Leading DNA Strand – Everything You Need To Know
At a replication fork, DNA is formed differently on the two strands.
- One new strand, the leading strand, is made continuously and runs 5′ to 3′ towards the fork.
- The lagging strand, on the other hand, runs 5′ to 3′ away from the fork and is made up of small pieces known as Okazaki fragments.
The leading DNA strand, which is a necessary component of DNA replication, is critical in the process of duplicating genetic information within cells. DNA encodes the instructions required for the development, function, and maintenance of all living organisms as our blueprint for life. Understanding the leading DNA strand is critical to unlocking the mysteries of genetic inheritance as well as the complexities of life itself.
The double-stranded DNA molecule unwinds to expose its two complementary strands during DNA replication, which is a critical process for cell growth, repair, and reproduction. The leading DNA strand serves as a template for the lagging strand’s complementary partner’s continuous synthesis. This intricate dance of replication machinery, which includes enzymes like DNA polymerase, ensures that the entire genome is accurately duplicated.
The ability of the leading DNA strand to provide a continuous template for replication simplifies the process and reduces the risk of errors. Its orderly and continuous nature enables efficient synthesis, ensuring that genetic information is faithfully transmitted to new cells. Understanding how the leading DNA strand functions has far-reaching implications not only for genetics and heredity, but also for biotechnology, medicine, and evolutionary biology.
In this introduction, we will journey into the enthralling world of the leading DNA strand, discovering its mechanisms, significance, and critical role in the perpetuation of life’s diversity.
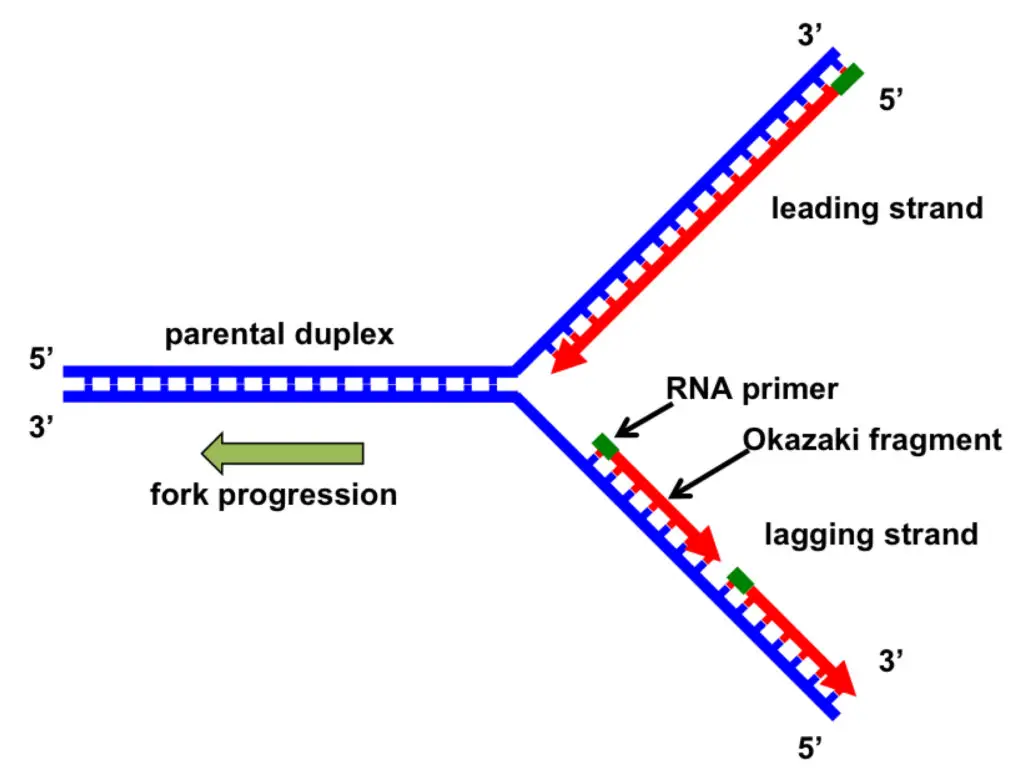
What is the role of the leading strand in DNA replication?
5 Key Roles of the Leading Strand in DNA Replication:
- Continuous Synthesis: During replication, the leading strand acts as a continuous template for DNA synthesis, streamlining the process.
- Efficient Replication: The leading strand ensures efficient and rapid replication of the DNA molecule by allowing DNA polymerase to work indefinitely.
- Template for Complementary Strand: DNA Polymerase uses the leading strand as a guide to synthesizing the complementary strand, ensuring the accuracy of genetic information.
- Directionality and Replication Fork: Synthesis of the leading strand occurs in the same direction as replication fork movement, allowing for synchronized replication.
- Fidelity of Genetic Information: The leading strand contributes to the integrity and fidelity of the genetic information being copied by playing a central role in DNA replication.
How does the leading strand differ from the lagging strand in terms of replication?
DNA replication is a fascinating choreography where the leading and lagging strands play unique roles. Let’s explore the intriguing differences between these two essential components of DNA replication:
- Synthesis Direction:
- Leading Strand: The leading strand is synthesized in the 5′ to 3′ direction, which corresponds to the replication fork movement. This allows for efficient and continuous replication.
- Lagging Strand: The lagging strand is synthesized in the 5′ to 3′ direction, but in the opposite direction of the replication fork movement. This makes continuous replication difficult.
- Continuous vs. Discontinuous Synthesis:
- Leading Strand: Because the leading strand is continuous, DNA polymerase can work along the template strand without interruption.
- Lagging Strand: The lagging strand is subjected to discontinuous synthesis. It’s broken down into small fragments known as Okazaki fragments, each of which requires its own primer and synthesis.
- Priming Frequency:
- Leading Strand: A single RNA primer initiates leading strand synthesis at the replication origin.
- Lagging Strand: The lagging strand requires multiple RNA primers, as a new primer is needed for each Okazaki fragment.
- Okazaki Fragment Processing:
- Leading Strand: No Okazaki fragments are formed on the leading strand, leading to straightforward replication.
- Lagging Strand: Okazaki fragments are synthesized on the lagging strand and need to be later joined by DNA ligase.
- Replication Challenges:
- Leading Strand: While the leading strand requires accurate replication, it faces fewer challenges due to its continuous nature.
- Lagging Strand: The lagging strand faces more challenges due to the discontinuous synthesis, creating potential opportunities for errors.
- Efficiency and Speed:
- Leading Strand: Continuous synthesis on the leading strand allows for efficient and rapid replication.
- Lagging Strand: The discontinuous synthesis on the lagging strand may slow down the replication process.
The leading strand elegantly facilitates continuous, accurate synthesis in the grand symphony of DNA replication, while the lagging strand copes with its discontinuous nature, ultimately ensuring the faithful transmission of genetic information. These distinctions highlight the complex mechanisms at work during DNA replication.
What is the significance of the 3′ to 5′ directionality in DNA synthesis on the leading strand?
5 Key Points on the Importance of 3′ to 5′ Directionality in Leading Strand DNA Synthesis:
- Base Pairing Consistency: The 3′ to 5′ directionality ensures that DNA synthesis on the leading strand adheres to the complementary base-pairing rules (adenine with thymine, guanine with cytosine), preserving genetic fidelity.
- Proofreading Mechanism: The enzyme responsible for DNA synthesis, DNA polymerase, has proofreading capabilities in the 3′ to 5′ direction. This enables it to correct errors in the newly synthesized strand, thereby improving replication accuracy.
- Primer Formation: DNA synthesis starts with the formation of a short RNA primer, which serves as the 3′ end to which DNA polymerase can attach. This process of initiation is required for continuous synthesis on the leading strand.
- Directional Replication: The leading strand’s 3′ to 5′ directionality ensures that DNA replication proceeds in the same direction as the replication fork, coordinating the replication machinery’s activities.
- Compatibility with Lagging Strand: The leading strand’s 3′ to 5′ orientation complements the lagging strand’s 5′ to 3′ orientation. Because of this compatibility, both strands can be synthesized concurrently, allowing for efficient and accurate DNA replication.
What enzymes are involved in the replication of the leading strand?
A Comprehensive Guide to the Enzymes Involved in Leading Strand DNA Replication:
- DNA Helicase: At the replication fork, this enzyme unwinds the double-stranded DNA, resulting in a single-stranded template for replication.
- Primase: Primase generates short RNA primers on the leading strand template, allowing DNA synthesis to begin.
- DNA Polymerase III: The primary enzyme in charge of extending the leading strand by adding complementary nucleotides to the growing DNA chain.
- Sliding Clamp: Also known as PCNA (Proliferating Cell Nuclear Antigen), this protein aids in the stabilization of DNA polymerase on the template, thereby increasing processivity.
- DNA Polymerase I: This enzyme replaces RNA primers with DNA nucleotides, ensuring the leading strand’s continuity.
- Ligase: DNA ligase closes the gaps between newly synthesized DNA fragments (Okazaki fragments) on the lagging strand and any nicks on the leading strand.
- Topoisomerases: These enzymes relieve the torsional stress caused by unwinding the DNA helix, preventing the DNA from overwinding or tangling during replication.
- Single-Stranded Binding Proteins (SSBs): These proteins bind to single-stranded DNA, preventing it from forming double-stranded structures and thus stabilizing the unwound template.
- RNAse H: RNAse H removes RNA primers, allowing DNA polymerase to replace them with DNA nucleotides.
- Exonuclease: Exonuclease activity in DNA polymerase allows it to proofread newly synthesized DNA, increasing replication accuracy.
How is the continuous synthesis of the leading strand accomplished during DNA replication?
By following these below steps, the continuous synthesis of the leading strand ensures efficient and accurate DNA replication.
- Continuous Template: The leading strand serves as a continuous template for DNA synthesis, allowing the process to proceed uninterrupted.
- 3′ to 5′ Directionality: The leading strand is oriented in the 3′ to 5′ direction, which corresponds to the 5′ to 3′ direction of DNA synthesis by DNA polymerase.
- Single Primer: Unlike the lagging strand, which requires multiple primers for each Okazaki fragment, the leading strand initiates replication with a single RNA primer.
- Replication Fork Synchronization: The leading strand is synthesized in the same direction as the replication fork movement, ensuring that the replication process is coordinated and synchronized.
- The efficiency of DNA Polymerase: DNA polymerase can continuously synthesize the leading strand by moving along the template and sequentially adding nucleotides.
- Ligase Sealing: Following the completion of the continuous synthesis process by DNA polymerase, DNA ligase seals any remaining gaps.
- Minimal RNA Primer Removal: Because the leading strand only requires one RNA primer, there is less primer removal required than for the lagging strand, simplifying the overall process.
- Fidelity Checkpoints: To ensure accurate replication of the genetic information, the continuous synthesis of the leading strand passes through fidelity checkpoints.
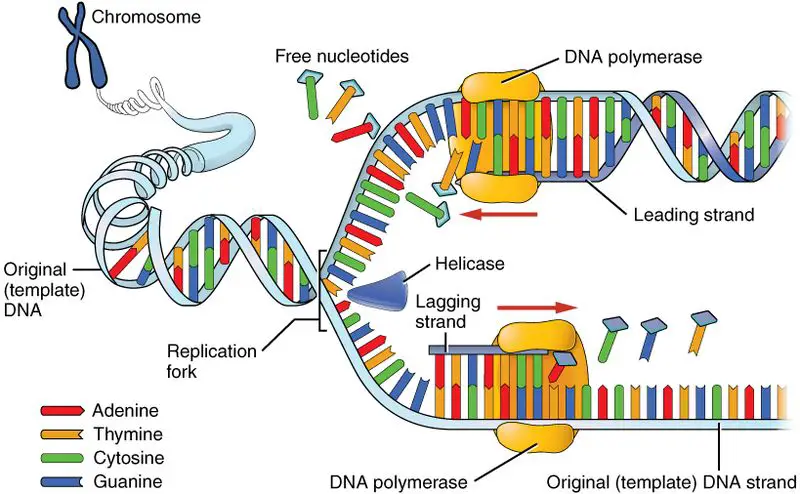
What is the importance of the primer in leading strand replication?
A 5-Point Listicle on the Importance of Primers in Leading Strand Replication:
- Initiating DNA Synthesis: Primers are short RNA sequences that serve as starting points for DNA synthesis. Primers are required in leading strand replication to start the synthesis of the complementary DNA strand.
- Directionality: DNA polymerase can only add nucleotides in the 5′ to 3′ direction, and it must begin with an existing strand. This initial segment is provided by primers, allowing DNA polymerase to elongate the strand in the correct direction.
- Stabilizing DNA Polymerase: Primers act as a platform for DNA polymerase to attach and begin synthesising the new DNA strand. Without a primer, DNA polymerase would have difficulty starting replication.
- Ensure Accuracy: Primers aid in the replication of DNA. They are intended to base-pair with the template strand, acting as a template for the precise placement of complementary nucleotides, thereby reducing errors.
- Okazaki Fragment Synthesis: While primers are more commonly associated with the lagging strand, they also play a role in leading strand replication. New primers are required for continuous Okazaki fragment synthesis on the lagging strand as the replication fork progresses. Primers play a role in this intricate process that promotes overall DNA replication.
In summary, primers in leading strand replication act as essential starting points, guiding the accurate and efficient synthesis of the complementary DNA strand, ultimately contributing to the faithful replication of genetic information.
How does the replication fork affect the leading strand during DNA synthesis?
The Impact of the Replication Fork on the Leading Strand: 5 Key Effects
- Directional Synthesis: The replication fork influences the leading strand by allowing DNA synthesis to occur in the same direction as the replication fork’s movement, ensuring continuous replication.
- Continuous Synthesis: The presence of the replication fork allows the leading strand to be synthesized continuously, eliminating the need for repeated starting points.
- Leading Strand Stability: The replication fork keeps the leading strand from degrading or unraveling during replication.
- Coordination with Enzymes: The replication fork facilitates the coordination of essential enzymes such as DNA polymerase, primase, and helicase, all of which work in tandem on the leading strand.
- Ensuring Efficient Replication: The replication fork contributes to the efficient replication of the leading strand, ensuring that the genetic information is accurately copied and transmitted to the daughter cells.
Are there any specific challenges or issues that arise during leading strand replication?
Specific challenges or issues that arise during leading strand replication:
- Strand Asymmetry: Because the leading strand is the continuous template, it must be synthesized continuously in the 3′ to 5′ direction, resulting in inherent asymmetry with respect to the lagging strand.
- Replication Fork Dynamics: Managing replication fork movement along the leading strand can be difficult, particularly in regions with a variety of secondary structures or obstacles.
- RNA Priming: Leading strand synthesis necessitates the use of an initial RNA primer, which must be carefully coordinated in order to initiate DNA synthesis.
- Polymerase Switch: It can be difficult to coordinate the transition from the primase-initiated RNA primer to DNA polymerase for continuous synthesis on the leading strand.
- Proofreading Complexity: The proofreading mechanism of DNA polymerase must be finely balanced in order to maintain fidelity without stalling or slowing down the replication process.
- Telomeres: Telomeres, the end regions of linear chromosomes, pose a challenge during leading strand replication due to incomplete replication and potential genetic information loss.
- Nucleotide Availability: It can be difficult to ensure an adequate supply of nucleotides for the rapid synthesis of the leading strand.
- DNA Damage: The leading strand is also vulnerable to various types of DNA damage, which can obstruct accurate replication.
- Replication Fidelity: Errors in leading strand replication can result in mutations or structural changes in the DNA, compromising the genome’s integrity.
- Coordination with Lagging Strand: To ensure that the entire DNA molecule is accurately replicated, the leading and lagging strands must be coordinated, which requires precise timing and synchronization of various enzymatic processes.
What is the relationship between the Okazaki fragments on the lagging strand and the leading strand?
The Relationship Between Okazaki Fragments and the Leading Strand:
- Different Synthesis Paths: Okazaki fragments and the leading strand are both essential components of DNA replication, but they serve different functions.
- Leading Strand: The leading strand is continuously synthesized in the 5′ to 3′ direction, with a single primer used to initiate DNA synthesis.
- Okazaki Fragments: The lagging strand, on the other hand, is synthesized in a more complicated way. It is composed of shorter segments known as Okazaki fragments.
- Lagging Strand Difficulties: Because DNA is antiparallel, the lagging strand is synthesized in the opposite direction as the replication fork movement, requiring the formation of Okazaki fragments.
- Fragment Assembly: These fragments are later joined to form a complete complementary strand to the parental DNA.
- The Role of DNA Ligase: An enzyme called DNA ligase is critical in closing the gaps between Okazaki fragments, resulting in continuous double-stranded DNA.
- Replication Efficiency: Despite the challenges posed by the antiparallel nature of the DNA structure, this process ensures that both strands of the DNA molecule are replicated with high fidelity.
- Genetic Diversity: Surprisingly, the presence of Okazaki fragments and recombination events during DNA replication contribute to offspring genetic diversity.
- Coordinated Action: During replication, the leading strand, and Okazaki fragments work together to ensure that the entire DNA molecule is accurately copied.
- Overall Importance: Understanding the relationship between Okazaki fragments on the lagging strand and continuous synthesis on the leading strand is critical for understanding the complexities of
How does the proofreading mechanism ensure the accuracy of DNA synthesis on the leading strand?
Here’s a listicle-style answer explaining how the proofreading mechanism ensures the accuracy of DNA synthesis on the leading strand:
- DNA Polymerase: The proofreading mechanism is primarily based on the DNA polymerase enzyme, which is in charge of synthesizing new DNA strands. DNA polymerase has an inherent proofreading ability that improves the fidelity of DNA replication.
- Exonuclease Activity: DNA polymerase has a unique property known as 3′ to 5′ exonuclease activity. This means it can “proofread” its work by detecting and removing mismatched or incorrect nucleotides that may have been incorporated during DNA synthesis.
- Base Pairing: DNA polymerase is extremely selective when it comes to base pairing. It ensures that complementary base pairs (adenine with thymine and guanine with cytosine) are correctly added to the growing strand.
- Monitoring the Fit: As each nucleotide is added to the growing DNA strand, DNA polymerase checks to see if it fits properly with the template strand.
- Maintaining Genetic Information: During DNA replication, the proofreading mechanism is critical for preserving genetic information. Accurate replication is required to ensure that the genetic code remains consistent and that errors that could lead to mutations are minimized.
- Overall Accuracy: The proofreading mechanism dramatically reduces the chances of errors in DNA synthesis on the leading strand due to the combined action of DNA polymerase’s accurate base pairing, the ability to detect mismatched nucleotides, and the exonuclease activity.
- Minimizing Mutations: By correcting errors in real-time, the proofreading mechanism contributes to the integrity of the DNA molecule, preventing the accumulation of mutations that could lead to genetic disorders or other problems.
- Biological Importance: The accuracy ensured by the proofreading mechanism is critical for the proper functioning of cells and an organism’s overall health. During cell division, it ensures that genetic information is faithfully passed on to daughter cells.
- Evolutionary Advantage: Organisms with efficient proofreading mechanisms have an evolutionary advantage in terms of genetic stability and adaptability. This advantage enables them to respond more effectively to changing environments over generations.
- Balancing Speed and Accuracy: While the proofreading mechanism improves accuracy, it is important to note that it does not eliminate all errors in DNA replication. It strikes a balance between speed and accuracy, ensuring efficient replication while minimizing errors in the genetic code.
CONCLUSION
The DNA leading strand has revealed itself to be more than just a component of genetic replication. It is a conductor conducting the symphony of inheritance, a blueprint dictating the formation and operation of all living organisms. We have discovered the essence of our existence, the code that connects us to the diversity of life on this planet, by studying its mechanisms, significance, and evolutionary impact.
Our journey has taken us from the elegance of continuous synthesis to the profundity of genetic fidelity, demonstrating the critical role of the leading DNA strand in maintaining the integrity of the genetic information passed down from generation to generation. It has led us down a path of medical breakthroughs, where our understanding of the role of the leading strand in heredity opens up new avenues for personalized medicine, genetic therapies, and the treatment of inherited diseases.
The role of the leading DNA strand in the grand story of evolution has served as a guidepost for us, allowing us to trace the branches of the tree of life and illuminate the paths of adaptation that have shaped the biodiversity we value. Its significance spans time, from the dawn of life to the cutting edge of biotechnology, providing us with the tools to engineer life for the betterment of society and the preservation of our planet’s natural wonders.
We discovered deep connections between replication, inheritance, evolution, and the limitless possibilities that lie in the mastery of the leading DNA strand during our research. As we stand on the brink of scientific discovery, we have the knowledge to unravel the complexities of life’s blueprint and pave the way for a future in which the secrets of the leading strand are harnessed for the advancement of humanity, understanding of our place in the cosmos, and preservation of the magnificent diversity of life that surrounds us.