 | 6.2 | Pneumonia, Tuberculosis |
2022 | 5.2 | 7 | E. coli infections, Salmonella |
2023 | 5.8 | 8.1 | Clostridium difficile infections, Urinary tract infections |
Bacterial Infections Statistics
B. How Viral Replication Affects Host Cells
Viral replication profoundly impacts host cells, altering their normal physiological functions and often leading to cell death. When a virus infects a host cell, it injects its genetic material and commandeers the cellular machinery to produce viral components. This hijacking disrupts the hosts metabolic processes and can lead to the shutdown of essential cellular functions. Furthermore, the accumulation of viral particles within the host can induce cellular stress, triggering immune responses that aim to eliminate the infected cells. However, this immune reaction not only targets the virus but can also damage surrounding healthy tissues. In many cases, the virus induces apoptosis, a programmed cell death, to release newly formed viral particles, perpetuating the cycle of infection. Visual aids, such as an image illustrating the stages of viral replication, highlight this process effectively, providing a clear representation of how viruses manipulate host cellular mechanisms to facilitate their own reproduction.
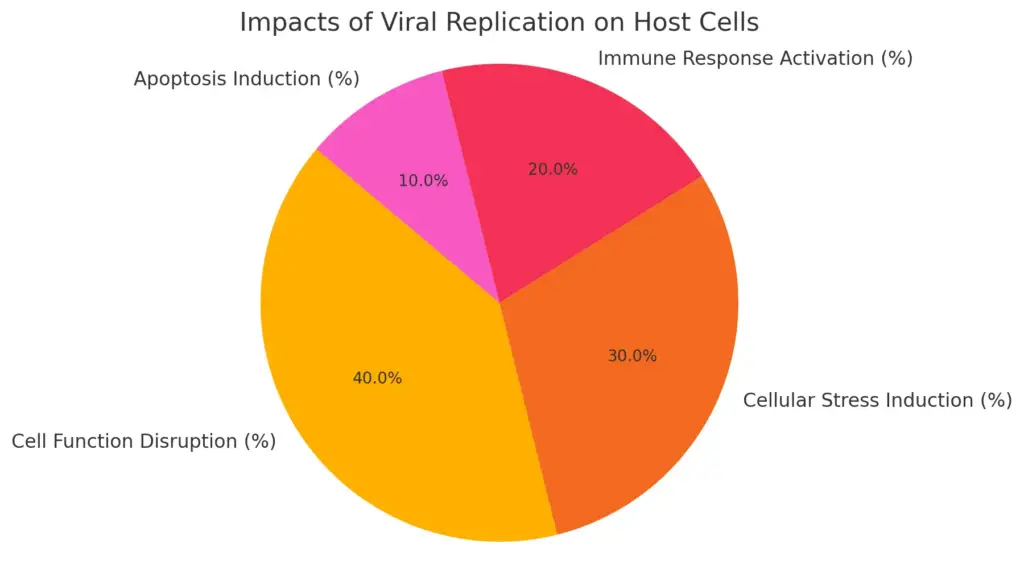
This pie chart illustrates the proportions of various impacts of viral replication on host cells. It shows that cell function disruption is the most significant factor, followed by cellular stress induction and immune response activation, highlighting the multifaceted effects viral replication has on cellular health.
V. Conclusion
In conclusion, the contrasting reproductive strategies of bacteria and viruses highlight fundamental differences in their biology and evolutionary adaptiveness. Bacteria reproduce through a well-defined process known as binary fission, enabling rapid population growth and survival in various environments, as illustrated in . This method allows for genetic stability, yet also facilitates horizontal gene transfer, enhancing adaptability amongst bacterial populations, evidenced by processes like transformation and transduction (). Conversely, viruses engage in a complex replication cycle that involuntarily harnesses host cellular machinery to propagate, resulting in a rapid accumulation of virions, as depicted in the viral growth patterns outlined in . This dichotomy illustrates not only the diverse mechanisms of reproduction but also the implications for the understanding of pathogenicity and treatment strategies. Ultimately, recognizing these differences is crucial for advancing microbiological research and developing effective interventions against bacterial and viral infections.
A. Key Takeaways
In concluding the exploration of reproductive mechanisms among bacteria and viruses, several key takeaways emerge that highlight fundamental biological distinctions. Bacteria utilize binary fission—a straightforward process involving DNA replication followed by cellular division into two progeny cells, each genetically identical to the original, as illustrated in . This method allows for rapid population increase under favorable conditions. In contrast, viral replication is contingent upon the host cells machinery, marked by distinct stages including attachment, penetration, synthesis, assembly, and release, which emphasizes their dependence on living cells for propagation. The associated growth dynamics, represented in , further elucidate how these processes differ in their growth patterns and timelines, with bacterial populations growing exponentially while viral replication exhibits more complex patterns. Understanding these reproductive strategies provides insights not only into microbial ecology but also into the implications for disease management and antibiotic resistance, emphasizing the need for targeted approaches in treating infections caused by these diverse entities.
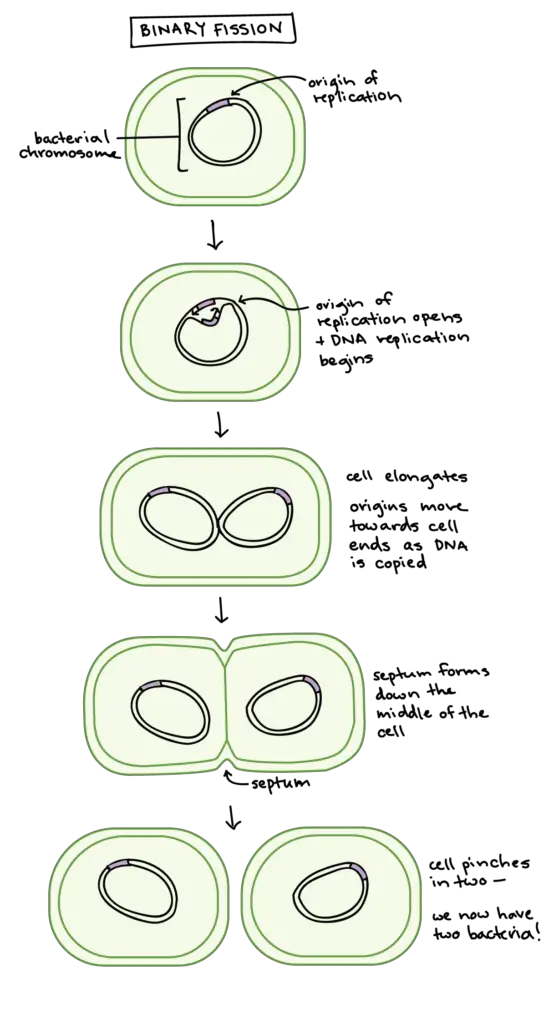
The image illustrates the process of binary fission in bacteria, depicting five key stages. It starts with a single bacterial cell containing a circular chromosome. The first diagram shows the origin of replication, where DNA replication initiates. The following steps illustrate the elongation of the cell as the DNA is duplicated and the origins move towards the ends of the cell. Next, the formation of a septum is shown, which divides the cell into two. Finally, the process culminates in the pinching off of the septum, resulting in two separate bacterial cells, each with a copy of the original chromosome. This sequence is essential for understanding prokaryotic cell division and its implications in microbiology and cellular biology.
B. Future Research on Microbial Reproduction
Future research on microbial reproduction holds immense potential for advancing our understanding of both bacterial and viral life cycles, particularly in the context of their reproductive strategies. By exploring the nuanced mechanisms behind binary fission and viral replication, researchers may uncover novel genetic pathways and regulatory networks that govern these processes. For example, the study of horizontal gene transfer mechanisms, as depicted in , could elucidate how bacteria adapt to environmental stresses and share advantageous traits. Furthermore, examining the intricate growth patterns of these microorganisms—illustrated through comparative graphs in —could provide insights into population dynamics and evolutionary strategies. Investigating the interactions between bacterial and viral populations, including co-infections and competition, promises to enhance our comprehension of microbial ecology and its implications for health and disease. Ultimately, such research endeavors will not only deepen our biological knowledge but also inform practical applications in medicine and biotechnology, particularly in combating resistant pathogens and viral epidemics.
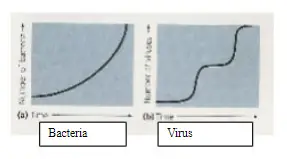
Image : Comparison of Growth Patterns in Bacteria and Viruses (The image presents two graphs comparing the growth patterns of bacteria and viruses over time. The graph on the left, labeled ‘Bacteria’, displays a continuous increasing trend that suggests exponential growth, commonly seen in bacterial populations under optimal growth conditions. Conversely, the graph on the right, labeled ‘Virus’, illustrates a more complex growth curve characterized by a lag phase followed by a rapid increase and eventual plateau, indicative of viral replication processes. This visual representation provides insights into the fundamental differences in the reproductive strategies and growth dynamics of prokaryotic and viral entities.)
REFERENCES
- Board on Global Health. ‘The Social Biology of Microbial Communities.’ Workshop Summary, Institute of Medicine, National Academies Press, 1/10/2013
- Jaime Wood. ‘The Word on College Reading and Writing.’ Carol Burnell, Open Oregon Educational Resources, 1/1/2020
- Leonard W. Ortmann. ‘Public Health Ethics: Cases Spanning the Globe.’ Drue H. Barrett, Springer International Publishing, 4/20/2016
- Center for Professional Responsibility (American Bar Association). ‘Model Rules of Professional Conduct.’ American Bar Association. House of Delegates, American Bar Association, 1/1/2007
- Gabriella Campadelli-Fiume. ‘Human Herpesviruses.’ Biology, Therapy, and Immunoprophylaxis, Ann Arvin, Cambridge University Press, 8/16/2007
- John Eggebrecht. ‘Biology for AP ® Courses.’ Julianne Zedalis, OpenStax, 10/16/2017
- Jennifer Louten. ‘Essential Human Virology.’ Academic Press, 5/28/2022
- Matthias Gotte. ‘Viral Genome Replication.’ Craig E. Cameron, Springer Science & Business Media, 5/28/2009
- Steven E. Schutzer. ‘Microbial Forensics.’ Bruce Budowle, Academic Press, 10/27/2010
- Alistair McCleery. ‘An Introduction to Book History.’ David Finkelstein, Routledge, 3/13/2006