Intracellular Bacteria: Exploring the Hidden World of Chlamydia, Rickettsia, and Mycobacteria
Table of Contents
I. Introduction to Intracellular Bacteria
Intracellular bacteria are a varied group of germs that can live and multiply inside host cells, greatly changing how host-pathogen interactions work. This special way of living creates big challenges for the immune system and makes it harder to treat infections from these germs. This group includes known types like Chlamydia, Rickettsia, and Mycobacteria, which have developed unique methods to get around host defenses, control key cellular processes, and avoid immune responses. For example, these germs can smartly take over the host’s cellular systems to build specific environments that help them survive, grow, and stay in the body, often resulting in different diseases. Understanding the details of these complicated relationships is important not just for basic scientific knowledge but also for creating effective treatment plans against these intracellular germs. A visual aid, on this, can show the complex interactions between different intracellular bacteria and host cells, pointing out the clever ways these germs change host cellular functions to gain an advantage. Such knowledge is crucial for understanding these mysterious organisms and their significant effects on human health, especially concerning new infectious diseases. Additionally, these topics are becoming more important due to the growing problems from antibiotic resistance, which continue to challenge modern medicine and public health efforts worldwide. As we explore intracellular bacteria more, it becomes clear that understanding them is essential for developing new methods to lessen their impact on health and disease management.
Table : Introduction to Intracellular Bacteria – Intracellular bacteria are a unique group of microorganisms that live inside host cells, either as obligate (requiring host cells for survival) or facultative (capable of living both inside and outside host cells). These bacteria play essential roles in health, disease, and even symbiotic relationships.
# | Type of Intracellular Bacteria | Definition | Mode of Infection/Survival | Examples | Associated Diseases or Functions |
---|---|---|---|---|---|
1 | Obligate Intracellular Bacteria | Bacteria that can only survive inside host cells | Avoids immune detection, relies on host nutrients | Chlamydia trachomatis | Chlamydia (STI), trachoma (eye infection) |
2 | Facultative Intracellular Bacteria | Bacteria that can live inside or outside host cells | Adapts to host or free-living conditions | Salmonella enterica | Typhoid fever, food poisoning |
3 | Pathogenic Intracellular Bacteria | Cause diseases by invading and multiplying in host cells | Evades immune responses, disrupts cellular function | Mycobacterium tuberculosis | Tuberculosis (TB) |
4 | Symbiotic Intracellular Bacteria | Live inside host cells, providing mutual benefits | Aid in nutrient synthesis, immunity, or development | Wolbachia | Controls insect reproduction, protects against viruses |
5 | Endosymbiotic Bacteria | Exist within host cells as permanent symbionts | Provide essential nutrients to the host | Buchnera aphidicola | Provides amino acids to aphids |
6 | Bacteria That Escape Phagocytosis | Evade destruction by immune cells | Resist lysosomal degradation, replicate in phagosomes | Legionella pneumophila | Legionnaires’ disease |
7 | Bacteria That Modify Host Cell Function | Manipulate host cell processes for survival | Alter immune signaling, cell division, or apoptosis | Rickettsia rickettsii | Rocky Mountain spotted fever |
8 | Bacteria That Form Intracellular Biofilms | Create protective communities inside host cells | Resist antibiotics and immune responses | Brucella spp. | Brucellosis (zoonotic disease) |
9 | Bacteria That Hijack Host Metabolism | Use host cellular resources for growth | Redirects host energy production and metabolism | Coxiella burnetii | Q fever |
10 | Bacteria That Spread Cell-to-Cell | Move directly between host cells to avoid immune detection | Utilize actin-based motility or other strategies | Listeria monocytogenes | Listeriosis (foodborne illness) |
Bacteria Type | Global Prevalence (millions) | Countries with Highest Cases | Common Symptoms |
Chlamydia | 127 | USA, India, China | Asymptomatic, Urogenital infections |
Rickettsia | 1.5 | India, Brazil, Vietnam | Fever, Rash, Headache |
Mycobacteria | 10 | India, China, Indonesia | Cough, Chest Pain, Fatigue |
Prevalence of Intracellular Bacteria Infections by Bacteria Type
A. What Makes Some Bacteria Live Inside Host Cells?
Certain bacteria can live inside host cells due to their advanced adaptations that allow them to control the host’s cellular functions in different ways. Many pathogens, like Chlamydia, Rickettsia, and Mycobacteria, have changed significantly over time to create specialized methods that not only let them enter host cells but also help them avoid detection by the host’s immune system. These intracellular bacteria use clever strategies to take advantage of cellular resources, which are key for their survival and reproduction in the challenging environment of the host. For example, these pathogens can significantly change host cell signaling pathways to support their life cycle, alter nutrient uptake processes to ensure a regular supply of necessary elements, and create localized environments that support their growth and reproduction. Understanding the complexities of these interactions is vital for a full understanding of bacterial infection processes, especially concerning how they attract specific host proteins and effectively use cellular structures to improve their own survival while dodging immune responses. The diagram showing these complex processes, especially detailing how intracellular bacteria influence cellular pathways, clearly illustrates the diverse nature of these bacterial lives. It highlights the vital relationship between host cells and harmful bacteria, revealing the intricate dynamics of infectious diseases, which ultimately helps in developing potential treatments and public health strategies to fight these harmful pathogens.
Table : List of 20 Key Factors That Enable Bacteria to Live Inside Host Cells (This expanded table includes 20 key factors that allow bacteria to survive and thrive inside host cells)
Factor No. | Factor | Description | Examples of Bacteria | Role in Intracellular Survival |
---|---|---|---|---|
1 | Adhesion to Host Cells | Surface proteins help bacteria attach to host cell receptors | Listeria monocytogenes, Salmonella enterica | Enables bacteria to initiate infection |
2 | Invasion Mechanisms | Bacteria use invasins to enter host cells | Chlamydia trachomatis, Shigella flexneri | Forces host cells to engulf bacteria via endocytosis |
3 | Evasion of Immune System | Blocks recognition by immune cells or hides inside host cells | Mycobacterium tuberculosis, Coxiella burnetii | Prevents detection and destruction by the immune system |
4 | Survival in Phagosomes | Resists destruction inside host immune cells | Legionella pneumophila, Brucella abortus | Modifies host cell compartments to avoid killing |
5 | Escape from Phagosomes | Breaks free from host vacuoles to replicate in the cytoplasm | Listeria monocytogenes, Rickettsia spp. | Allows bacteria to move freely inside the host cell |
6 | Nutrient Scavenging | Extracts nutrients from the host cell | Chlamydia trachomatis, Mycobacterium leprae | Supplies essential molecules for bacterial growth |
7 | Modifying Host Cell Functions | Alters host signaling pathways to benefit bacterial survival | Salmonella typhimurium, Bartonella henselae | Suppresses host immune response and promotes persistence |
8 | Actin-Based Motility | Hijacks host actin to move between cells | Listeria monocytogenes, Shigella flexneri | Spreads infection without exposure to the immune system |
9 | Biofilm Formation Inside Cells | Creates protective bacterial communities inside host tissues | Brucella spp., Helicobacter pylori | Enhances resistance to antibiotics and immune responses |
10 | Chronic Persistence Mechanisms | Enters a dormant state to evade immune clearance | Mycobacterium tuberculosis, Coxiella burnetii | Enables long-term infection and latent disease |
11 | Antigenic Variation | Alters surface proteins to evade immune recognition | Borrelia burgdorferi, Neisseria gonorrhoeae | Prevents immune system from recognizing and attacking bacteria |
12 | Secretion Systems (Type III, IV, VI, etc.) | Injects bacterial proteins into host cells to manipulate functions | Salmonella, Yersinia pestis, Legionella pneumophila | Helps bacteria control host immune response and cell survival |
13 | Intracellular Acid Resistance | Can survive in acidic environments inside host cells | Helicobacter pylori, Salmonella typhimurium | Allows survival in harsh environments like lysosomes |
14 | Quorum Sensing Regulation | Uses chemical signals to regulate infection-related genes | Pseudomonas aeruginosa, Vibrio cholerae | Enables bacteria to coordinate survival and virulence |
15 | Host DNA Manipulation | Modifies host DNA to suppress immune responses | Chlamydia trachomatis, Bartonella henselae | Helps bacteria alter host cell behavior for survival |
16 | Iron Scavenging Mechanisms | Uses siderophores to capture iron from host cells | Mycobacterium tuberculosis, Yersinia pestis | Ensures bacteria get essential nutrients for growth |
17 | Delayed Apoptosis Induction | Prevents host cells from dying too quickly | Rickettsia rickettsii, Coxiella burnetii | Extends bacterial replication time inside the host |
18 | Efflux Pumps for Antibiotic Resistance | Pumps out antibiotics to avoid being killed | Mycobacterium tuberculosis, Salmonella enterica | Enhances bacterial survival against antibiotic treatments |
19 | Intracellular Stress Response Mechanisms | Adapts to oxidative stress and nutrient limitations | Legionella pneumophila, Francisella tularensis | Allows bacteria to endure harsh intracellular conditions |
20 | Endosymbiosis in Host Cells | Forms long-term symbiotic relationships with hosts | Wolbachia, Buchnera aphidicola | Provides mutual benefits such as nutrient production |
B. Differences Between Obligate and Facultative Intracellular Bacteria
The distinction of intracellular bacteria into obligate and facultative groups shows key differences in how they survive and cause illness, which is important for knowing their roles in disease. Obligate intracellular bacteria, like Chlamydia and Rickettsia, rely entirely on a host cell to replicate, meaning they cannot live or reproduce outside of the host. These bacteria have developed complex ways to avoid the host’s immune system while also manipulating the host cell processes for their own growth and survival. For example, they might disrupt the host’s signaling pathways or block apoptosis, creating a better environment for their development. In contrast, facultative intracellular bacteria, such as Mycobacterium tuberculosis, can live both inside and outside of cells, demonstrating significant adaptability. This ability allows facultative bacteria to survive in different settings, giving them a wider range of survival tactics, which helps them endure under varying conditions or threats. This adaptability makes treatment more difficult, as these bacteria can evade the immune system’s detection and adjust to antibiotic treatments. A visual display of different harmful bacteria interacting with host cells can clearly show these differing lifestyles and the advanced adaptations these organisms use for survival and growth against host defenses. Recognizing these differences is vital for creating targeted treatments for infections from these varied bacterial pathogens, as treatment plans may need to differ greatly between obligate and facultative intracellular bacteria to improve effectiveness and reduce resistance.
Type | Examples | Dependence on Host | Nutritional Source | Host Interaction | Transmission |
Obligate Intracellular Bacteria | Chlamydia, Rickettsia | Cannot reproduce outside host cells | Dependent on host for ATP and metabolites | Generally pathogenic | Transmission often requires compromised host conditions or vectors |
Facultative Intracellular Bacteria | Mycobacterium, Listeria | Can reproduce inside and outside host cells | Can utilize nutrients independent of host | Can be pathogenic or non-pathogenic | Often spread through environmental sources or contaminated food |
Comparison of Obligate and Facultative Intracellular Bacteria
II. Common Intracellular Bacterial Pathogens
Intracellular bacterial pathogens are a complicated area to study because they can invade host cells and change host cellular functions to survive and grow. Important examples include Chlamydia trachomatis, Rickettsia spp., and Mycobacterium tuberculosis, each with its own methods and relationships with host cells. For example, Chlamydia makes use of the inside of cells to avoid detection by the immune system while allowing itself to multiply in a specific space called inclusions. This method protects the bacteria from immune attacks and creates a suitable environment for its growth. Rickettsia takes a different path by using actin polymerization to move and spread to nearby cells. This active process, which various studies have shown, helps Rickettsia invade other tissues and cause infections. Meanwhile, Mycobacterium tuberculosis is known for its ability to survive inside macrophages. It changes the host cell signaling to prevent cell death and improve its survival, enabling it to avoid destruction by the immune response. Knowing these complex actions is important for creating targeted treatments and effective public health measures against the diseases these intracellular pathogens cause. The specific ways these bacteria function underline the important role of host-pathogen interactions in infectious diseases. By continuing to research these interactions, scientists might find new ways to fight these persistent intracellular threats and enhance the health outcomes for individuals affected by these infections.
Pathogen | Disease | Transmission | Estimated Cases per Year (U.S.) | Infection Type |
Chlamydia trachomatis | Chlamydia Infection | Sexual contact, maternal-fetal | 1800000 | Urogenital |
Rickettsia rickettsii | Rocky Mountain Spotted Fever | Tick bites | 5 | Vector-borne |
Mycobacterium tuberculosis | Tuberculosis | Airborne | 8 | Respiratory |
Chlamydia pneumoniae | Pneumonia | Airborne droplets | 250000 | Respiratory |
Common Intracellular Bacterial Pathogens
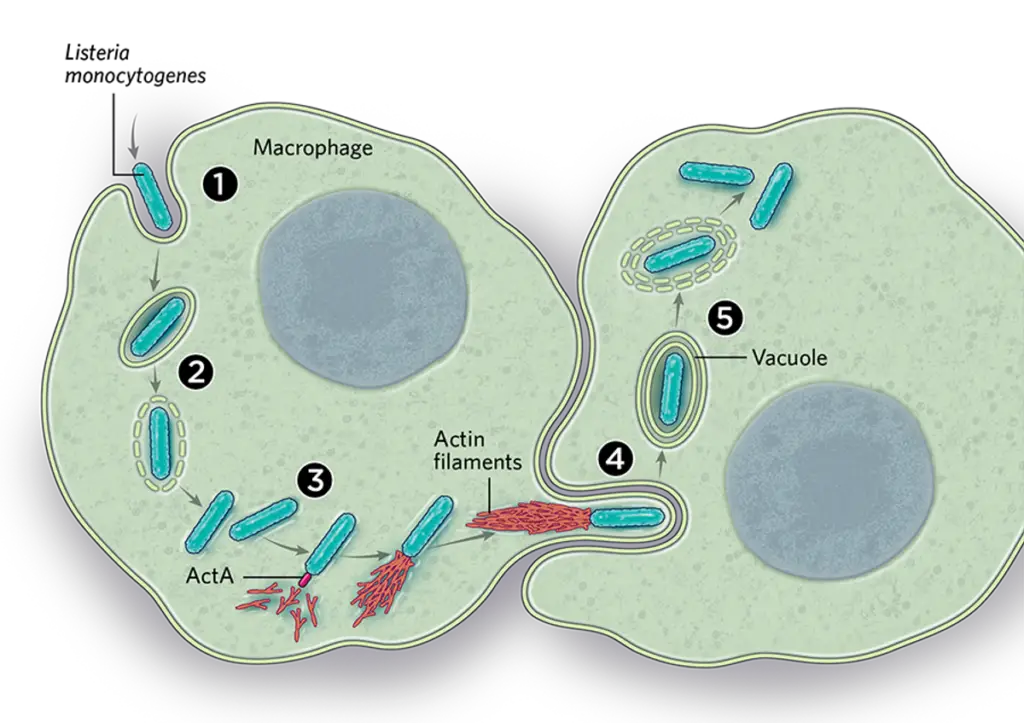
Image : Pathogen interaction with macrophages in cellular processes (The image illustrates the cellular process involving a macrophage interacting with pathogens. It depicts the stages of pathogen uptake and manipulation within the macrophage as represented by numbered steps. Step 1 shows the pathogen (cyan) entering the macrophage. Step 2 indicates the formation of a vacuole around the pathogen. Step 3 portrays the recruitment of ActA protein and actin filaments (depicted in red) to aid in pathogen movement within the cell. Step 4 illustrates the transportation of the pathogen along the actin filaments. Step 5 emphasizes the vacuole containing the pathogen as part of its lifecycle. This detailed visual representation aids in understanding the interactions between immune cells and pathogens, which is crucial for studies in immunology and cell biology.)
A. Chlamydia: A Silent but Widespread Infection
Intracellular bacterial pathogens are interesting and complicated to study because they can invade host cells and control various cell processes to survive and grow. Examples of such pathogens include Chlamydia trachomatis, Rickettsia spp., and Mycobacterium tuberculosis, each with unique and complex relationships with host cells. Chlamydia particularly uses the inside of the host cell to avoid the immune response while it replicates in a specific compartment called an inclusion. This ability helps it survive and gather necessary resources for growth and division. On the other hand, Rickettsia uses actin polymerization to move, allowing it to spread from one host cell to nearby cells. This impressive process has been shown in many studies that describe its complex invasion and movement methods. Additionally, Mycobacterium tuberculosis survives in macrophages by altering the host’s signaling pathways to block programmed cell death, or apoptosis, while using various strategies that may change the host cell’s metabolism. Understanding these complex methods is important for creating focused treatments and effective public health plans to fight the diseases caused by these intracellular pathogens. Moreover, recognizing how infections like Chlamydia can be silent is essential since many may not show symptoms while still spreading the infection, complicating control measures. A thorough look into the exact strategies these bacteria use highlights the significance of host-pathogen relationships in infectious diseases, providing valuable knowledge for researchers and healthcare workers in addressing these public health challenges. By improving our understanding of these interactions, we can more effectively manage the issues these infections pose in communities and enhance prevention strategies on a broader scale.
Table : Key Aspects of Chlamydia Infection
Category | Details |
---|---|
Causative Agent | Chlamydia trachomatis (an obligate intracellular bacterium) |
Transmission | Primarily through sexual contact (vaginal, anal, or oral sex); can also be passed from mother to child during childbirth |
Symptoms | Often asymptomatic; when present, symptoms may include abnormal genital discharge, burning sensation during urination, pelvic pain, or testicular discomfort |
High-Risk Groups | Sexually active individuals, particularly those with multiple partners; adolescents and young adults |
Complications | If untreated, can lead to pelvic inflammatory disease (PID), ectopic pregnancy, infertility, epididymitis in men, and increased risk of other STIs |
Diagnosis | Nucleic acid amplification tests (NAATs) via urine samples or swabs from the cervix, urethra, rectum, or throat |
Treatment | Antibiotics (typically azithromycin or doxycycline) |
Prevention | Consistent use of condoms, regular STI screening, mutual monogamy, and prompt treatment of infected individuals |
Global Impact | One of the most reported bacterial STIs worldwide, with millions of new cases annually |
Table : Major Chlamydia Diseases and Their Characteristics
Disease | Causative Species | Mode of Transmission | Primary Symptoms | Complications | At-Risk Groups |
---|---|---|---|---|---|
Chlamydia (STI) | Chlamydia trachomatis (D–K serotypes) | Sexual contact (vaginal, anal, oral) | Often asymptomatic; genital discharge, burning urination, pelvic pain | Pelvic inflammatory disease (PID), infertility, ectopic pregnancy, epididymitis | Sexually active individuals, especially young adults |
Trachoma | Chlamydia trachomatis (A, B, C serotypes) | Direct contact, contaminated hands, flies | Eye irritation, conjunctivitis, eyelid scarring | Corneal damage, blindness | People in poor sanitation areas (Africa, Asia, Middle East) |
Chlamydia pneumonia | Chlamydia pneumoniae | Airborne respiratory droplets | Mild cough, sore throat, pneumonia-like symptoms | Chronic lung disease, asthma exacerbation | Elderly, immunocompromised individuals |
Psittacosis (Parrot Fever) | Chlamydia psittaci | Inhalation of bird droppings/dust | Fever, chills, headache, severe pneumonia | Respiratory failure, systemic infection | Bird owners, pet shop workers, poultry farmers |
Neonatal Conjunctivitis & Pneumonia | Chlamydia trachomatis (D–K serotypes) | Vertical transmission (mother to baby during birth) | Eye redness, swelling, discharge, respiratory distress (in pneumonia cases) | Blindness (rare), chronic lung problems | Newborns of infected mothers |
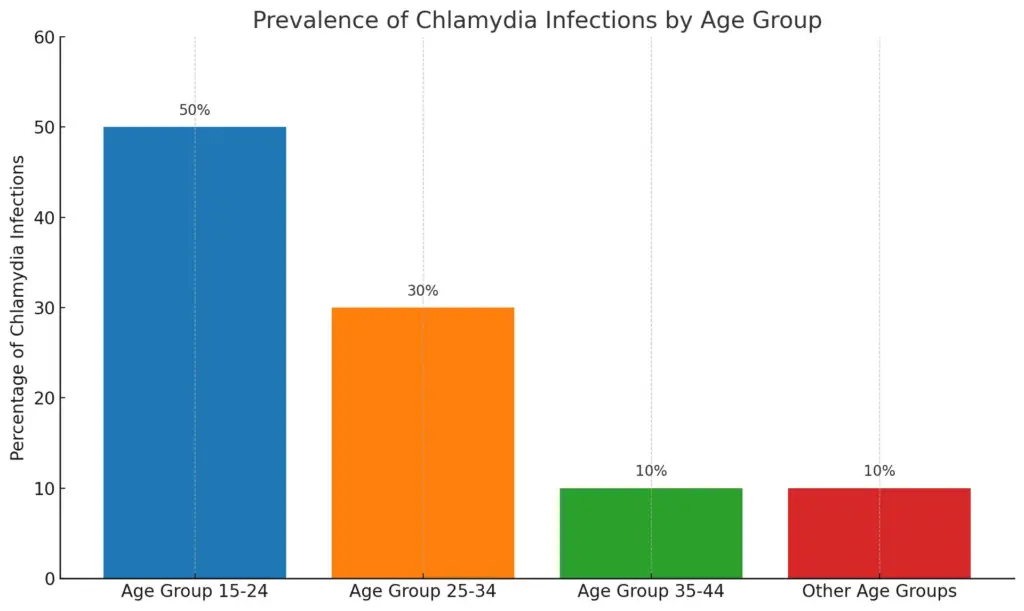
This bar chart illustrates the prevalence of Chlamydia infections across different age groups. It highlights that 50% of infections occur in the age group of 15-24 years, while 30% are found in the age group of 25-34 years. The age groups of 35-44 and other age groups each account for 10% of infections. This data underscores the challenge posed by the asymptomatic nature of the infection, particularly among younger populations, and reflects the classification of Chlamydia as a silent yet widespread infection.
B. Rickettsia: The Cause of Typhus and Rocky Mountain Spotted Fever
Rickettsia is a type of bacteria that live inside cells. They cause diseases like typhus and Rocky Mountain spotted fever (RMSF), which are important health issues and can cause serious problems if not diagnosed and treated quickly. These bacteria usually use lice and ticks as vectors, which help them get into human blood and infect the cells lining blood vessels. After entering the host, Rickettsia take over host cell processes to grow and spread, often causing strong inflammatory responses that can harm health. In RMSF cases, this results in a rash that may start as small spots and can grow into big skin lesions, along with dysfunction in multiple organs, leading to many troubling symptoms for patients and medical professionals. The way Rickettsia cause disease shows how they evade the immune system, helping them continue their lifecycle while causing harm. Many studies depict these complex interactions and strategies, helping us understand how Rickettsia affect the host and create symptoms. Managing Rickettsial diseases requires a teamwork approach with public health monitoring, control strategies for vectors, and education efforts to inform people about prevention and prompt treatment for those infected.
Table : Key Aspects of Rickettsia Infections
Category | Details |
---|---|
Causative Agent | Rickettsia species, including R. prowazekii (typhus) and R. rickettsii (RMSF) |
Mode of Transmission | Arthropod vectors: lice (typhus), ticks (RMSF), fleas (murine typhus) |
Target Cells | Endothelial cells lining blood vessels |
Major Diseases | Epidemic typhus, endemic typhus, Rocky Mountain spotted fever |
Symptoms | Fever, rash, headache, muscle pain, vascular damage, organ failure (in severe cases) |
Diagnosis | Blood tests (PCR, serology), clinical symptoms, history of insect bites |
Treatment | Doxycycline (effective if given early) |
Complications | Severe cases can lead to multi-organ failure, shock, or death if untreated |
Prevention | Avoiding tick and flea bites, using insect repellents, improving sanitation |
Geographical Distribution | Worldwide, with endemic areas in the U.S., South America, Africa, and parts of Asia |
Major Rickettsia Diseases and Their Characteristics
Disease | Causative Bacteria | Vector | Key Symptoms | Risk Factors |
---|---|---|---|---|
Epidemic Typhus | Rickettsia prowazekii | Body lice | High fever, severe headache, rash, delirium | Crowded, unsanitary conditions (e.g., refugee camps, prisons) |
Endemic (Murine) Typhus | Rickettsia typhi | Fleas | Mild fever, rash, muscle pain | Exposure to flea-infested rodents |
Rocky Mountain Spotted Fever | Rickettsia rickettsii | Ticks | High fever, spotted rash, vascular damage, organ failure | Tick exposure in rural areas, outdoor activities |
C. Mycobacteria: Tuberculosis and Leprosy
Mycobacteria, mainly Mycobacterium tuberculosis and Mycobacterium leprae, are important for studying intracellular bacterial infections and how they interact with the host’s immune system. Tuberculosis (TB), which is caused by M. tuberculosis, is a major health issue worldwide. It can create latent infections that might last for many years before becoming active again, possibly causing serious respiratory problems and leading to high rates of sickness and death globally. On the other hand, leprosy, also called Hansen’s disease, caused by M. leprae, mostly impacts peripheral nerves and results in serious issues like loss of sensation, muscle weakness, and disfigurement, affecting those who suffer from it. Both species of mycobacteria have complex ways to survive inside host cells, avoiding immune responses by altering macrophage function and creating a special environment for their growth. This relationship between mycobacteria and the host is vital for creating targeted treatments and vaccine strategies, especially with the rise of drug-resistant TB strains that challenge public health. The way these pathogens interact with host cells highlights the urgent need for advanced research, as shown in recent scientific studies, which detail important mechanisms affecting how microbes survive and manipulate hosts. By fully grasping these interactions, researchers can develop new treatments and prevention strategies, stressing the need for a united effort to tackle these tough infectious diseases that still pose a risk to global health.
Table : Comparison of Tuberculosis and Leprosy
Feature | Tuberculosis (TB) | Leprosy (Hansen’s Disease) |
---|---|---|
Causative Agent | Mycobacterium tuberculosis | Mycobacterium leprae |
Mode of Transmission | Airborne droplets from infected individuals | Prolonged skin-to-skin contact or respiratory secretions |
Primary Affected Areas | Lungs (but can spread to other organs) | Skin, peripheral nerves, mucous membranes |
Incubation Period | Weeks to months | Several years (up to 20 years) |
Symptoms | Chronic cough, weight loss, night sweats, fever, coughing blood | Skin lesions, numbness, muscle weakness, deformities |
Complications | Lung fibrosis, meningitis, multi-organ failure | Nerve damage, limb deformities, blindness |
Diagnosis | Tuberculin skin test (Mantoux), chest X-ray, sputum culture, PCR test | Skin biopsy, slit-skin smear, PCR test |
Treatment | Combination of antibiotics (isoniazid, rifampin, ethambutol, pyrazinamide) for 6–9 months | Multi-drug therapy (MDT) using dapsone, rifampin, and clofazimine for 6–24 months |
Prevention | BCG vaccine, early diagnosis, public health interventions | Early detection, treatment, contact tracing |
Global Burden | 10 million new cases per year, leading infectious disease killer worldwide | ~200,000 new cases annually, mostly in tropical regions |
Table : Major Mycobacterium Diseases and Their Characteristics
Disease | Causative Bacteria | Mode of Transmission | Primary Symptoms | Complications | At-Risk Groups |
---|---|---|---|---|---|
Tuberculosis (TB) | Mycobacterium tuberculosis | Airborne droplets (coughing, sneezing) | Persistent cough, fever, night sweats, weight loss | Lung damage, meningitis, multi-organ failure | Immunocompromised individuals, malnourished people, those in crowded conditions |
Leprosy (Hansen’s Disease) | Mycobacterium leprae | Prolonged close contact, respiratory droplets | Skin lesions, numbness, muscle weakness | Permanent nerve damage, disfigurement, disability | People in endemic regions, those with prolonged exposure to untreated patients |
III. How Intracellular Bacteria Evade the Immune System
Intracellular bacteria have gotten very complex ways to avoid the host immune system, which helps them live and grow inside host cells for long times. For example, Chlamydia species are good at changing host cell processes by changing the expression of immune-related genes, which tricks the host’s defenses. This ability lets them create a setting that helps them survive and multiply. Also, these bacteria are good at making a safe space inside the host cell, often using host-made vesicles to stay hidden from immune cells that would usually attack and destroy them. Similarly, Mycobacterium tuberculosis has several strategies to stop the fusion of phagosomes and lysosomes, a key step that usually leads to the bacteria’s breakdown. By stopping this fusion, the bacteria create a safe place that helps them live long-term inside the host. The image showing different intracellular bacteria around a host cell shows how these germs use various cellular pathways to stay hidden, reducing the immune system’s ability to respond. This complex relationship between intracellular bacteria and the immune system not only shows the tricky methods used by these pathogens but also points out the major difficulties in creating effective treatments and vaccines against these hard-to-detect bacteria. Knowing how these mechanisms work is vital for improving our understanding and treatment options for infections caused by these tough pathogens.
Table: How Intracellular Bacteria Evade the Immune System (Intracellular bacteria have evolved sophisticated mechanisms to evade the immune system, allowing them to survive, replicate, and persist within host cells. Below is a step-by-step process outlining how these bacteria bypass immune defenses.)
Step | Mechanism of Immune Evasion | Examples of Bacteria | Impact on Host |
---|---|---|---|
1. Host Cell Entry | Bacteria use surface adhesins or secretion systems to invade host cells, often targeting macrophages or epithelial cells. | Salmonella, Listeria monocytogenes, Chlamydia | Avoids extracellular immune defenses like antibodies and complement proteins. |
2. Inhibition of Phagosome-Lysosome Fusion | Bacteria prevent the merging of the phagosome with lysosomes, avoiding degradation. | Mycobacterium tuberculosis, Legionella pneumophila | Allows bacteria to survive inside immune cells instead of being destroyed. |
3. Escape from Phagosomes into Cytoplasm | Some bacteria break open the phagosome and enter the cytoplasm, avoiding lysosomal degradation. | Listeria monocytogenes, Shigella | Avoids digestion by host enzymes and facilitates intracellular movement. |
4. Modification of the Phagosome | Bacteria modify the vacuole membrane to create a protective niche for survival and replication. | Coxiella burnetii, Brucella | Establishes a long-term intracellular habitat, avoiding detection. |
5. Resistance to Oxidative Stress | Bacteria produce enzymes like superoxide dismutase (SOD) and catalase to neutralize reactive oxygen species (ROS). | Salmonella, Mycobacterium tuberculosis | Prevents oxidative killing by immune cells. |
6. Subversion of Host Signaling Pathways | Bacteria interfere with immune signaling, reducing the production of inflammatory cytokines. | Helicobacter pylori, Francisella tularensis | Weakens immune response, allowing persistent infection. |
7. Antigenic Variation | Bacteria alter their surface proteins to evade antibody recognition. | Chlamydia trachomatis, Neisseria gonorrhoeae | Prevents immune memory and allows reinfection. |
8. Suppression of Host Apoptosis | Bacteria prevent programmed cell death to maintain a stable intracellular environment. | Rickettsia, Mycobacterium tuberculosis | Prolongs bacterial survival inside host cells. |
9. Formation of Biofilms or Granulomas | Some intracellular bacteria trigger host cells to form granulomas or biofilms for protection. | Mycobacterium tuberculosis, Brucella | Creates a protective barrier against immune attacks and antibiotics. |
10. Persistent Infection & Latency | Some bacteria enter a dormant state, avoiding immune detection for years. | Mycobacterium tuberculosis, Chlamydia pneumoniae | Leads to chronic infections and reactivation under weakened immunity. |
A. Hiding Inside Host Cells to Avoid Detection
Intracellular bacteria have developed clever ways to hide from the immune systems of their hosts, making them important for studying infectious diseases. By living inside host cells like macrophages, these germs take advantage of the cell’s resources for necessary nutrients while staying out of reach of immune detection, which improves their chances of surviving. This specific living space gives them a protective environment where they can grow and multiply without being immediately attacked by the immune system. For instance, Chlamydia species can affect the signaling pathways in the host cells, creating a better environment for their growth. This manipulation alters the host’s immune response, prolonging their existence and helping them spread infections to nearby cells, which keeps their lifecycle going. Additionally, many bacterial pathogens use various methods, like changes in gene expression and host factor modifications, to survive in these hidden intracellular spaces. These adaptive tactics make them particularly hard to eliminate, posing major challenges for the host’s defense systems. To effectively fight against intracellular infections, it is essential to understand these complicated interactions, as it helps researchers find weaknesses in how microbes behave. Therefore, examining these tactics further highlights the significant difficulties that modern medicine faces in dealing with bacteria that can hide, emphasizing the urgent need for new ways to combat these tricky germs and reduce their effects on public health.
Table: Hiding Inside Host Cells to Avoid Detection (Intracellular bacteria have evolved various mechanisms to hide within host cells, effectively avoiding immune detection and destruction. This enables them to persist in the body, sometimes leading to chronic infections. Below is a detailed step-by-step breakdown of how these bacteria achieve this.)
Step | Mechanism of Hiding | Examples of Bacteria | Effect on Host |
---|---|---|---|
1. Entry into Host Cells | Bacteria use adhesins and secretion systems to invade non-immune and immune cells (e.g., macrophages, epithelial cells). | Listeria monocytogenes, Salmonella, Chlamydia | Allows bacteria to escape immune surveillance in extracellular spaces. |
2. Prevention of Phagosome-Lysosome Fusion | Bacteria block the fusion of the phagosome (where they are trapped) with lysosomes, preventing destruction by digestive enzymes. | Mycobacterium tuberculosis, Legionella pneumophila | Enables long-term survival inside immune cells. |
3. Escape into the Cytoplasm | Bacteria break out of the phagosome and move freely inside the cytoplasm to avoid lysosomal degradation. | Listeria monocytogenes, Shigella | Avoids immune detection and allows intracellular movement. |
4. Modifying the Vacuole for Protection | Bacteria create a modified vacuole that resists immune attacks and provides a safe environment for replication. | Coxiella burnetii, Brucella, Chlamydia | Prevents recognition and destruction by immune cells. |
5. Inhibiting Apoptosis (Cell Death) | Some bacteria produce proteins that stop infected host cells from self-destructing, ensuring a stable home. | Mycobacterium tuberculosis, Rickettsia | Prolongs bacterial survival inside host cells. |
6. Manipulating Host Immune Signaling | Bacteria interfere with immune signaling pathways, reducing inflammatory responses and immune activation. | Francisella tularensis, Helicobacter pylori | Suppresses the immune response, allowing bacterial persistence. |
7. Antigenic Variation | Bacteria change their surface proteins over time to prevent recognition by antibodies. | Chlamydia trachomatis, Neisseria gonorrhoeae | Prevents immune memory formation and reinfection detection. |
8. Formation of Granulomas | Some bacteria trigger host cells to form protective granulomas, shielding them from immune attack. | Mycobacterium tuberculosis, Brucella | Creates a chronic infection site, making treatment difficult. |
9. Entering a Dormant (Latent) State | Bacteria slow down metabolism and become dormant, avoiding immune detection and antibiotic effects. | Mycobacterium tuberculosis, Chlamydia pneumoniae | Leads to chronic infections that can reactivate later. |
10. Using Host Actin for Movement | Some bacteria hijack host actin filaments to move between cells, avoiding extracellular immune defenses. | Listeria monocytogenes, Shigella | Spreads infection without exposure to antibodies. |
B. How These Bacteria Manipulate Host Cellular Processes
Intracellular bacteria like Chlamydia, Rickettsia, and Mycobacteria have developed complex ways to manage host cellular processes, helping them survive and reproduce inside the host. By taking over the host’s signaling pathways, these pathogens make environments that support their growth while avoiding the host’s immune responses. For example, Chlamydia trachomatis changes the host’s cytoskeleton through special proteins to aid its own movement and replication, making sure it can stay in the host. Rickettsia is known for its strong movement and uses the host cell’s actin polymerization system to spread from one cell to another, effectively using the host’s resources to grow and survive. Mycobacteria can affect how phagosomes mature in immune cells, allowing them to avoid being destroyed while thriving in a tough environment. Diagrams or illustrations can help show the complex relationships between these bacteria and host cells, emphasizing the important biological processes in this manipulation. This detailed interaction of molecular processes shows how these pathogens have evolved and adjusted over time, emphasizing their advanced strategies. Given these strategies, it is clear that more research is needed to learn about their methods and understand how they affect host cell functions. Creating focused treatment strategies will be vital in fighting these infections and reducing their effects on human health.
Table: How Intracellular Bacteria Manipulate Host Cellular Processes (Intracellular bacteria have evolved sophisticated mechanisms to manipulate host cell processes, ensuring their survival, replication, and evasion of the immune system. These bacteria hijack cellular pathways to avoid destruction and persist in the host. Below is a step-by-step breakdown of these strategies.)
Step | Mechanism of Manipulation | Examples of Bacteria | Effect on Host |
---|---|---|---|
1. Host Cell Invasion | Bacteria use adhesins, invasins, or secretion systems to enter host cells, often targeting epithelial or immune cells. | Listeria monocytogenes, Salmonella, Chlamydia | Allows bacteria to escape extracellular immune defenses. |
2. Altering Host Cytoskeleton | Bacteria hijack actin filaments to promote entry, intracellular movement, or cell-to-cell spread. | Listeria monocytogenes, Shigella | Enables bacteria to move within cells and avoid immune detection. |
3. Blocking Phagosome-Lysosome Fusion | Bacteria prevent their own destruction by stopping lysosomal fusion with the phagosome. | Mycobacterium tuberculosis, Legionella pneumophila | Allows bacteria to survive inside immune cells. |
4. Escaping the Phagosome | Some bacteria produce toxins or enzymes to rupture the phagosome and enter the cytoplasm. | Listeria monocytogenes, Shigella | Avoids digestion by host cell enzymes. |
5. Reprogramming Host Gene Expression | Bacteria interfere with host DNA transcription to suppress immune responses. | Helicobacter pylori, Chlamydia trachomatis | Weakens immune response, promoting bacterial persistence. |
6. Suppressing Apoptosis (Cell Death) | Some bacteria prevent host cells from dying to maintain their intracellular niche. | Mycobacterium tuberculosis, Rickettsia | Prolongs bacterial survival inside infected cells. |
7. Modifying Host Signaling Pathways | Bacteria interfere with cytokine and immune signaling, reducing inflammation and immune activation. | Francisella tularensis, Brucella | Weakens the immune response and allows bacterial survival. |
8. Creating Specialized Vacuoles | Some bacteria create protective compartments inside the host cell for replication. | Coxiella burnetii, Chlamydia | Provides a safe environment for bacterial growth. |
9. Triggering Host Cell Autophagy for Their Benefit | Bacteria manipulate autophagy to create nutrient-rich environments. | Salmonella, Legionella | Enhances bacterial survival and replication. |
10. Inducing Host Cell Lysis for Spread | Some bacteria cause host cell rupture to exit and infect new cells. | Rickettsia, Listeria monocytogenes | Spreads infection while avoiding immune defenses. |
IV. Treatment and Challenges of Intracellular Bacterial Infections
Treating infections caused by bacteria that live inside cells is very complex. This is because different bacteria, like Chlamydia, Rickettsia, and Mycobacteria, have distinct life cycles and methods of avoiding the immune system. These bacteria grow inside host cells, which protects them from common antibiotics that work on bacteria outside cells. Therefore, we need drugs that can specifically hit functions inside the cells that the bacteria need to survive and multiply. For example, macrolides and tetracyclines are effective against Chlamydia because they stop protein production. For Rickettsial infections, fluoroquinolones and other specialized drugs are needed to meet the unique metabolic requirements of these bacteria. Nevertheless, treating Mycobacteria, especially in diseases like tuberculosis, is challenging. These bacteria can enter a dormant state, requiring long treatment and combinations of several drugs to fully clear the infection from the body. Furthermore, the rise of antibiotic resistance makes it even harder to treat these infections, as it reduces the effectiveness of current antibiotics and highlights the urgent need for new drugs to address resistant strains. To successfully tackle these issues, we must understand how these pathogens behave inside cells. By identifying and targeting the specific pathways used by intracellular bacteria, we can develop better treatment options that bypass their defense mechanisms. Research is ongoing in this area, which could greatly improve patient outcomes in the future, including looking into new types of drugs and therapies that can enhance the immune response. Addressing intracellular infections in a systematic and innovative way is an essential focus of infectious disease research. As we learn more, we may discover better methods to fight these tough infections and enhance global health outcomes.
Table: Treatment and Challenges of Intracellular Bacterial Infections (Intracellular bacterial infections are difficult to treat due to the bacteria’s ability to hide within host cells, evade immune responses, and resist conventional antibiotics. Below is a detailed breakdown of treatment options and the challenges associated with these infections.)
Aspect | Details | Examples of Bacteria | Impact on Treatment |
---|---|---|---|
1. Antibiotic Selection | Requires antibiotics that can penetrate host cells and target intracellular bacteria. | Mycobacterium tuberculosis, Chlamydia trachomatis, Legionella pneumophila | Standard antibiotics like β-lactams are ineffective because they do not reach intracellular compartments. |
2. Use of Lipophilic Antibiotics | Lipophilic antibiotics (e.g., macrolides, fluoroquinolones, tetracyclines) are preferred as they can cross cell membranes. | Chlamydia, Rickettsia, Brucella | Ensures effective intracellular penetration and bacterial eradication. |
3. Long Treatment Duration | Many intracellular bacteria grow slowly, requiring prolonged antibiotic regimens. | Mycobacterium tuberculosis, Brucella | Risk of antibiotic resistance, poor patient compliance, and relapse. |
4. Immune System Modulation | Some treatments include immunomodulators (e.g., corticosteroids) to control excessive immune responses. | Legionella, Mycobacterium tuberculosis | Reduces host tissue damage but may suppress immune clearance of bacteria. |
5. Vaccine Development | Vaccines can prevent some intracellular infections but are challenging to develop. | Mycobacterium tuberculosis (BCG vaccine) | Not always effective in adults, requires booster doses or novel strategies. |
6. Antibiotic Resistance | Intracellular bacteria often develop multidrug resistance (MDR), complicating treatment. | Mycobacterium tuberculosis (MDR-TB), Chlamydia | Requires combination therapy and development of new antibiotics. |
7. Limited Drug Penetration | Some bacteria hide in granulomas or specialized vacuoles, making drug delivery difficult. | Mycobacterium tuberculosis, Brucella | Leads to persistence of infection and potential relapse. |
8. Latency and Reactivation | Some bacteria enter a dormant state, reactivating when the immune system is weakened. | Mycobacterium tuberculosis, Chlamydia pneumoniae | Long-term monitoring and preventive therapy are needed. |
9. Co-Infections and Host Factors | Immunocompromised patients (e.g., HIV-positive individuals) are more susceptible to intracellular infections. | Mycobacterium tuberculosis (TB-HIV co-infection) | Requires integrated treatment approaches for both infections. |
10. Emerging Therapies | Research focuses on host-directed therapies, phage therapy, and nanoparticle-based drug delivery. | Mycobacterium tuberculosis, Salmonella | Potential breakthroughs but still under investigation. |
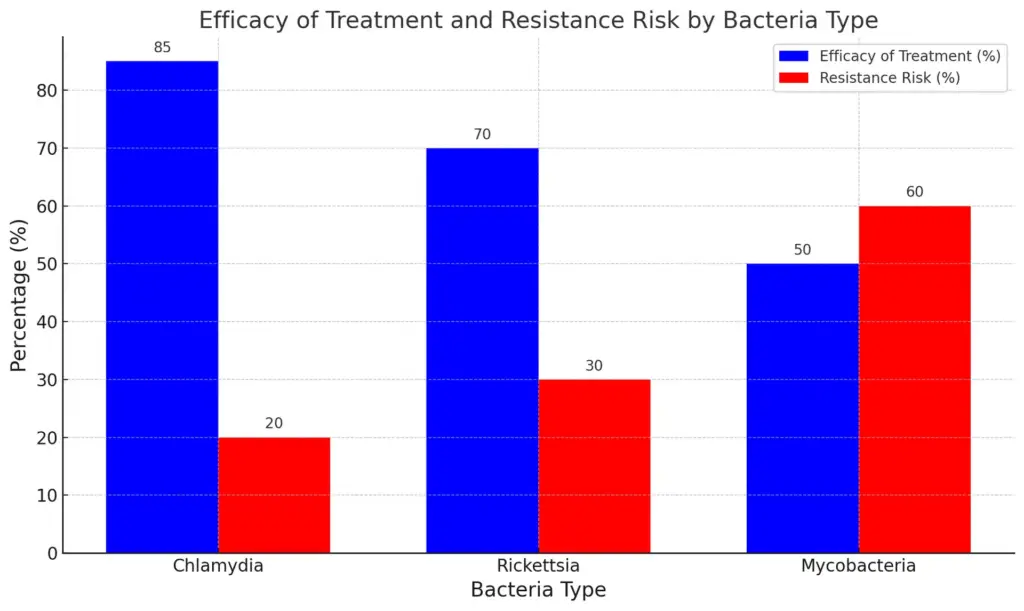
This bar chart compares the efficacy of treatments and resistance risks associated with three types of intracellular bacteria: Chlamydia, Rickettsia, and Mycobacteria. It effectively illustrates that while Chlamydia has a high treatment efficacy rate of 85%, it also faces a significant resistance challenge of 20%. In contrast, Mycobacteria, notably in tuberculosis, shows a lower treatment efficacy of 50% along with a higher resistance risk of 60%, emphasizing the complexity of managing these infections.
A. Why Intracellular Bacteria Are Harder to Kill with Antibiotics
Intracellular bacteria, like Chlamydia, Rickettsia, and Mycobacteria, create major issues for treating infections mainly because they can avoid antibiotics effectively. These germs have the skill to change their host’s cellular settings, making special areas where they can live and grow without being found by the immune system. By living inside host cells, they can stay away from the circulation of antimicrobial drugs, which mainly focus on germs that are outside the cells. This internal presence is key for their survival as it shields them from antibiotics, which usually don’t work well against bacteria in cells. For example, these bacteria can use the host’s cellular processes to grow while changing important functions of the host, making them less vulnerable to medicines. The ways they alter host cell functions also have major effects on the immune system, allowing a suitable environment for their growth while hindering normal immune responses. This situation complicates the way infections are managed clinically and brings about chronic infections that are tough to treat with standard antibiotics. Studies show that the interactions between these bacteria and host cells reveal complex survival mechanisms and increase their harmfulness. This ability extends the infection duration and complicates creating effective treatment plans, calling for a more detailed and varied therapy approach. Additionally, the growth of antibiotic resistance in these intracellular bacteria adds extra challenges as they become able to avoid many types of once-effective antibiotics. Therefore, it is crucial to better understand how these bacteria cause disease in order to develop targeted treatments capable of entering host cells and effectively combating these tough pathogens, which would help improve outcomes for patients and lessen the impact of these challenging infections.
10 Reasons Why Intracellular Bacteria Are Harder to Kill with Antibiotics (with Examples):
- They Hide Inside Host Cells – Intracellular bacteria evade antibiotics by residing inside human cells, where many drugs cannot reach them. Example: Chlamydia trachomatis remains inside epithelial cells, avoiding antibiotic exposure.
- Many Antibiotics Can’t Enter Host Cells – Large or hydrophilic antibiotics, such as penicillins, struggle to cross host cell membranes, limiting their effectiveness. Example: Mycobacterium tuberculosis resists β-lactam antibiotics due to poor penetration into macrophages.
- They Create Protective Vacuoles – Some bacteria form specialized compartments inside host cells, preventing antibiotics from reaching them. Example: Legionella pneumophila survives inside alveolar macrophages by forming the Legionella-containing vacuole (LCV).
- They Grow Slowly or Enter Dormancy – Many intracellular bacteria, like Mycobacterium tuberculosis, reduce their metabolic activity, making them less susceptible to antibiotics that target dividing cells. Example: Mycobacterium leprae enters a dormant state, prolonging infection and making treatment difficult.
- They Form Biofilms or Granulomas – Certain bacteria trigger the formation of biofilms or granulomas, which act as physical barriers that shield them from antibiotics. Example: Brucella spp. forms granulomas in organs like the liver and spleen, limiting antibiotic effectiveness.
- They Use Efflux Pumps to Expel Antibiotics – Some intracellular bacteria have efflux pumps that actively remove antibiotics from their cells, reducing drug effectiveness. Example: Salmonella enterica uses efflux pumps like AcrAB-TolC to remove antibiotics.
- They Modify Their Antibiotic Target Sites – Some bacteria alter their ribosomes or enzymes, preventing antibiotics from binding and disrupting their function. Example: Rickettsia spp. modifies ribosomes to resist tetracycline antibiotics.
- They Suppress the Host Immune System – Intracellular bacteria, like Francisella tularensis, manipulate immune responses, reducing inflammation and allowing them to persist longer. Example: Francisella tularensis inhibits phagosome maturation, evading immune detection.
- They Lack Effective Vaccines – Many intracellular bacteria do not have widely available vaccines, making treatment with antibiotics the only option, which increases the risk of resistance. Example: There is no widely available vaccine for Chlamydia trachomatis, leading to high infection rates.
- They Mutate and Adapt Quickly – Intracellular bacteria can rapidly develop resistance to antibiotics, requiring constant development of new drugs and treatment strategies. Example: Mycobacterium tuberculosis rapidly develops resistance to isoniazid and rifampin, requiring combination therapy.
B. Potential New Therapies Targeting Intracellular Pathogens
The search for new treatment methods aimed at intracellular pathogens has become more important, especially for diseases linked to Chlamydia, Rickettsia, and Mycobacteria. These pathogens have developed advanced ways to avoid the host’s immune responses, making regular antibiotics less effective and often resulting in long-lasting infections that are hard to manage. New studies have suggested various innovative methods, such as using effector protein blockers and therapies that target the host to disrupt the intracellular environment that benefits the pathogens. By identifying and understanding the specific pathways and mechanisms these bacteria use to manipulate host cellular functions, researchers can create treatments that directly counter these interactions, leading to better treatment options. For example, new small molecules that stop the formation of bacterial effector proteins could hinder the pathogens from taking control of critical cellular functions they need to survive and reproduce. Additionally, using nanotechnology and targeted drug delivery systems could improve how effectively therapeutic agents reach the sites of infection inside cells, allowing for more efficient and precise treatment. These developments could help enhance treatment effectiveness, especially in cases resistant to traditional antibiotics. Moreover, looking into combination therapies that use several mechanisms together might further improve treatment results. These advancements highlight the necessity of a comprehensive approach to address these complex infections, as seen in recent studies that outline the detailed pathways these bacteria use within host cells and suggest potential targets for treatment. By advancing in these areas, there is increasing hope for significantly enhancing patient outcomes in battling intracellular pathogens.
20 Potential New Therapies Targeting Intracellular Pathogens (with Examples):
- Host-Directed Therapies (HDTs) – Strengthening the host’s immune system to fight infections rather than directly targeting bacteria. Example: IFN-γ therapy to enhance immune response against tuberculosis.
- Nanoparticle-Based Drug Delivery – Using nanoparticles to improve antibiotic penetration into infected cells. Example: Liposomal amikacin for treating Mycobacterium avium infections.
- Phage Therapy – Using bacteriophages (viruses that infect bacteria) to eliminate intracellular pathogens. Example: Phage therapy for Mycobacterium abscessus in cystic fibrosis patients.
- Autophagy-Boosting Drugs – Enhancing the body’s natural autophagy process to remove intracellular bacteria. Example: Rapamycin to stimulate autophagy against Salmonella infections.
- CRISPR-Based Antibacterial Strategies – Using gene-editing tools to target and destroy bacterial DNA. Example: CRISPR-Cas9 to eliminate antibiotic-resistant Mycobacterium tuberculosis strains.
- Immunotherapy Approaches – Using monoclonal antibodies or immune-modulating drugs to enhance pathogen clearance. Example: Anti-TNF therapy in combination with antibiotics for tuberculosis.
- Small Molecule Inhibitors – Developing drugs that block bacterial survival mechanisms inside host cells. Example: Bortezomib to inhibit protein degradation pathways in Legionella pneumophila infections.
- Host Cell Metabolism Modulation – Altering metabolism to deprive bacteria of essential nutrients. Example: Metformin to reduce glucose availability for Mycobacterium tuberculosis.
- Targeting Bacterial Secretion Systems – Blocking bacterial secretion systems that manipulate host cells. Example: T3SS inhibitors for Chlamydia trachomatis infections.
- Combination Therapy with Existing Antibiotics – Using multiple antibiotics with different mechanisms to improve treatment.
Example: Rifampin and doxycycline for intracellular Brucella infections. - Peptide-Based Antimicrobials – Short antimicrobial peptides designed to penetrate host cells and kill bacteria. Example: LL-37 peptide against Listeria monocytogenes infections.
- Live-Attenuated Bacterial Vaccines – Using weakened bacteria to train the immune system. Example: BCG vaccine for Mycobacterium tuberculosis.
- Probiotic-Based Treatments – Engineering beneficial bacteria to outcompete harmful intracellular pathogens. Example: Lactobacillus strains preventing Salmonella infections in the gut.
- RNA Interference (RNAi) Therapy – Silencing bacterial genes essential for survival inside host cells. Example: siRNA therapy targeting Francisella tularensis.
- Artificial Intelligence (AI)-Designed Drugs – AI-driven discovery of new intracellular pathogen-targeting drugs. Example: AI-predicted antimicrobial peptide PLG0206 for multi-drug resistant bacteria.
- Liposome-Encapsulated Antibiotics – Using liposomes to deliver antibiotics directly into infected cells. Example: Liposomal ciprofloxacin for treating intracellular Pseudomonas aeruginosa.
- Iron Chelation Therapy – Starving bacteria of essential iron using iron-binding molecules. Example: Deferoxamine to inhibit Brucella growth inside macrophages.
- Mimicking Natural Host Defenses – Designing drugs that replicate the body’s antimicrobial peptides. Example: Cathelicidin-mimicking peptides against Staphylococcus aureus.
- Gene Therapy for Enhanced Immunity – Modifying host genes to improve resistance against intracellular infections. Example: Genetic engineering of macrophages to resist Listeria monocytogenes infections.
- Targeting Quorum Sensing – Disrupting bacterial communication systems to prevent intracellular survival. Example: Quorum-sensing inhibitors against Pseudomonas aeruginosa infections.
V. The Future of Intracellular Bacteria Research
As research continues, the future of studying bacteria inside cells will depend on new tools in molecular biology and genetic engineering that are changing quickly in science today. Learning how pathogens like Chlamydia, Rickettsia, and Mycobacteria change host cell processes will help researchers not only understand these changes but also find new treatment targets and create better therapies to fight these infections. Because these bacteria change easily, new methods like CRISPR-Cas9 gene editing and better imaging technologies will improve our ability to see how bacteria interact with host cells in real-time. This ability will lead to finding new ways these pathogens operate and escape the immune system, thus creating new chances for treatment options. Additionally, combining proteomics with data from high-throughput sequencing will give a clearer picture of the complex relationship between intracellular bacteria and their hosts. This combination is crucial for understanding the changes in metabolism and traits that happen during infection and will eventually help in developing better prevention and treatments. For example, various recent studies show how these pathogens change host cell behavior, paving the way for future advancements in treating infectious diseases and finding new treatments that are both effective and safe. Ongoing investment in research across different subjects and teamwork among scientists will be essential to reach these objectives as we progress in this area.
TABLE – The Future of Intracellular Bacteria Research
Research Area | Description | Potential Impact | Examples |
---|---|---|---|
Host-Directed Therapies (HDTs) | Targeting host pathways to enhance immune response against intracellular pathogens. | Reduces antibiotic resistance and enhances pathogen clearance. | IFN-γ therapy for tuberculosis. |
CRISPR-Based Antibacterials | Using gene-editing tools to disrupt bacterial DNA inside host cells. | Precise elimination of intracellular bacteria without harming host cells. | CRISPR-Cas9 therapy for Mycobacterium tuberculosis. |
Nanotechnology-Driven Drug Delivery | Developing nanoparticles to improve intracellular antibiotic penetration. | Increases drug effectiveness and reduces systemic toxicity. | Liposomal rifampin for tuberculosis treatment. |
Artificial Intelligence (AI) in Drug Discovery | AI-driven screening of new antimicrobial compounds targeting intracellular bacteria. | Faster discovery of novel antibiotics and reduced failure rates. | AI-predicted antimicrobial peptides for Salmonella. |
Bacteriophage Therapy | Engineering phages to target and eliminate intracellular bacteria. | Alternative to traditional antibiotics, especially for resistant strains. | Phage therapy for Mycobacterium abscessus. |
Autophagy-Enhancing Compounds | Stimulating the body’s natural ability to clear intracellular pathogens. | Promotes bacterial degradation inside host cells. | Rapamycin-induced autophagy for Listeria monocytogenes. |
Live-Attenuated Vaccines | Developing genetically modified bacteria to train the immune system. | Prevents infections and reduces antibiotic dependency. | New tuberculosis vaccine candidates beyond BCG. |
Quorum Sensing Inhibitors | Disrupting bacterial communication to limit intracellular survival. | Reduces virulence and infection persistence. | Quorum-sensing blockers for Pseudomonas aeruginosa. |
Combination Antibiotic Therapy | Using synergistic drug combinations to improve intracellular bacterial clearance. | Increases treatment success rates and prevents resistance. | Rifampin + doxycycline for Brucella infections. |
Targeting Bacterial Secretion Systems | Inhibiting bacterial secretion systems that manipulate host cells. | Blocks bacterial survival mechanisms inside host cells. | T3SS inhibitors for Chlamydia trachomatis. |
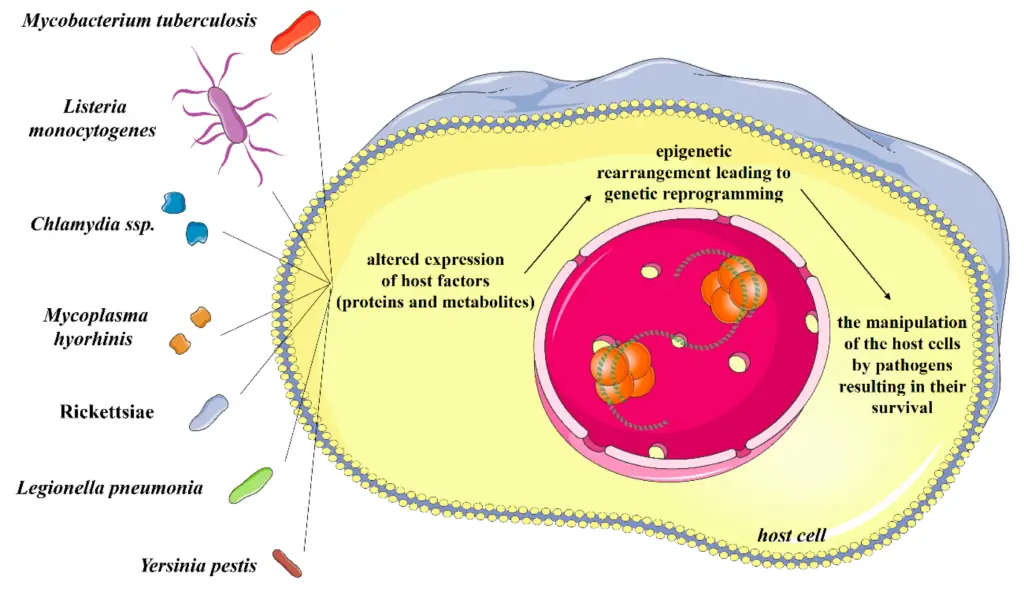
Image : Illustration of Pathogen Manipulation of Host Cell Functions (The image illustrates various bacterial pathogens, including Mycobacterium tuberculosis, Listeria monocytogenes, Chlamydia spp., Mycoplasma hyorhinis, Rickettsiae, Legionella pneumonia, and Yersinia pestis, surrounding a host cell. It highlights the mechanisms by which these pathogens manipulate host cell biological processes. Key concepts include the epigenetic rearrangement leading to genetic reprogramming, altered expression of host factors such as proteins and metabolites, and the overall manipulation of host cells by these pathogens to enhance their survival. This visual representation is valuable for understanding pathogenic mechanisms and interactions with host cells in microbiology and infectious disease studies.)
REFERENCES
- Anol Bhattacherjee. ‘Social Science Research.’ Principles, Methods, and Practices, CreateSpace Independent Publishing Platform, 3/16/2012
- PenZen Summaries. ‘Summary of ALIEN Thinking – [Review Keypoints and Take-aways].’ by Mocktime Publication, 11/29/2022
- Pan American Health Organization. ‘Vaccines.’ Preventing Disease & Protecting Health, Ciro A. de Quadros, Pan American Health Org, 1/1/2004
- World Health Organization. ‘Guidelines for the Prevention Care and Treatment of Persons with Chronic Hepatitis B Virus Infection.’ Mar-15, WHO., World Health Organization, 1/1/2015
- Ludwig Eichinger. ‘Dictyostelium discoideum Protocols.’ Springer Science & Business Media, 2/2/2008
- Larry A. Greenbaum. ‘Practical Strategies in Pediatric Diagnosis and Therapy.’ Robert Kliegman, Elsevier Saunders, 1/1/2004
- Stefano Bertozzi. ‘Disease Control Priorities, Third Edition (Volume 6).’ Major Infectious Diseases, King K. Holmes, World Bank Publications, 11/6/2017
- C. M. Black. ‘Chlamydial Infection: A Clinical and Public Health Perspective.’ Karger Medical and Scientific Publishers, 6/19/2013
- Geert Wiegertjes. ‘Host-Parasite Interactions.’ Gert Flik, Taylor & Francis, 7/1/2004
- Lucile Vaughan Payne. ‘The Lively Art of Writing.’ W. Ross MacDonald School Resource Services Library, 1/1/2006
- Philippe Parola. ‘Rickettsial Diseases.’ Didier Raoult, CRC Press, 4/26/2007
- Alistair McCleery. ‘An Introduction to Book History.’ David Finkelstein, Routledge, 3/13/2006
Image References:
- Image: Pathogen interaction with macrophages in cellular processes., Accessed: 2025.https://cdn.the-scientist.com/assets/articleNo/70729/iImg/48505/12-22-d1-intracellularfeature-1-l.png
- Image: Illustration of Pathogen Manipulation of Host Cell Functions, Accessed: 2025.https://www.mdpi.com/ijms/ijms-21-04573/article_deploy/html/images/ijms-21-04573-g001.png