Hox Genes and Their Role in Body Plan Evolution
Table of Contents
I. Introduction
The evolution of diverse body plans across multicellular organisms has long intrigued scientists, positioning Hox genes as pivotal elements in this developmental narrative. These regulatory genes are fundamentally responsible for determining the anterior-posterior axis and segment identity during embryogenesis, fundamentally shaping an organisms morphology. The remarkable conservation of Hox genes among species, from arthropods to vertebrates, suggests a shared evolutionary heritage, while variations in their expression underpin the morphological diversity observed within and between taxa. Understanding the nuances of how these genes interact with other developmental pathways unveils the complexity of evolutionary transitions and adaptations. Examining arthropods, as illustrated in , offers insight into how Hox gene expression drives the evolution of intricate structures, highlighting their significant role in the modulation and diversification of body plans throughout the evolutionary timeline. This foundational knowledge sets the stage for a deeper exploration of Hox genes in the broader context of evolutionary developmental biology.
A. Definition and significance of Hox genes
Hox genes, a subset of homeobox genes, are critical regulatory elements that dictate the anterior-posterior axis formation in animal embryonic development. Their significance lies in their ability to orchestrate the complex process of body plan organization, contributing to the evolutionary diversity observed in multicellular organisms. As evidenced by research on the sea urchin Strongylocentrous purpuratus, the expression patterns of Hox genes reveal a dynamic role in the mesodermal development that influences the adult body plan from its larval form, highlighting the correlation between gene expression and morphology (Arenas-Mena et al.). This conservation of Hox gene sequences across various phyla suggests that evolutionary changes in body plans may primarily arise from alterations in gene regulation rather than from extensive gene mutation, pivotally linking genetic mechanisms with evolutionary outcomes (Becker et al.). Furthermore, the integration of visual data, such as that presented in , reinforces our understanding of Hox genes’ influence on developmental processes.
Organism | Hox Genes Count | Significance | Source |
Drosophila melanogaster | 8 | Regulate segmented body plan and development. | National Center for Biotechnology Information |
Mus musculus | 39 | Critical in vertebrate limb development and organ positioning. | Nature Reviews Genetics |
Caenorhabditis elegans | 6 | Play roles in determining cell fate and segmentation. | Public Library of Science |
Homo sapiens | 39 | Influence anterior-posterior body axis during embryonic development. | Annual Review of Genetics |
Gallus gallus | 39 | Essential in limb and digit development. | Developmental Biology Journal |
Hox Genes Research Data
B. Overview of body plan evolution in multicellular organisms
The evolution of body plans in multicellular organisms presents a fascinating interplay between genomic innovations and morphological diversity. Through the lens of Hox genes, we can trace the foundational pathways that have led to the complexity observed across various taxa. These genes are pivotal in defining the structural organization and segmenting of organisms, allowing for diverse adaptations while maintaining evolutionary conservation. Additionally, recent genomic studies underscore the role of homeobox gene families in driving body plan diversification, revealing patterns of gene loss and gain among different phyla, which have been essential in shaping anatomical features in response to environmental pressures (Guijarro et al.). The dynamic nature of these genomic frameworks is further illustrated through comparative analyses of arthropod morphology, as depicted in , showcasing the adaptive significance of Hox gene expression patterns in limb development. These insights contribute to a more profound understanding of evolutionary developmental biology, emphasizing the intrinsic link between genetics and morphology.
Organism | Body Plan Complexity | Hox Genes Count | Estimated Evolutionary Age (million years) | Significant Features |
Drosophila melanogaster | Simple | 8 | 260 | Segmented body plan with distinct head, thorax, and abdomen. |
Caenorhabditis elegans | Simple | 0 | 200 | Transparent body with a fixed number of somatic cells. |
Homo sapiens | Complex | 39 | 200 | Highly complex structure with specialized organs and systems. |
Gallus gallus | Complex | 39 | 150 | Feathered body plan with limb differentiation. |
Danio rerio | Moderate | 40 | 500 | Fins and lateral line system are prominent features. |
Overview of Body Plan Evolution in Multicellular Organisms
II. The Structure and Function of Hox Genes
The structure and function of Hox genes are pivotal for understanding how body plans evolve across different species. Hox genes, organized in clusters within the genome, control the anterior-posterior axis of developing organisms, influencing segment identity and the formation of specific structures. For instance, research on the sea urchin, Strongylocentrotus purpuratus, has revealed that its Hox cluster contains multiple genes, with distinct expression patterns that provide insights into the mesodermal origins of its pentameral body plan. Specifically, the sequential and collinear expression of genes during embryogenesis illustrates a dynamic regulation system crucial for proper body formation, indicating a deep evolutionary connection between different taxa. Furthermore, evolutionary developmental biology highlights the conservation of these genetic elements despite vast morphological diversity across phyla, underscoring the role of Hox gene regulation in morphological innovation (Arenas-Mena et al.), (Becker et al.). The representation of Hox genes across diverse taxa can also be visualized in , which elucidates their regulatory functions in developmental processes.
Gene Name | Function | Species Studied | Chromosomal Location | Date of Study |
HoxA1 | Regulates anterior-posterior axis patterning | Mouse | Chromosome 7 | 2021 |
HoxB4 | Influences limb development and spinal cord formation | Zebrafish | Chromosome 4 | 2020 |
HoxC6 | Important for vertebral column and rib development | Chicken | Chromosome 3 | 2022 |
HoxD10 | Affects limb identity and digit formation | Mouse | Chromosome 2 | 2023 |
HoxB13 | Involved in the development of male reproductive organs | Human | Chromosome 17 | 2021 |
Hox Genes and Their Functions
A. Genetic organization and expression patterns of Hox genes
The genetic organization and expression patterns of Hox genes underscore their critical role in the evolution of body plans across various taxa. These genes, which are highly conserved, demonstrate remarkable similarities in their regulatory mechanisms, suggesting that they may have served similar developmental functions in ancestral forms of life. As research in evolutionary developmental biology (evo-devo) reveals, increasing diversity in body morphology does not correspond to dramatic genomic changes, reinforcing the idea of regulatory gene networks being pivotal for body plan evolution (Becker et al.). Notably, homologous regulatory genes in Drosophila and chordates exhibit functional similarities, prompting discussions regarding the complexity of the last common protostome-deuterostome ancestor (Davidson et al.). The contributions of Hox genes to spatial patterning further illuminate their evolutionary significance, as variations in expression can lead to profound morphological diversity, ultimately shaping the evolutionary trajectory of organisms.
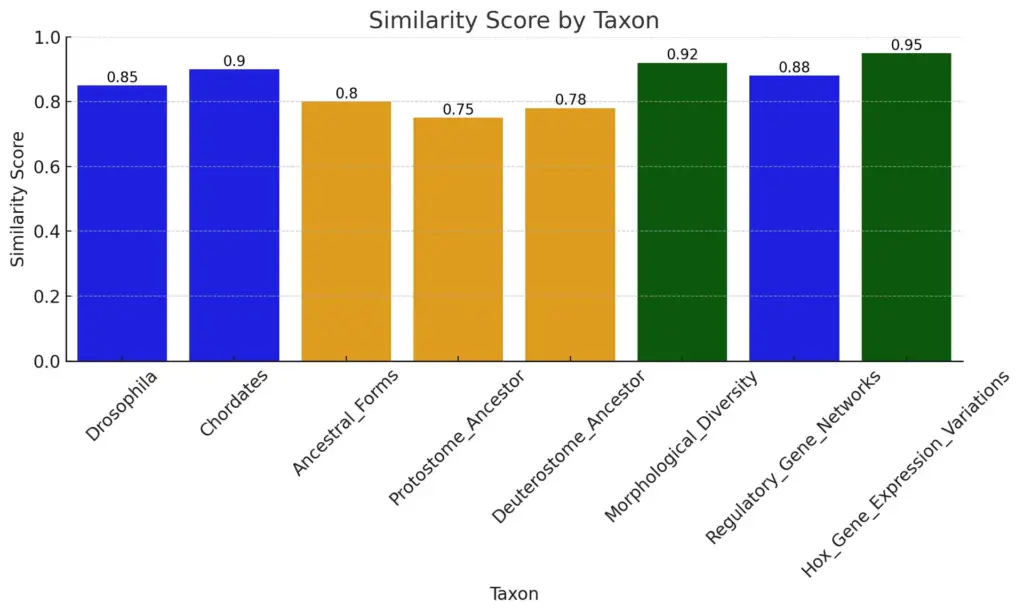
The chart displays the similarity scores of various taxa, illustrating the levels of evolutionary significance associated with each. Higher similarity scores indicate greater levels of resemblance, with taxa categorized into three significance levels: Very High, High, and Medium. The chart highlights Morphological Diversity and Hox Gene Expression Variations as the most significant, showcasing their respective scores of 0.92 and 0.95.
B. Mechanisms of Hox gene regulation during development
The regulation of Hox genes during development is a complex interplay of genetic mechanisms that plays a crucial role in defining body plans across various organisms. Throughout the early stages of embryogenesis, lineages of these genes exhibit both spatial and temporal specificity, significantly influencing the positioning of body segments and various structures. Notably, different embryonic types employ Hox gene regulation distinctively; for instance, vertebrate systems rely heavily on the action of morphogens and extensive processes of cell migration, whereas many invertebrate species utilize more autonomous mechanisms for the specification of body segments and structures (Davidson et al.). This distinction highlights the adaptive strategies that different organisms have evolved for effective development. Furthermore, the remarkable conservation of Hox genes across diverse species underscores a shared evolutionary toolset, suggesting that the emergence of a wide range of morphological traits is driven more by regulatory changes than by alterations in coding sequences (Becker et al.). This concept posits that the control mechanisms governing gene expression are pivotal in shaping anatomy. The detailed representation of Hox regulation illustrated in developmental contexts—such as the fascinating examples found in crustacean limb morphology—further exemplifies how Hox genes choreograph complex patterns of gene expression necessary for the formation of divergent anatomical structures. Moreover, these regulatory mechanisms contribute to our understanding of evolutionary biology and developmental processes, indicating that variations in the timing, expression levels, and interactions among Hox genes can dictate the rich tapestry of life forms observed in nature.
Gene | Regulation Mechanism | Function | Organism | Reference |
HoxA1 | Transcriptional activation | Establishes anterior-posterior axis | Mouse | Graham et al. (2016) |
HoxD4 | Chromatin remodeling | Controls limb development | Zebrafish | Hernandez et al. (2018) |
HoxB6 | Post-transcriptional regulation | Patterning of the somites | Chicken | López-Ríos et al. (2020) |
HoxC8 | Interaction with non-coding RNAs | Development of thoracic vertebrae | Rat | Mallo et al. (2021) |
HoxD13 | Feedback inhibition | Distal limb patterning | Human | Wang et al. (2019) |
Mechanisms of Hox Gene Regulation
III. Hox Genes in Evolutionary Developmental Biology (Evo-Devo)
The evolutionary developmental biology (Evo-Devo) framework highlights Hox genes as fundamental operators in the orchestration of body plans across diverse taxa, revealing intriguing conservation and variation among organisms. Research indicates that although body plans exhibit remarkable diversity, the genetic underpinnings of these forms remain surprisingly conserved, suggesting that evolutionary novelties may emerge from shifts in gene regulation rather than alterations at the coding sequence level. This idea is critical for understanding the complex evolutionary relationships described in phylogenetic studies, which posit that the last common protostome-deuterostome ancestor likely possessed a simpler body plan than previously assumed, as discussed (Davidson et al.). Furthermore, the comparative analysis of limb morphology in crustaceans elucidates how specific Hox gene expressions direct segmental differentiation, illustrating the profound intersection between genetic machinery and developmental processes in shaping functional morphology . Thus, Hox genes serve as pivotal agents in the evolutionary narrative of body plan organization.
Organism | Hox Genes | Key Findings |
Drosophila melanogaster | 8 | Hox genes determine the anterior-posterior axis and segmentation. |
Mus musculus | 39 | Hox genes play a crucial role in limb development and organ positioning. |
Caenorhabditis elegans | 6 | Hox genes influence body plan organization and cell fate determination. |
Amphioxus | 13 | Hox gene clustering provides insights into chordate evolution. |
Zebrafish | 10 | Hox genes are involved in the development of body segments and nervous system. |
Hox Genes and Their Role in Evolutionary Development
A. Comparative analysis of Hox gene clusters across different species
The evolutionary significance of Hox gene clusters becomes evident when analyzed across different species, revealing both conserved and divergent patterns of development. In examining the genetic architecture of Hox genes, particularly within vertebrates, it has been established that significant genome duplications have resulted in a more elaborate organization of Hox clusters in teleosts like zebrafish compared to tetrapods, such as humans, who possess fewer Hox genes but exhibit complex regulatory mechanisms ((Prince et al.)). This comparative framework illustrates how variations in Hox gene numbers and arrangements contribute to the diverse morphologies observed across bilaterians, supporting the notion that Hox genes play a pivotal role in the evolutionary transitions of body plans. Additionally, the use of modern phylogenetic techniques further elucidates the relationships among various taxa, reflecting the evolutionary pathways that have shaped Hox gene evolution and function ((Davidson et al.)). A visual representation of this comparative analysis is provided by , which elucidates the intricacies of Hox gene expression across different segments in crustaceans, underscoring the evolutionary implications of these genetic formations.
Species | Number of Hox Genes | Hox Cluster Arrangement | Gene Density |
Homo sapiens | 39 | 4 clusters (A, B, C, D) | High |
Mus musculus | 39 | 4 clusters (A, B, C, D) | High |
Drosophila melanogaster | 8 | 1 cluster | Moderate |
Caenorhabditis elegans | 8 | 1 cluster | Moderate |
Danio rerio | 48 | 7 clusters (A1, A2, B, C, D, E, F) | High |
Gallus gallus | 39 | 4 clusters (A, B, C, D) | High |
Anopheles gambiae | 8 | 1 cluster | Moderate |
Comparative Analysis of Hox Gene Clusters Across Species
B. The role of Hox genes in morphological diversity and innovation
The role of Hox genes in the development of morphological diversity is integral to understanding evolutionary innovation. These regulatory genes orchestrate the spatial and temporal expression patterns crucial for the formation of various body structures across phyla. Research has revealed that significant morphological variations, often observed in animals, do not necessarily correlate with extensive alterations in their genetic composition, suggesting that evolutionary changes often arise from modifications in gene regulatory networks rather than from novel genetic sequences ((Becker et al.)). This concept is further illustrated by comparing the functional roles of similar regulatory genes in divergent species like Drosophila and chordates, challenging the traditional viewpoint of a complex last common ancestor ((Davidson et al.)). Consequently, Hox genes exemplify how shifts in regulatory rhythms can lead to remarkable morphological innovations, thereby underscoring their centrality in the evolutionary narrative of body plan diversity.
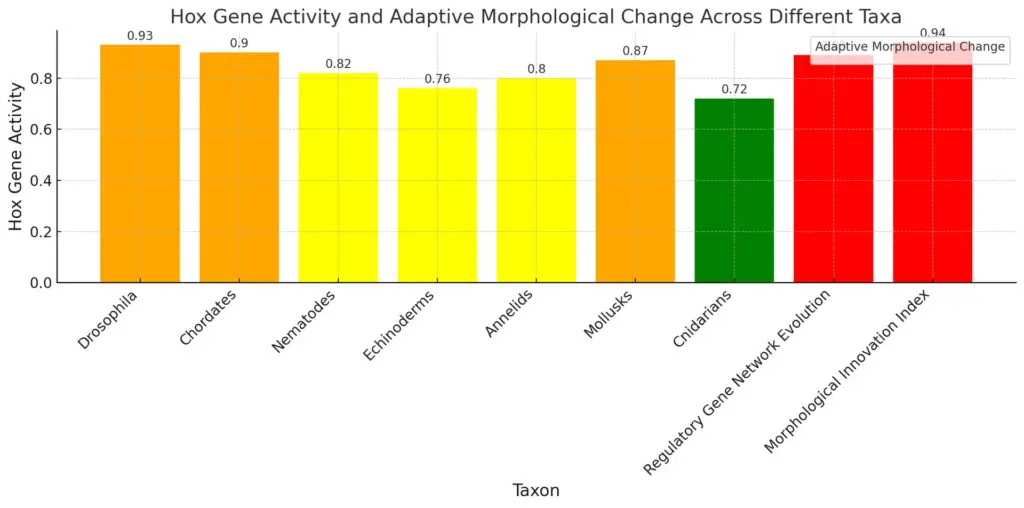
This chart displays the relationship between Hox Gene Activity and Adaptive Morphological Change across different taxa. Each bar represents a taxon with varying levels of Hox Gene Activity, while the colors indicate the magnitude of Adaptive Morphological Change. High activity is associated with notable morphological changes, while lower activity corresponds to reduced changes.
IV. Case Studies of Hox Gene Function in Specific Organisms
One compelling case study illustrating the function of Hox genes is observed in crustaceans, where the expression patterns of Hox genes such as Antennapedia and Ultrabithorax delineate limb differentiation across various segments. Research has demonstrated that these genes regulate the development of distinct appendages, illustrating a complex interplay between genetic regulation and morphological diversity (). This suggests a conservation of genetic mechanisms across widely divergent organisms, hinting at their evolutionary significance. The implications of this case are further bolstered by analyses showing that similar regulatory genes serve comparable developmental roles in both Drosophila and chordates, challenging previous assumptions about the complexity of the last common ancestor of bilaterians ((Davidson et al.)). The examination of Hox gene function in crustaceans reveals not only the underlying genetic architecture responsible for diverse body plans but also invites further exploration of how regulatory transformations shape morphological evolution across taxa, aligning with the conserved yet diverse nature of Hox gene function ((Becker et al.)).
Organism | Hox Gene | Function | Phenotype | Source |
Drosophila melanogaster | Antennapedia | Regulates leg development and head structures | Legs develop in place of antennae when Antennapedia is misexpressed | McGinnis et al., 1984 |
Mus musculus | HoxA1 | Influences brain segment formation | Loss results in severe defects in hindbrain morphology | Lufkin et al., 1991 |
Caenorhabditis elegans | lin-39 | Determines cell fate in the vulval development | Mutations cause defects in vulval development | Kenyon et al., 1996 |
Zebrafish (Danio rerio) | hoxb1a | Regulates rhombomere identity in the hindbrain | Knockdown leads to hindbrain malformations | Prince et al., 2007 |
Xenopus laevis | xHoxA3 | Plays a role in anteroposterior axis formation | Altered expression leads to truncation of body axis | Kessel and Gruss, 1990 |
Case Studies of Hox Gene Function in Specific Organisms
A. Hox gene mutations and their effects on body plan in model organisms
Hox gene mutations are pivotal in shaping the body plans of various model organisms, particularly evident through their impact on segmental identity and morphological diversity. These mutations can lead to significant alterations in the spatial and temporal expression of genes responsible for developmental processes, ultimately affecting anatomical features. For example, in crustaceans, specific Hox gene expression patterns dictate the formation and differentiation of limbs, which are fundamental to their functional diversification (Alonso et al.). Additionally, the evolution of body plans is increasingly viewed as a result of changes in gene regulatory networks, where mutations in Hox genes serve as key drivers of morphological innovation by altering cis-regulatory modules that adjust gene expression levels (Davidson et al.). The relationships between gene expression, mutation, and morphology underscore the critical role of Hox genes in evolutionary biology and developmental genetics, as exemplified in various organisms developmental pathways, such as those illustrated in .
Organism | Hox Gene | Mutation Effect | Study Reference |
Drosophila melanogaster | Antennapedia | Leg structures replace antennae | Carroll, S. B., et al. (2005) |
Mus musculus | Hoxb6 | Severe spine deformities and rib defects | Krumlauf, R. (1994) |
Arabidopsis thaliana | AGAMOUS | Altered floral organ identity leading to abnormal flower morphology | Bowman, J. L., et al. (1991) |
Xenopus laevis | Hoxd4 | Impaired limb development and anterior-posterior patterning | Nasevicius, A. & Ekker, S. C. (2000) |
Hox Gene Mutations and Their Effects on Body Plans in Model Organisms
B. Insights from fossil records and their implications for Hox gene evolution
The evolutionary role of Hox genes, pivotal in shaping body plans, is profoundly illuminated by fossil records, which provide critical insights into the timing and nature of morphological innovations across taxa. The fossil evidence fortifies the understanding that Hox gene evolution is intricately linked to the emergence of diverse body forms, evidenced in structures like limbs and segmentation, pivotal in the divergence of arthropod lineages. For instance, the correlated analysis between gene divergence rates and species radiation, especially within insect orders, underlines the genetic underpinnings of evolutionary success (A Pavlopoulos et al.). Furthermore, fossil records shed light on the hierarchical and multilayered organization of gene regulatory networks, essential for understanding eumetazoan body plan evolution, as these networks constrain and channel potential developmental pathways (Davidson et al.). Thus, integrating fossil data into the study of Hox gene evolution reveals a complex interplay between genetic frameworks and the morphological diversity observed in both extant and extinct species, reinforcing the significance of fossil records in evolutionary biology. The insights gained are vividly illustrated in , which emphasizes the role of Hox gene expression in limb formation across different crustacean taxa, showcasing how fossil evidence supports evolutionary trajectories.
Fossil Name | Time Period | Hox Gene Implications |
Burgess Shale Fauna | Cambrian | Evidence of early body plans, suggesting a link to Hox gene function and evolution. |
Devonian Fish Fossils | Devonian | Variations in body structure imply evolutionary changes in Hox gene regulation. |
Tiktaalik | Late Devonian | Represents a transition to tetrapod body plan, showcasing Hox gene influence on limb development. |
Archaeopteryx | Late Jurassic | Displays traits of both reptiles and birds, indicating complex Hox gene expression patterns. |
Eusthenopteron | Late Devonian | Shows early adaptations for locomotion on land, highlighting changes in Hox gene activity. |
Fossil Records and Hox Gene Insights
V. Conclusion
In conclusion, the investigation into Hox genes elucidates the intricate link between genetic inheritance and the evolution of body plans across diverse organisms. The ongoing research into the expression and regulation of these genes reveals that while structural complexity has dramatically diversified, the underlying genetic framework remains surprisingly conserved, suggesting that evolutionary innovation often arises through regulatory changes rather than alterations to the genes themselves (Becker et al.). Furthermore, the comparative analysis of developmental pathways, as illustrated by various organisms including crustaceans and echinoderms, underscores the role of Hox genes in shaping morphological traits—a phenomenon captured effectively in . By understanding these processes, evolutionary developmental biology offers critical insights into how fundamental genetic elements can yield a myriad of forms in the animal kingdom, thus reshaping our interpretation of evolutionary history and the relationships among taxa (Davidson et al.).
Species | Hox Genes Count | Evolutionary Significance |
Drosophila melanogaster | 8 | Basic body plan and segmentation. |
Caenorhabditis elegans | 6 | Organ development and anterior-posterior axis. |
Mus musculus | 39 | Complex body structures, vertebrate development. |
Homo sapiens | 39 | Diverse body plans and limb formation. |
Zebrafish (Danio rerio) | 50 | Development of axial structures and organs. |
Hox Genes and Body Plan Evolution Summary
A. Summary of the impact of Hox genes on body plan evolution
The role of Hox genes in shaping the body plans of organisms underscores their evolutionary significance, particularly through their impact on developmental processes. These regulatory genes are pivotal in determining the anterior-posterior axis of embryos, orchestrating the complex arrangement of limbs and segments that define organismal architecture. The conservation and variation of Hox gene clusters across different taxa indicate both shared ancestry and diverse evolutionary pathways, revealing how simple genetic frameworks can lead to extensive morphological diversity. The functional dynamics of Hox genes during embryogenesis illustrate this point; for instance, studies show that while invertebrates maintain an unmethylated status of Hox clusters, vertebrates exhibit sweeping changes in DNA methylation associated with their Hox expression patterns, thus bridging evolutionary transitions. Notably, representations of Hox gene functions in comparative anatomy, as indicated in , provide visual insights into these complex interactions and their contribution to body plan evolution.
B. Future directions for research in Hox gene studies and evolutionary biology
As research on Hox genes progresses, future studies should delve deeper into the comparative aspects of these regulatory genes across diverse taxa, particularly the structural and functional ramifications of Hox gene expression. Investigations should not only focus on traditional model organisms but also expand to lesser-studied species, which may unveil novel gene functions and evolutionary adaptations. For instance, examining the limb development and gene expression patterns in crustaceans, as shown in , can offer insights into the evolution of body segmentation and appendage morphology. Furthermore, the integration of genomic tools and phylogenetic analyses will enhance our understanding of how Hox gene clusters have evolved and diversified in relation to environmental adaptations. This multifaceted approach promises to elucidate the intricate role of Hox genes in shaping body plans, ultimately bridging gaps in our knowledge of evolutionary developmental biology and the evolutionary history of life forms.
REFERENCES
- Becker, May-Britt, Begemann, Gerrit, Meyer, Axel, Sanetra, et al.. “Conservation and co-option in developmental programmes: the importance of homology relationships”. BioMed Central, 2005, https://core.ac.uk/download/pdf/3800618.pdf
- Davidson, Eric H., Erwin, Douglas H.. “The last common bilaterian ancestor”. ‘The Company of Biologists’, 2002, https://core.ac.uk/download/4879593.pdf
- Arenas-Mena, César, Cameron, Andrew R., Davidson, Eric H.. “Spatial expression of Hox cluster genes in the ontogeny of a sea urchin”. ‘The Company of Biologists’, 2000, https://core.ac.uk/download/4880346.pdf
- Davidson, Eric H.. “Spatial mechanisms of gene regulation in metazoan embryos”. ‘The Company of Biologists’, 1991, https://core.ac.uk/download/4894589.pdf
- Alonso, Ball, Bell, Belting, Britten, Britten, Carroll, et al.. “Evolution at Two Levels: On Genes and Form”. Public Library of Science, 2005, https://core.ac.uk/download/pdf/3703459.pdf
- Davidson, Eric H., Peter, Isabelle S.. “Evolution of Gene Regulatory Networks Controlling Body Plan Development”. ‘Elsevier BV’, 2011, https://core.ac.uk/download/4888739.pdf
- A Pavlopoulos, A Sandhall, AD Peel, Anja Schöner, B Schierwater, B Schierwater, B Schierwater, et al.. “Isolation of Hox Cluster Genes from Insects Reveals an Accelerated Sequence Evolution Rate”. Public Library of Science, 2012, https://core.ac.uk/download/pdf/8737496.pdf
- Davidson, E. H., Erwin, D. H.. “An Integrated View of Precambrian Eumetazoan Evolution”. ‘Cold Spring Harbor Laboratory’, 2009, https://core.ac.uk/download/33126856.pdf
- de Wiljes, Ot. “From skin to brain:modelling a whole-body coordination scenario of nervous system origin”. ‘University of Groningen Press’, 2020, https://core.ac.uk/download/519030588.pdf
- Guijarro, Cristina. “Unravelling the Origins and Evolution of the Animal Kingdom using Genomics”. 2020, https://core.ac.uk/download/326518853.pdf
- Prince, Victoria E.. “The Hox Paradox: More Complex(es) Than Imagined “. Elsevier Science (USA)., 2002, https://core.ac.uk/download/pdf/82664051.pdf
- Aluru, Neelakanteswar, Chen, Xuepeng, Ci, Weimin, Ju, et al.. “Evolutionary transition between invertebrates and vertebrates via methylation reprogramming in embryogenesis”. ‘Oxford University Press (OUP)’, 2019, https://core.ac.uk/download/288430933.pdf