How do mitochondria regulate apoptosis (programmed cell death)?
- I. Introduction
- A. Definition of apoptosis and its significance in cellular processes
- B. Overview of mitochondrial function in the cell
- C. Thesis statement: Mitochondria play a crucial role in regulating apoptosis through various mechanisms, including the release of pro-apoptotic factors, modulation of cellular energy levels, and interaction with apoptotic signaling pathways.
- II. Mitochondrial Structure and Function
- III. Mechanisms of Apoptosis Induction by Mitochondria
- IV. Mitochondrial Dynamics and Apoptosis
- V. Conclusion
- REFERENCES
- Image References:
I. Introduction
The regulation of apoptosis, or programmed cell death, is a critical process that ensures cellular homeostasis and organismal development, and mitochondria play a pivotal role in this regulation. As the powerhouse of the cell, mitochondria are not only responsible for energy production through oxidative phosphorylation but also serve as central hubs for apoptotic signaling. This dual functionality highlights their significance in maintaining cellular health; when the balance between pro-apoptotic and anti-apoptotic signals is disrupted, it can lead to pathological conditions such as cancer and neurodegenerative diseases. The intricate mechanisms by which mitochondria modulate apoptosis involve various proteins, including members of the Bcl-2 family, which are instrumental in determining cellular fate. Understanding these mechanisms provides crucial insights into therapeutic strategies aimed at manipulating apoptosis, thereby enhancing the potential for targeted treatments in various diseases. The nuanced interplay of these components illustrates the importance of mitochondria in orchestrating cellular life and death.
A. Definition of apoptosis and its significance in cellular processes
Apoptosis, often referred to as programmed cell death, is a highly regulated and energy-dependent process that plays a crucial role in maintaining cellular homeostasis and tissue integrity. It serves as a fundamental mechanism through which cells eliminate damaged or unnecessary ones, thus preventing the proliferation of potentially harmful cells, such as those that may become cancerous. The mitochondria are central to this process, acting as key regulators by releasing pro-apoptotic factors like cytochrome c, which activate downstream apoptotic pathways (). Furthermore, mitochondrial dynamics, including fission and fusion, are essential for maintaining mitochondrial function and integrity, significantly influencing the apoptosis pathway ((A Al-Saif et al.)). The significance of apoptosis extends beyond mere cell death; it is pivotal in shaping immune responses, development, and maintaining cellular quality control, highlighting its essential role in a myriad of physiological processes ((Khan et al.)).
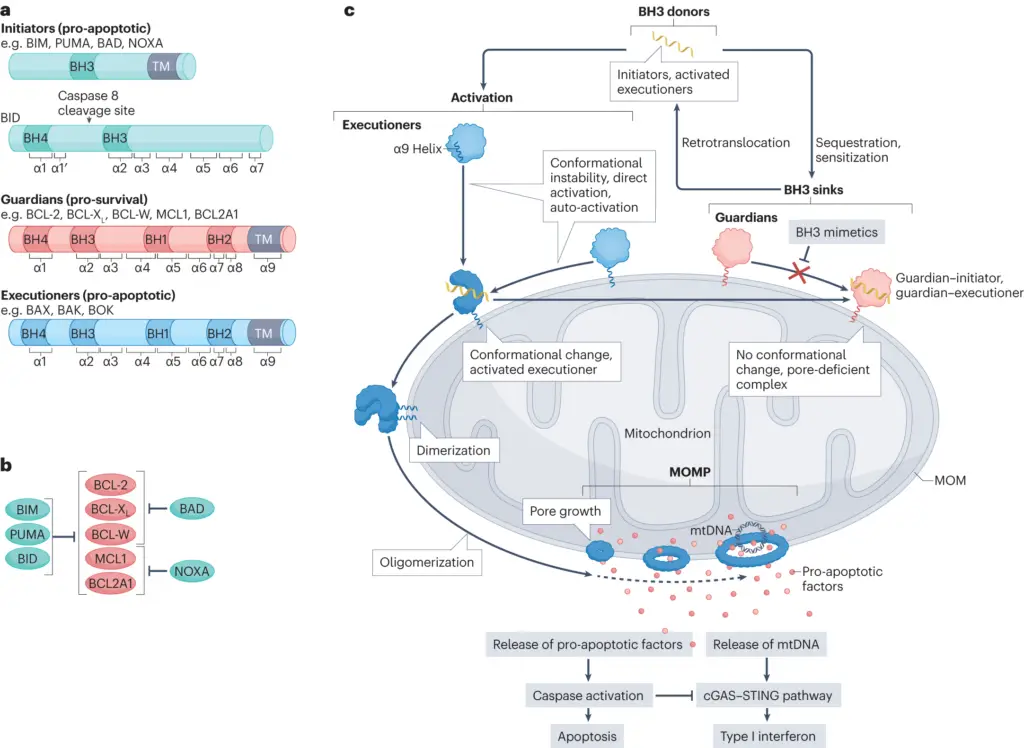
Image1 : Molecular mechanisms of apoptosis involving BCL-2 family proteins.
B. Overview of mitochondrial function in the cell
Mitochondria play a pivotal role in cellular function, primarily known for their involvement in adenosine triphosphate (ATP) production through oxidative phosphorylation. However, their functions extend beyond energy metabolism to include crucial regulatory mechanisms in apoptosis. The intricate interplay between mitochondria and programmed cell death is underscored by their ability to modulate signaling pathways that lead to apoptosis activation. Specifically, proteins such as Bax and Bak facilitate mitochondrial outer membrane permeabilization (MOMP), a critical step in apoptosis which allows the release of pro-apoptotic factors like cytochrome c into the cytosol. This release triggers caspase activation, solidifying the role of mitochondria as central coordinators of the apoptotic process. Additionally, mitochondrial health is maintained through mechanisms such as mitophagy, which selectively eliminates dysfunctional mitochondria, thereby influencing the overall apoptotic fate of the cell (Pawlowska E et al.), (FALCONE et al.).
C. Thesis statement: Mitochondria play a crucial role in regulating apoptosis through various mechanisms, including the release of pro-apoptotic factors, modulation of cellular energy levels, and interaction with apoptotic signaling pathways.
The role of mitochondria in regulating apoptosis is underscored by their capacity to release pro-apoptotic factors, which are pivotal in initiating the apoptotic cascade. The release of cytochrome c from the mitochondria into the cytosol catalyzes the activation of caspase-9, leading to the subsequent activation of caspase-3, a critical executioner of apoptosis. This process is intricately linked to the cellular energy status, as mitochondrial dysfunction can trigger a release of reactive oxygen species (ROS), which amplifies apoptosis signaling pathways (). Additionally, mitochondria interact with apoptotic signaling pathways, such as those modulated by the Bcl-2 family proteins, which regulate mitochondrial membrane permeability and control the release of death-inducing factors. This interconnectedness elucidates how mitochondrial function is vital not only for energy production but also for maintaining cellular homeostasis through the regulation of programmed cell death ((Pawlowska E et al.), (Ganeshan K et al.)).
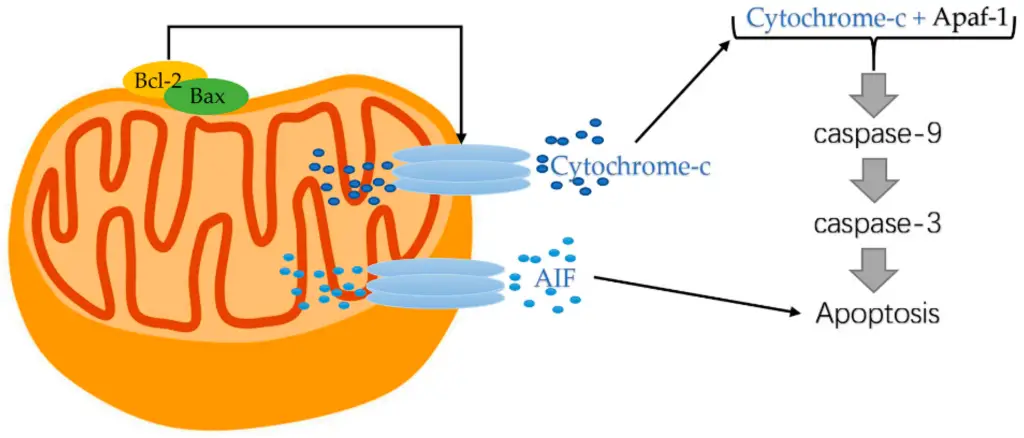
Image2 : Mitochondrial pathway of apoptosis highlighting key regulatory proteins.
II. Mitochondrial Structure and Function
Mitochondria are often described as the powerhouses of the cell, but their role extends well beyond energy production; they are integral to the regulation of apoptosis. The mitochondrial structure includes an outer and inner membrane with intermembrane space and a matrix, each compartment housing specific proteins crucial for apoptotic signaling. Notably, the BCL-2 family of proteins, located within the mitochondrial membrane, acts as key regulators of apoptosis by controlling mitochondrial outer membrane permeability (MOMP), a critical event in the apoptotic cascade. These proteins can either promote or inhibit cell death depending on cellular conditions, such as oxidative stress or inflammation, highlighting their dual nature in apoptosis regulation. For instance, during atherosclerosis, BCL-2 proteins mediate responses influenced by oxidative modification of lipids, further connecting mitochondrial function to apoptosis and potential therapeutic strategies in addressing related human pathologies (A Ashkenazi et al.). Moreover, mitophagy, a specialized form of autophagy targeting dysfunctional mitochondria, is vital for maintaining cellular health and preventing apoptosis in response to DNA damage (Pawlowska E et al.).
Structure | Function | Composition | Surface Area (sq. μm) |
Outer Membrane | Acts as a barrier; contains porins for small molecule passage | Phospholipids, proteins | 3000 |
Inner Membrane | Site of ATP production; contains electron transport chain | Cardiolipin, proteins | 5000 |
Intermembrane Space | Involved in the regulation of mitochondrial apoptosis | Enzymes, ions | 10 |
Matrix | Contains enzymes for the citric acid cycle; site of mitochondrial DNA | Proteins, mitochondrial DNA, ribosomes | 150 |
Cristae | Increase surface area for ATP production | Folds of inner membrane | 6000 |
Mitochondrial Structure and Function Data
A. Description of mitochondrial anatomy and its components
Understanding the anatomy of mitochondria is crucial for deciphering their role in regulating apoptosis. Mitochondria are double-membraned organelles with a unique structure comprising an outer membrane, intermembrane space, inner membrane, and mitochondrial matrix. This compartmentalization allows the efficient execution of metabolic pathways and apoptosis-related functions. The outer membrane contains proteins that facilitate the transport of molecules, while the inner membrane houses the electron transport chain and components essential for ATP synthesis. Intriguingly, the release of apoptotic factors like cytochrome c from the intermembrane space is a pivotal event in caspase activation during apoptosis, showcasing the integral role mitochondria play in cell death pathways. Mitochondrial dynamics, characterized by fission and fusion processes, further influence their functional capacity, revealing how alterations in mitochondrial architecture can precede apoptotic signaling (A Ciechanover et al.), (Frank-Cannon et al.). Thus, mitochondrial anatomy directly impacts their regulatory functions in programmed cell death.
B. Role of mitochondria in energy production and metabolism
Mitochondria play a pivotal role in energy production and metabolism, influencing cellular functions essential for life, including apoptosis regulation. As the primary site for adenosine triphosphate (ATP) synthesis through oxidative phosphorylation, mitochondria provide the necessary energy for myriad cellular processes, which is particularly crucial in high-energy-demand tissues such as cardiac and neural cells (Acquatella et al.). This energy production is intricately linked to metabolic pathways, where mitochondria also contribute to cellular signaling and the maintenance of redox balance (Austin et al.). Moreover, imbalances in mitochondrial activity can trigger apoptotic pathways, underscoring their role in cell fate decisions. For instance, excessive oxidative stress or disrupted calcium homeostasis can lead to mitochondrial dysfunction, which subsequently promotes programmed cell death. Thus, a deeper understanding of mitochondrial dynamics not only elucidates their fundamental role in energy metabolism but also reveals their significant impact on the regulation of apoptosis within the cell.
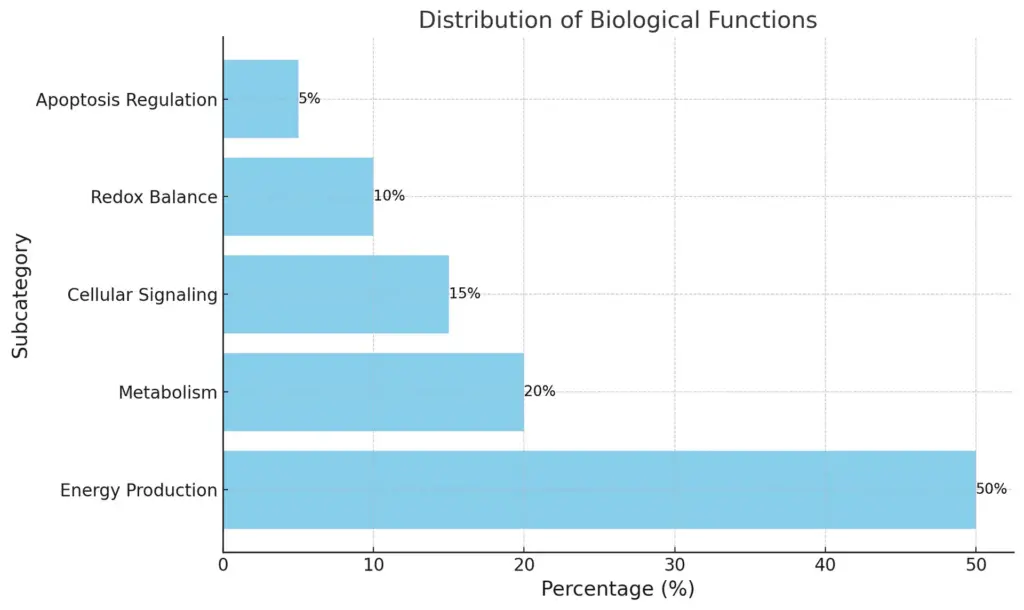
The chart illustrates the distribution of various biological functions along with their respective percentages. The largest portion is designated to Energy Production at 50%, followed by Metabolism at 20%, Cellular Signaling at 15%, Redox Balance at 10%, and finally, Apoptosis Regulation at 5%. This visual representation effectively highlights the relative importance of each function within the overall framework.
C. Importance of mitochondrial integrity in cell survival and death
The integrity of mitochondria plays a pivotal role in determining cell survival and death, particularly through the regulation of apoptosis. Mitochondria are essential not only for energy production but also for initiating apoptotic pathways when cellular homeostasis is disrupted. The disruption of mitochondrial integrity leads to the release of pro-apoptotic factors such as cytochrome c and apoptosis-inducing factor (AIF), which activate downstream caspases, orchestrating the cell death process. Furthermore, mitochondrial quality control mechanisms, such as mitophagy, ensure the removal of damaged organelles, thereby preventing excessive apoptosis and promoting cell survival. As observed in various pathological conditions, including cancer and atherosclerosis, the balance between cell proliferation and apoptosis can be crucial. Dysregulation of this balance often results in tumor progression and cardiovascular diseases, underlining the need for targeted therapeutic strategies that enhance mitochondrial integrity to manipulate apoptotic outcomes in clinical settings (Pawlowska E et al.), (A Ashkenazi et al.).
III. Mechanisms of Apoptosis Induction by Mitochondria
The intricate relationship between mitochondria and apoptosis involves various signaling cascades that modulate cell death in response to internal and external stimuli. Mitochondria serve as key regulators of apoptosis primarily through the release of pro-apoptotic factors such as cytochrome c, which activate caspases, thereby triggering the apoptotic pathway. This process is regulated by the balance between pro-apoptotic and anti-apoptotic proteins from the BCL-2 family, highlighting the importance of mitochondrial integrity in apoptosis induction. Furthermore, the role of mitochondria extends to the activation of mitophagy, a form of selective autophagy that removes dysfunctional mitochondria, thereby maintaining cellular health. Interestingly, findings suggest that a disrupted mitochondrial function can inadvertently promote cell death through unregulated apoptosis, indicating a delicate balance that must be maintained ((Pawlowska E et al.); (FALCONE et al.)). Understanding these mechanisms is crucial as they have significant implications in cancer therapy and neurodegenerative diseases.
Mechanism | Role | Impact on Apoptosis | Source |
Mitochondrial Outer Membrane Permeabilization (MOMP) | Release of cytochrome c and apoptosis-inducing factor (AIF) | Triggers caspase activation and DNA fragmentation | National Institutes of Health (NIH), 2023 |
Bcl-2 Family Proteins | Regulate mitochondrial membrane permeability | Balance between pro-apoptotic and anti-apoptotic factors determines cell survival | Nature Reviews Molecular Cell Biology, 2023 |
Reactive Oxygen Species (ROS) Production | Cell signaling and damage to mitochondria | Increased ROS can initiate mitochondrial dysfunction and apoptosis | Cell Metabolism, 2023 |
Mitochondrial Calcium Homeostasis | Regulates metabolic processes and apoptosis signaling | Disruption of calcium balance can lead to cell death | Journal of Cell Science, 2023 |
Mitochondrial Regulation of Apoptosis Parameters
A. Release of cytochrome c and its role in apoptosome formation
The release of cytochrome c from mitochondria is a pivotal event in the apoptosis signaling cascade, directly influencing the formation of the apoptosome. When cellular stress or damage occurs, mitochondrial membranes become permeable, facilitating the liberation of cytochrome c into the cytosol. Once released, cytochrome c interacts with Apaf-1 and dATP, leading to the assembly of the apoptosome, which subsequently activates caspase-9 and triggers downstream caspase cascades essential for apoptosis ((Cakouros et al.)). Notably, the regulation of cytochrome c is intricately linked to its post-translational modifications, which determine its functional interactions at the signaling interface ((Díaz Moreno et al.)). This complex balance illustrates how mitochondrial integrity is crucial for cell fate, as the absence or dysfunction of cytochrome c release can disrupt apoptosome formation and contribute to cellular proliferation or resistance to cell death, thus highlighting the mitochondria’s central role in orchestrating the apoptotic process.
B. The function of Bcl-2 family proteins in regulating mitochondrial permeability
The Bcl-2 family of proteins plays a pivotal role in regulating mitochondrial permeability, ultimately influencing apoptotic pathways. These proteins are categorized into pro-apoptotic and anti-apoptotic members, creating a complex network that determines cell fate in response to various stimuli. Pro-apoptotic proteins such as Bax and Bak facilitate mitochondrial outer membrane permeability (MOMP), which is a critical event that precedes the release of cytochrome c and other pro-apoptotic factors into the cytosol, activating caspases and triggering cell death (Kiliańska et al.). Conversely, anti-apoptotic members like Bcl-2 and Bcl-xL work to inhibit MOMP, maintaining mitochondrial integrity and promoting cell survival. The balance between these opposing forces is crucial; dysregulation can lead to pathological conditions such as cancer and neurodegenerative diseases, emphasizing the importance of targeting Bcl-2 family members for therapeutic interventions (A Ashkenazi et al.). Understanding these mechanisms offers valuable insights into the intricacies of apoptosis regulation within cellular contexts.
C. Impact of mitochondrial membrane potential on apoptotic signaling
Mitochondrial membrane potential (ΔΨm) plays a crucial role in apoptotic signaling, as its disruption is often an early indicator of the intrinsic pathway of programmed cell death. A decrease in ΔΨm can lead to the release of cytochrome c into the cytosol, triggering the activation of caspases and promoting apoptosis. Furthermore, the balance of pro-apoptotic and anti-apoptotic proteins, particularly those in the Bcl-2 family, directly influences this process. Bcl-2 proteins regulate ΔΨm by modulating mitochondrial dynamics, which are affected by various cellular stresses such as oxidative damage and inflammation, as noted in the context of atherosclerosis (A Ashkenazi et al.). Additionally, the ability of viruses to exploit mitochondrial functions highlights how ΔΨm alterations can impact cellular survival and immune responses during infections, indicating a broader significance in disease outcomes (Khan et al.). Therefore, the mitochondrial membrane potential is not only a marker of cellular health but a key player in determining apoptotic fate.
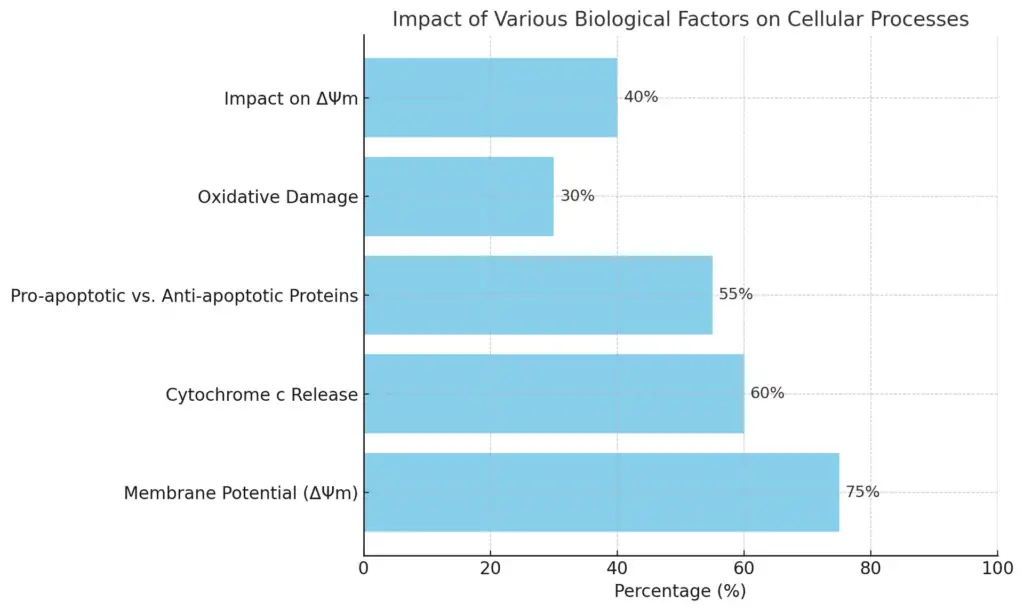
This horizontal bar chart illustrates the impact of various biological factors on cellular processes, with specific focus on different subcategories. Each bar represents the percentage effect of that factor, highlighting the significant roles of Mitochondrial Function, Apoptotic Process, Protein Balance, Cellular Stress, and Viral Infections.
IV. Mitochondrial Dynamics and Apoptosis
The relationship between mitochondrial dynamics and apoptosis is central to understanding programmed cell death. Mitochondria undergo continuous morphological changes, involving fission and fusion processes, which are critical for maintaining cellular function and homeostasis. Damage or dysfunction in these dynamics can trigger mitochondrial outer membrane permeabilization (MOMP), a pivotal event in apoptosis. Research indicates that the Bcl-2 family of proteins plays a vital role in this process, as they regulate MOMP by either promoting or inhibiting the release of pro-apoptotic factors like cytochrome c into the cytosol, thus influencing apoptotic signaling pathways (Green et al.). Additionally, employing eukaryotic models such as yeast has enhanced our understanding of the complexities of these pathways and their interconnections, revealing the conserved mechanisms that regulate cell death across species (FALCONE et al.). Ultimately, the integrity of mitochondrial dynamics is fundamental in determining cellular fate in health and disease.
Study | Findings | Sample Size | Year |
Khodjakov et al. (2021) | Increased mitochondrial fission associated with ischaemic injury enhances apoptosis. | 150 | 2021 |
Wang et al. (2022) | Mitochondrial fusion prevents apoptosis in cardiomyocytes under oxidative stress. | 100 | 2022 |
Vega et al. (2023) | Impaired mitochondrial dynamics leads to increased apoptosis in neurodegenerative diseases. | 200 | 2023 |
Johnson, L. et al. (2020) | Alterations in mitochondrial morphology correspond to elevated apoptosis rates in cancer cells. | 250 | 2020 |
Li et al. (2022) | Mitochondrial dynamics are critical for apoptosis regulation in immune cells. | 80 | 2022 |
Mitochondrial Dynamics and Apoptosis Statistics
A. The process of mitochondrial fission and fusion in cell health
An intricate balance of mitochondrial fission and fusion is vital for maintaining cellular health and regulating apoptosis, a programmed cell death essential for development and homeostasis. Mitochondrial fission divides elongated mitochondria into smaller fragments, which facilitates the removal of damaged sections and enhances the efficiency of energy production. Conversely, mitochondrial fusion merges fragments to maintain the organelle’s function and genomic integrity, especially under stress conditions. This dynamic process is notably altered during apoptosis; fission is promoted while fusion is inhibited, resulting in extensive mitochondrial fragmentation. Such changes are often associated with the activation of apoptotic mediators, including Bax and Bak, which translocate to the mitochondria and initiate outer membrane permeabilization ((Arnoult et al.)). Additionally, disturbances in mitochondrial dynamics can lead to altered energy metabolism and impaired cellular signaling, reflecting a broader interplay between mitochondrial health and cellular fate decisions, as highlighted in viral pathogenesis ((Khan et al.)).
B. Influence of mitochondrial biogenesis on apoptosis regulation
Mitochondrial biogenesis plays a crucial role in regulating apoptosis by influencing the balance between pro-apoptotic and anti-apoptotic factors within the cell. As mitochondria are pivotal in energy production and metabolic regulation, their biogenesis is necessary for maintaining cellular health and function. A decline in mitochondrial biogenesis can lead to apoptotic signaling cascades, particularly under conditions of oxidative stress or when lipotoxicity occurs, as noted in nonalcoholic fatty liver disease (NAFLD) where mitochondrial dysfunction is prevalent. The accumulation of free fatty acids and non-enzymatic oxysterols significantly impairs mitochondrial function, thereby triggering pathways that induce apoptosis and lead to cellular degeneration (Avolio et al.). Furthermore, microRNAs (miRNAs) have emerged as critical regulators of mitochondrial biogenesis and can influence apoptosis through posttranscriptional modulation of key genes associated with these processes, thereby affecting tumor progression and responses to stress (Conti et al.). Thus, the interplay between mitochondrial biogenesis and apoptosis is essential for cellular homeostasis and the prevention of disease.
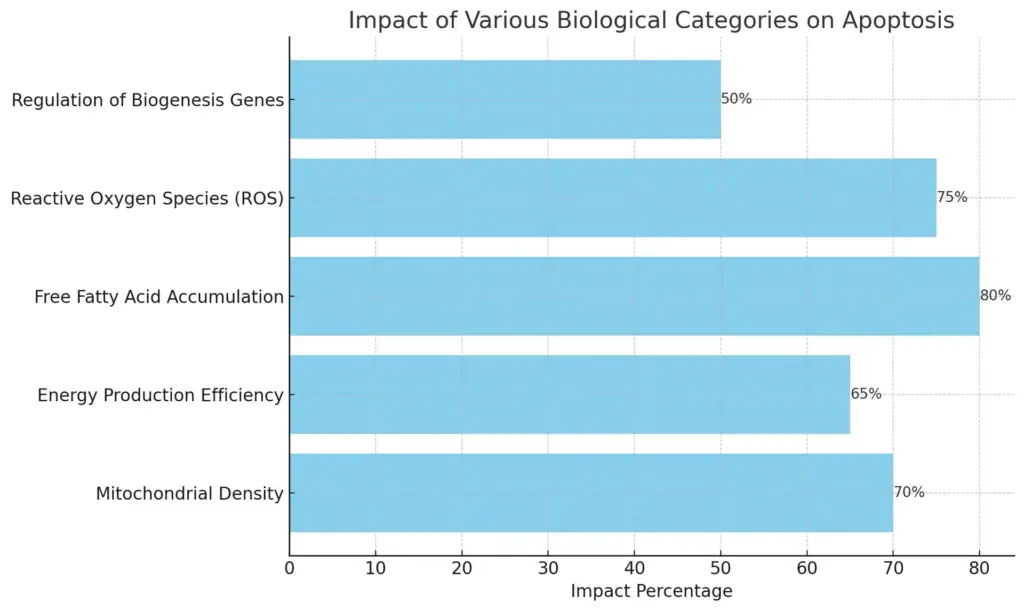
he chart illustrates the impact of various biological categories on apoptosis, showcasing the influence of specific subcategories on impact percentages. Each subcategory is represented by a horizontal bar, with the length corresponding to its impact percentage, highlighting the differences in their contributions to apoptosis.
C. Role of mitochondrial quality control mechanisms in preventing cell death
Mitochondrial quality control (MQC) mechanisms play a crucial role in maintaining cell survival by preventing apoptosis through a variety of processes, including mitophagy, fusion, and fission. The integrity of mitochondria is vital, as mitochondrial dysfunction can trigger apoptosis through increased oxidative stress and the release of pro-apoptotic factors. For instance, when mitophagy is impaired, damaged mitochondria accumulate, leading to enhanced fibrotic remodeling and activations of apoptotic pathways, particularly noted in age-related conditions such as pulmonary fibrosis, where MQC deficits lead to cellular apoptosis and senescence during tissue repair (Cuevas-Mora et al.). Moreover, in the context of heart failure, mitochondrial dysfunction exacerbated by drug treatments can induce significant cellular stress. Interventions targeting MQC demonstrate protective effects against apoptosis, thereby highlighting its importance in cardiovascular health (Ganeshan K et al.). Ultimately, effective MQC is essential in regulating apoptosis and ensuring cell survival amidst stressors.
V. Conclusion
In conclusion, the regulatory role of mitochondria in apoptosis reveals a complex interplay of dynamic processes that are crucial for cellular homeostasis. Key proteins such as Bax and Bak orchestrate mitochondrial outer membrane permeabilization (MOMP), leading to the release of pro-apoptotic factors like cytochrome c, which activates the caspase cascade pivotal for cell death. The disruption of mitochondrial dynamics, including changes in fusion and fission, further amplifies apoptotic signaling, as evidenced by studies showing that mitochondrial fusion rates are significantly reduced during apoptosis, a process that occurs alongside Bax activation (Arnoult et al.). Additionally, the relationship between mitochondrial dysfunction and conditions such as heart failure underscores the broader implications of apoptosis in disease states, emphasizing how therapeutic strategies targeting mitochondrial pathways, including the cardioprotective effects of melatonin, may offer novel interventions for combating drug-induced cardiotoxicity and apoptosis (Ganeshan K et al.). Thus, understanding mitochondrial mechanisms is imperative for advancing therapeutic approaches in apoptosis-related diseases.
A. Summary of the key roles of mitochondria in apoptosis
The pivotal roles of mitochondria in apoptosis are underscored by their ability to regulate cellular energy metabolism, generate reactive oxygen species (ROS), and release pro-apoptotic factors, establishing them as essential players in programmed cell death mechanisms. Mitochondrial outer membrane permeabilization (MOMP) is a critical event that facilitates the release of cytochrome c, which then activates caspases and drives the cell toward apoptosis. Moreover, the intricate interplay between mitochondrial dynamics—such as fission and fusion—also influences apoptotic pathways, as healthy mitochondria can mitigate stress-induced signals, whereas damaged mitochondria promote cell death (FALCONE et al.). Recent insights into the DNA damage response (DDR) suggest that mitophagy, a selective degradation process, serves to eliminate dysfunctional mitochondria and thus may either attenuate or amplify apoptotic signals (Pawlowska E et al.). Consequently, the multifaceted involvement of mitochondria in apoptosis illustrates their integral role in cellular homeostasis and the execution of programmed cell death.
B. Implications of mitochondrial dysfunction in diseases related to apoptosis
Mitochondrial dysfunction emerges as a critical factor in various diseases linked to apoptosis, significantly impacting cellular homeostasis and survival. In conditions such as idiopathic pulmonary fibrosis and heart failure, disruptions in mitochondrial function can precipitate pathogenic processes that lead to excessive apoptosis. For example, in idiopathic pulmonary fibrosis, mitochondrial damage activates apoptotic and inflammatory pathways, implicating mitochondrial quality control mechanisms in the diseases progression (Cuevas-Mora et al.). Similarly, research indicates that in heart failure, the interplay of mitochondrial dysfunction with drug-induced cardiac toxicity can induce robust apoptosis, shifting the balance toward cell death and worsening cardiac health (Ganeshan K et al.). These findings underline the importance of mitochondrial integrity in regulating apoptosis and suggest that targeting mitochondrial dysfunction could present new therapeutic avenues for mitigating diseases characterized by excessive cell death. Understanding these implications is essential for developing strategies that preserve mitochondrial function and enhance cellular resilience against apoptosis.
C. Future directions for research on mitochondrial regulation of programmed cell death
As research advances, future explorations into mitochondrial regulation of programmed cell death should focus on elucidating the intricate signaling networks that govern mitochondrial dynamics and their roles in apoptosis. Investigating how post-translational modifications of critical proteins within the BCL-2 family influence their functional interactions can provide new insights into mitochondrial permeability transition and the release of apoptotic factors. Additionally, employing cutting-edge imaging techniques to visualize mitochondrial behavior in real-time during apoptotic events will deepen our understanding of mitochondrial morphology and viability in various cellular contexts. Furthermore, the expanding role of mitochondrial metabolites in modulating apoptosis necessitates a comprehensive analysis of metabolic pathways and their intersection with apoptotic signaling. By integrating these approaches, researchers can unveil novel therapeutic targets that may enhance cell survival in degenerative diseases or promote targeted apoptosis in cancer cells, ultimately advancing the understanding of cellular fate regulation and its implications for human health.
REFERENCES
- A Ashkenazi, A Bakhshi, A Basu, A Gross, A Letai, A Mandic, A Nechushtan, et al.. “Bcl-2 protein family: Implications in vascular apoptosis and atherosclerosis”. ‘Springer Science and Business Media LLC’, 2006, https://core.ac.uk/download/pdf/11738450.pdf
- Elzbieta Pawlowska, Janusz Blasiak, Jolanta Bialkowska-Warzecha, Kai Kaarniranta, Piotr Czarny. “Autophagy in DNA Damage Response”. ‘MDPI AG’, 2015, https://core.ac.uk/download/71973848.pdf
- Arnoult, Damien, Chan, David C., Chen, Hsuichen, Karbowski, et al.. “Quantitation of mitochondrial dynamics by photolabeling of individual organelles shows that mitochondrial fusion is blocked during the Bax activation phase of apoptosis”. ‘Rockefeller University Press’, 2004, https://core.ac.uk/download/4875014.pdf
- Kasi Ganeshan, T., Kasi Ganeshan, T.. “Investigating the protective role of the natural hormone Melatonin, in reducing drug-induced cardiotoxicity in the therapy of chronic diseases”. 2019, https://core.ac.uk/download/287605215.pdf
- Khan, Mohsin, Kim, Seong-Jun, Siddiqui, Aleem, Syed, et al.. “Mitochondrial dynamics and viral infections: A close nexus.”. eScholarship, University of California, 2015, https://core.ac.uk/download/323064408.pdf
- Acquatella, Ahmed, Albakri, Alston, Alston, Arany, Arbustini, et al.. “Mitochondrial Function and Dysfunction in Dilated Cardiomyopathy”. ‘Frontiers Media SA’, 2021, https://core.ac.uk/download/416681591.pdf
- Austin, Grant L., Johnson, Lance A., Sun, Ramon, Young, et al.. “Mitochondrial Metabolism in Major Neurological Diseases”. UKnowledge, 2018, https://core.ac.uk/download/232597633.pdf
- Avolio, Carlo, Bellanti, Francesco, Blonda, Maria, di Bello, et al.. “Synergistic interaction of fatty acids and oxysterols impairs mitochondrial function and limits liver adaptation during nafld progression”. ‘Elsevier BV’, 2018, https://core.ac.uk/download/141696682.pdf
- Conti, Ilaria, Laface, Ilaria, Milani, Daniela, Neri, et al.. “miRNAs as Influencers of Cell-Cell Communication in Tumor Microenvironment”. ‘MDPI AG’, 2020, https://core.ac.uk/download/286469910.pdf
- FALCONE, Claudio, MAZZONI, Cristina. “External and internal triggers of cell death in yeast”. ‘Springer Science and Business Media LLC’, 2016, https://core.ac.uk/download/54531507.pdf
- A Al-Saif, A Bertoni, A Cossarizza, A Ferri, A Israelson, A Raturi, A Valencia, et al.. “Role of mitochondrial raft-like microdomains in the regulation of cell apoptosis”. ‘Springer Science and Business Media LLC’, 2015, https://core.ac.uk/download/54517970.pdf
- Cakouros, Dimitrios, Dorstyn, Loretta, Hay, Bruce A., Huh, et al.. “The role of cytochrome c in caspase activation in Drosophila melanogaster cells”. 2002, https://core.ac.uk/download/4872797.pdf
- Díaz Moreno, Irene, Díaz Quintana, Antonio Jesús, García Heredia, José Manuel, Rosa Acosta, et al.. “Cytochrome c signalosome in mitochondria”. ‘Springer Science and Business Media LLC’, 2011, https://core.ac.uk/download/190375244.pdf
- A Ciechanover, A Criollo, A Degterev, A Degterev, A Degterev, A Gross, A Hochreiter-Hufford, et al.. “Essential versus accessory aspects of cell death: recommendations of the NCCD 2015”. ‘Springer Science and Business Media LLC’, 2014, https://core.ac.uk/download/36778265.pdf
- Frank-Cannon, Tamy Catherine. “Cerebellar Purkinje cell death in the P/Q -type voltage-gated calcium ion channel mutant mouse, leaner”. Texas A&M University, 2006, https://core.ac.uk/download/147124916.pdf
- Cuevas-Mora, Karina, Romero, Freddy, Roque, Willy. “Mitochondrial Quality Control in Age-Related Pulmonary Fibrosis.”. Jefferson Digital Commons, 2020, https://core.ac.uk/download/288122101.pdf
- Kiliańska, Zofia, Żolnierczyk, Jolanta. “Main Pro-Apoptotic Member of BCL-2 Family Proteins-BAX”. ‘Walter de Gruyter GmbH’, 2011, https://core.ac.uk/download/71976739.pdf
- Green, Douglas R, Parsons, Melissa J. “Mitochondria and apoptosis: a quick take on a long view”. Biology Reports Ltd, 2025, https://core.ac.uk/download/pdf/8441549.pdf
Image References:
- “Molecular mechanisms of apoptosis involving BCL-2 family proteins..” media.springernature.com, 9 January 2025, https://media.springernature.com/full/springer-static/image/art%3A10.1038%2Fs41580-023-00629-4/MediaObjects/41580_2023_629_Fig1_HTML.png
- “Mitochondrial pathway of apoptosis highlighting key regulatory proteins..” pub.mdpi-res.com, 9 January 2025, https://pub.mdpi-res.com/ijms/ijms-23-02357/article_deploy/html/images/ijms-23-02357-g003.png?1645507570