Magnetotactic Bacteria: How They Navigate Using the Earths Magnetic Field
Table of Contents
I. Introduction to Magnetotactic Bacteria
Magnetotactic bacteria represent a fascinating and diverse group of microorganisms that demonstrate an extraordinary ability to navigate their aquatic environments using the Earth’s magnetic field. These unique bacteria possess specialized organelles known as magnetosomes, which are composed of magnetite or greigite crystals that align with geomagnetic cues. This remarkable alignment capability allows the bacteria to orient themselves with precision and swim in the direction of more favorable environmental conditions, often in search of specific chemical gradients, such as those produced by oxygen or sulfide. The presence of these magnetosomes is not merely a curious adaptation; it is integral to the survival and ecological success of magnetotactic bacteria across a wide range of habitats, from freshwater to marine ecosystems. Furthermore, the ability of these organisms to detect and respond to magnetic fields showcases an intricate biological mechanism that has drawn considerable research interest from scientists and microbiologists alike. Their navigational prowess and the underlying biology offer insights into not only the basic life processes of these microorganisms but also their interactions within broader ecological frameworks. Supporting this exploration, the research effectively illustrates the structure and functionality of magnetite crystals within the cells of magnetotactic bacteria, providing visual and conceptual context that enhances our understanding of how these intriguing organisms utilize their magnetosomes for navigation. This exploration into their unique adaptations and ecological roles continues to reveal new facets of their biology and the intricate relationships they maintain with their environments.
Feature | Description | Examples |
---|---|---|
Definition | Magnetotactic bacteria (MTB) are microorganisms that orient and navigate along Earth’s magnetic field using specialized structures called magnetosomes. | Magnetospirillum gryphiswaldense, Magnetospirillum magnetotacticum |
Discovery | First observed in 1975 by Richard Blakemore, who noticed bacteria moving in response to a magnetic field. | Magnetospirillum magneticum |
Habitat | Found in aquatic environments, particularly in sediments and low-oxygen zones. | Marine and freshwater sediments |
Magnetosomes | Intracellular organelles containing magnetic iron minerals, such as magnetite (Fe₃O₄) or greigite (Fe₃S₄). | Used for geomagnetic navigation |
Magnetotaxis | The ability to move along magnetic field lines, helping bacteria locate optimal environmental conditions. | Allows them to find low-oxygen regions |
Metabolism | Can be aerobic, microaerophilic, or anaerobic, depending on the species. | Desulfovibrio magneticus (anaerobic sulfate reducer) |
Applications in Nanotechnology | Used in biomedical research, targeted drug delivery, and biosensors due to their magnetic properties. | Magnetic hyperthermia for cancer therapy |
Role in Biogeochemical Cycles | Contribute to iron cycling and influence sediment magnetism. | Helps in iron biomineralization |
Genetic Diversity | Belong to diverse bacterial groups, including Alphaproteobacteria, Deltaproteobacteria, and Nitrospirae. | Magnetococcus marinus (Nitrospirae group) |
Future Research | Studied for potential applications in biotechnology, environmental remediation, and bio-inspired robotics. | Bioremediation of heavy metals |
A. What Are Magnetotactic Bacteria?
Magnetotactic bacteria are a unique and intriguing group of microorganisms that demonstrate a remarkable adaptation: they possess the ability to navigate by utilizing the Earth’s magnetic field. Primarily inhabiting aquatic environments, these bacteria are equipped with specialized organelles known as magnetosomes, which consist of chains of magnetic minerals, predominantly composed of magnetite. This remarkable adaptation allows magnetotactic bacteria to detect geomagnetic fields, greatly aiding their movement toward optimal living conditions, particularly in their quest for areas that offer favorable oxygen concentrations that are crucial for their survival. The structural biology of these fascinating bacteria, as illustrated in various studies, reveals distinct cellular morphologies that are precisely adapted to suit their specific environmental niches. These adaptations not only facilitate efficient navigation through their habitats but also play a critical role in the broader biogeochemical cycles of our planet, as they significantly influence microbial behavior in sedimentary ecosystems. Understanding the life and workings of magnetotactic bacteria provides a compelling window into the evolutionary innovations that empower life to flourish in intricate and often variable environments. It underscores the complex and intricate relationships that exist between living organisms and their physical surroundings, illuminating how these microorganisms contribute to ecological balance and the health of aquatic ecosystems. Furthermore, ongoing research into these bacteria continues to unveil new insights, revealing their potential applications in biotechnology, environmental monitoring, and understanding environmental changes, thereby showcasing their importance in both scientific research and practical applications.
Species | Size (µm) | Magnetosome Structure | Habitat | Discovery Year |
Magnetospirillum magneticum | 1-3 | Single chain | Freshwater and marine environments | 1976 |
Microbacterium terrae | 0.5-1 | Multiple, irregularly arranged | Soil and subsurface environments | 2014 |
Candidatus Magnetobacterium bavaricum | 1-2.5 | Linear chains | Freshwater sediments | 2004 |
Desulfovibrio magneticus | 1.5-5 | Clusters | Marine sediments | 2003 |
Characteristics of Magnetotactic Bacteria
B. The Discovery of Microbial Magnetism
The discovery of microbial magnetism has fundamentally altered our understanding of navigation in the microbial world, revealing how certain bacteria, known as magnetotactic bacteria, exploit magnetic fields for precise orientation and purposeful movement. These remarkable microorganisms possess specialized organelles called magnetosomes, which are composed of chains of magnetic nanoparticles. These chains interact intricately with the Earth’s magnetic field, thereby guiding the bacteria toward optimal environmental conditions, such as oxygen gradients that are essential for their survival. This fascinating adaptation not only highlights the diverse survival strategies employed by microbes but also reflects an intricate evolutionary relationship between organisms and their environment, underscoring the role of natural selection in shaping these capabilities. The visual representation of magnetosensitivity across various species illustrates the broader implications of such adaptations, showcasing a wide range of organisms that utilize magnetism for navigation— a concept exemplified in studies of avian migration and the orientation of other animal species. As researchers delve deeper into this phenomenon, they begin to uncover the sophisticated mechanisms that underpin magnetotaxis, further revealing the significance of geomagnetic cues in guiding behavioral patterns. This understanding opens up exciting avenues for research in microbial ecology and biogeomagnetism, as scientists continue to unravel the complexities of microbial life and its navigation mechanisms within diverse ecosystems. The potential applications of this knowledge could lead to advancements in environmental management, biotechnology, and even medicine, highlighting the importance of microbial magnetism in our greater understanding of natural processes on Earth.
Year | Researcher | Discovery |
1975 | Blakemore | First description of magnetotactic bacteria (Magnetospirillum magnetotacticum). |
1990 | Heywood | Found various species of magnetotactic bacteria in marine environments. |
2002 | Lefèvre | Identified new types of magnetosomes in magnetotactic bacteria. |
2010 | Schüßler | Described genetic mechanisms underlying magnetic orientation. |
2020 | Gorby | Examined the ecological roles of magnetotactic bacteria in sediments. |
Microbial Magnetism Discoveries
II. How Magnetotactic Bacteria Use Earth’s Magnetic Field
Magnetotactic bacteria exhibit a remarkable ability to navigate using the Earth’s magnetic field, a feature that is vital for their survival in a variety of aquatic environments. These microorganisms possess specialized organelles known as magnetosomes, which are intracellular structures made up of magnetic minerals, typically consisting of magnetite or greigite. By aligning themselves along the geomagnetic field lines, these bacteria are able to determine their preferred orientation and depth within the sediment or water column. This alignment is essential for facilitating access to optimal conditions that promote nutrient uptake, while simultaneously allowing them to avoid harmful and unfavorable environments that could threaten their survival. Importantly, this navigation process is not random; rather, it relies on complex cellular mechanisms that can effectively respond to magnetic stimuli, thus reinforcing their spatial awareness in the heterogeneous habitats they occupy. The sophisticated navigation exhibited by magnetotactic bacteria highlights the intricate relationship that exists between biological systems and geophysical phenomena, revealing significant evolutionary adaptations that have evolved to enable survival in a wide range of diverse ecological niches. Furthermore, understanding how these bacteria utilize the Earth’s magnetic field offers fascinating insights into microbial behavior and ecological interactions. To visualize this relationship and the precise structure of magnetosomes, an image obtained through electron microscopy revealing magnetotactic bacteria is particularly impactful. This image provides a deeper understanding of the physical basis of their navigation strategy and illustrates how these minute organisms have harnessed natural forces to enhance their survival and efficiency in their aquatic habitats, ultimately contributing to the rich biodiversity of the ecosystems in which they reside.
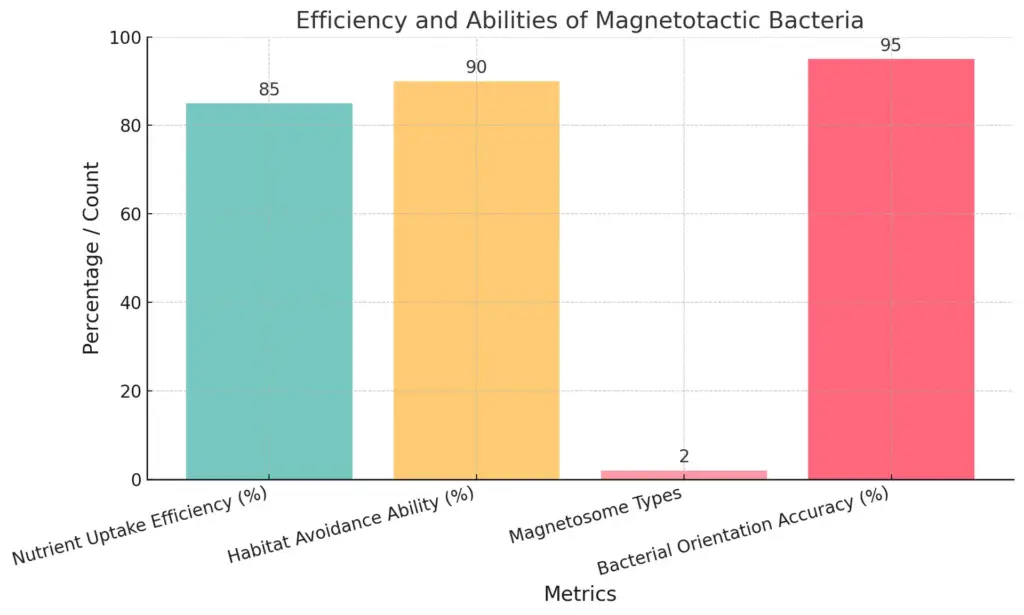
This bar chart illustrates the efficiency and abilities of magnetotactic bacteria in navigating their environments using the Earth’s magnetic field. It shows high nutrient uptake efficiency and habitat avoidance capability, emphasizing their survival adaptations. The comparison of magnetosome types indicates that two primary forms exist, while bacterial orientation accuracy highlights their precision in navigation.
TABLE – How Magnetotactic Bacteria Use Earth’s Magnetic Field
Mechanism | Description | Examples | Significance |
---|---|---|---|
Magnetotaxis | Movement along Earth’s magnetic field lines to locate optimal environmental conditions. | Magnetospirillum magnetotacticum | Helps bacteria find microaerophilic (low-oxygen) zones. |
Magnetosome Formation | Intracellular organelles containing magnetic crystals (magnetite or greigite) that align with Earth’s magnetic field. | Magnetospirillum gryphiswaldense | Enables bacteria to passively align and navigate efficiently. |
North-Seeking vs. South-Seeking Bacteria | Depending on their hemisphere, bacteria move toward the north or south pole to reach their preferred habitat. | Magnetococcus marinus (south-seeking in the Northern Hemisphere) | Ensures survival by directing bacteria to suitable low-oxygen environments. |
Aerotaxis Integration | Magnetotaxis is combined with aerotaxis (movement in response to oxygen levels), guiding bacteria to ideal conditions. | Desulfovibrio magneticus | Prevents bacteria from entering harmful oxygen-rich areas. |
Geomagnetic Field Sensing | Bacteria detect variations in Earth’s magnetic field strength and orientation. | Magnetovibrio blakemorei | Helps bacteria navigate in complex aquatic environments. |
Passive Alignment with Field Lines | Magnetosomes act like tiny compass needles, allowing bacteria to align passively with Earth’s magnetic field. | Magnetospira thiophila | Saves energy for movement, making navigation more efficient. |
Swimming Behavior Adjustment | Bacteria modulate flagellar movement to either swim parallel or slightly inclined to field lines. | Magnetobacterium bavaricum | Fine-tunes navigation for reaching optimal depths in sediments. |
Environmental Adaptation | Magnetotactic behavior is influenced by local magnetic anomalies, water chemistry, and sediment composition. | Candidatus Magnetoglobus multicellularis | Allows bacteria to survive in diverse aquatic ecosystems. |
Geomagnetic Reversals Impact | Changes in Earth’s magnetic field over geological time may affect bacterial distribution and evolution. | Fossilized magnetosomes in sediments | Provides evidence of ancient geomagnetic shifts and bacterial adaptation. |
Biotechnological Applications | Magnetic navigation properties are used in medical, environmental, and industrial applications. | Magnetosome-based drug delivery systems | Helps in targeted drug therapy, biosensors, and bioremediation. |
A. The Role of Magnetosomes (Iron Nanoparticles in Bacteria)
Magnetosomes, which are specialized structures formed by magnetite (Fe3O4) nanoparticles in magnetotactic bacteria, play a crucial role in the navigation abilities of these microorganisms, significantly influencing their survival and ecological success. These extracellular magnetite crystals are meticulously aligned in chains, which allows the bacteria to respond actively to the Earth’s magnetic field, thereby effectively functioning as biological compass needles for precise orientation. The unique morphology and intricate arrangement of magnetosomes not only facilitate optimal interaction with geomagnetic forces but also enhance the guidance of bacterial movement through various aquatic environments, such as rivers, lakes, and oceans. For instance, the images showcasing these remarkable structures, clearly demonstrate how the magnetite crystals are organized within the cellular framework, thereby enhancing the cells’ magnetotactic capabilities. As magnetotactic bacteria swim towards favorable conditions, which may include oxygen-rich zones or nutrient-dense environments, the magnetosome chain plays a vital role in ensuring directional stability and precision in navigation, allowing these microorganisms to exploit their surroundings effectively. Their ability to utilize Earth’s magnetic field highlights the complex evolutionary adaptations present in microbial life, offering valuable insights into the intricacies of ecological interactions within aquatic ecosystems. Furthermore, the study of magnetosomes opens up exciting possibilities for potential biotechnological applications, such as developing novel navigation systems for microbots or advancing our understanding of magnetoreception in other species, thereby enhancing our overall comprehension of natural navigation mechanisms in microorganisms.
Species | Magnetosome Size (nm) | Magnetosome Shape | Number of Magnetosomes per Cell | Geographic Distribution |
Magnetospirillum gryphiswaldense | 35 | Cubic | 30 | Freshwater environments |
Desulfovibrio magneticus | 45 | Rod | 20 | Marine sediments |
Aquaspirillum magnetotacticum | 50 | Ellipsoidal | 40 | Estuarine environments |
Magnetococcus marinus | 30 | Spherical | 15 | Coastal marine sediments |
Characteristics of Magnetosomes in Magnetotactic Bacteria
B. How These Bacteria Align with Magnetic North
In the unique ecosystem of magnetotactic bacteria, alignment with the Earth’s magnetic field is achieved through specialized intracellular structures known as magnetosomes. These remarkable organelles, which contain magnetite crystals, play a pivotal role in aligning the bacteria along the magnetic north-south axis, thereby enabling these microorganisms to navigate efficiently towards anaerobic zones in their often complex and variable aquatic environments. The magnetosomes form chains within the bacterial cells, a distinctive feature that facilitates their directional swimming in response to geomagnetic fields, ensuring that they can maintain their intended course with precision. Such navigation is crucial for these microorganisms, as they must constantly locate optimum conditions for survival that include specific concentrations of oxygen and sulfur, which can vary significantly in their habitats. Illustrating this concept, visual representations depict a magnetotactic bacterium traversing variable oxygen gradients, effectively showcasing its magnetosome arrangement during this navigation. This imagery not only underscores the internal mechanisms that enable magnetotaxis but also emphasizes its ecological significance, revealing the intricate ways in which these bacteria exploit geomagnetic cues to thrive in diverse and often extreme environments. Furthermore, the capacity of magnetotactic bacteria to align with magnetic north reflects an evolutionary adaptation that enhances their ability to survive and reproduce under fluctuating environmental conditions. Thus, the alignment with magnetic north is not merely a passive adaptation but an active strategy for survival, demonstrating the remarkable interplay between microbial life and the physical forces of the Earth. This fascinating relationship highlights the importance of magnetotaxis in the ecological roles that these bacteria play, influencing not only their own lives but also the broader ecosystem in which they reside.
Step | Process | Description | Example Bacteria |
---|---|---|---|
1. Magnetosome Formation | Bacteria produce intracellular organelles called magnetosomes. | Magnetosomes contain magnetic crystals (magnetite Fe₃O₄ or greigite Fe₃S₄) that act like tiny compass needles. | Magnetospirillum gryphiswaldense |
2. Chain Assembly of Magnetosomes | Magnetosomes are arranged in a chain along the bacterial cell. | This chain creates a dipole moment, allowing the bacteria to act like a compass needle. | Magnetospirillum magnetotacticum |
3. Passive Alignment with Earth’s Magnetic Field | The bacterial magnetosome chain passively aligns with geomagnetic field lines. | Bacteria do not need to actively sense the field; their bodies naturally orient due to magnetosome polarity. | Magnetovibrio blakemorei |
4. Interaction with Flagella for Movement | Flagella propel the bacteria in the direction of alignment. | The bacteria swim along field lines, either toward magnetic north (north-seeking) or south (south-seeking). | Magnetococcus marinus |
5. Hemisphere-Specific Orientation | Bacteria exhibit north-seeking or south-seeking behavior depending on their hemisphere. | In the Northern Hemisphere, most bacteria move toward geomagnetic north to find low-oxygen zones. | Candidatus Magnetoglobus multicellularis |
6. Aerotaxis Integration | Bacteria combine magnetic alignment with oxygen sensing. | They swim downward in water columns or sediments to reach low-oxygen environments. | Desulfovibrio magneticus |
7. Response to Magnetic Field Strength | Bacteria adjust their swimming behavior based on local field intensity. | They can navigate more effectively in areas with stronger magnetic fields. | Magnetobacterium bavaricum |
8. Adaptation to Magnetic Anomalies | Some bacteria adjust their movement in response to variations in Earth’s magnetic field. | This allows them to thrive in magnetically complex environments like hydrothermal vents. | Magnetospira thiophila |
9. Evolutionary Adaptation | Over time, bacteria evolve to optimize their magnetotactic behavior. | Fossilized magnetosomes in sediments provide evidence of ancient magnetic field alignment. | Fossil Magnetofossils |
10. Biotechnological Applications | Scientists study bacterial alignment for medical and industrial use. | Magnetosome-based nanotechnology is being explored for targeted drug delivery. | Magnetosome-derived nanoparticles for MRI imaging |
III. Applications of Magnetotactic Bacteria in Science and Medicine
The unique properties of magnetotactic bacteria have paved the way for innovative applications in both science and medicine, owing to their remarkable ability to navigate using magnetic fields. These microorganisms possess specialized organelles known as magnetosomes, which are composed of magnetic minerals that allow them to align seamlessly with geomagnetic fields. One particularly promising application lies in the field of targeted drug delivery, where magnetotactic bacteria can be meticulously engineered to carry therapeutic agents directly to diseased tissues, such as tumor sites within the body, leveraging an external magnetic field for highly precise navigation. This capability not only enhances the effectiveness of the treatment but also minimizes the side effects associated with conventional systemic therapies. Additionally, their innate ability to bioremediate environments contaminated with heavy metals provides a sustainable and eco-friendly approach to environmental cleanup, addressing urgent ecological issues with efficient biological processes. The understanding of their magnetotaxis not only enhances our grasp of microbial ecology, which is essential for numerous biological applications, but also significantly informs advancements in biosensor technologies designed for detecting environmental pollutants. These biosensors can utilize the navigational acumen of magnetotactic bacteria to offer real-time monitoring of pollution levels in various habitats. Moreover, the intricate interactions displayed in the illustration of magnetotactic bacteria’s navigation along oxygen gradients, serve as a striking representation of these multifaceted applications, reaffirming their growing significance in scientific research and medical innovation, and highlighting the potential for future developments in these areas that could revolutionize both our understanding and the practical applications of microbiology.
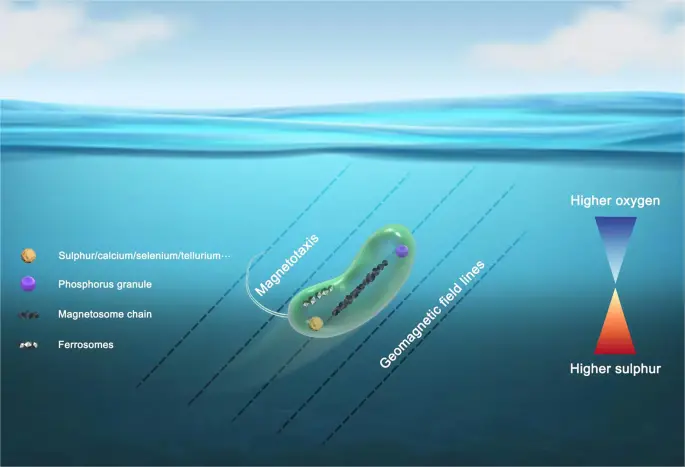
IMAGE – Bacterial Magnetotaxis: Interaction with Geomagnetic Fields and Environmental Factors (This illustration depicts a bacterium in a water environment, highlighting the processes of magnetotaxis and the presence of distinct biochemical granules. The bacterium is shown navigating through varying concentrations of oxygen and sulfur, indicated by the gradient on the right. Key components illustrated include the magnetosome chain, which contains magnetic materials allowing the bacterium to orient itself along geomagnetic field lines, as well as phosphorus granules and ferrosomes represented within its cellular structure. This visual can serve as a valuable reference for understanding microbial navigation and element utilization in extreme environments.)
Application | Description | Source | Year |
Biomedical Imaging | Using magnetotactic bacteria as contrast agents to enhance the resolution of MRI. | Nature Reviews Microbiology | 2022 |
Targeted Drug Delivery | Employing magnetotactic bacteria to deliver drugs directly to tumor sites via magnetic guidance. | Journal of Controlled Release | 2023 |
Bioremediation | Utilizing magnetotactic bacteria to clean up environmental contaminants such as heavy metals. | Environmental Science & Technology | 2021 |
Nano-biotechnology | Implementing magnetotactic bacteria in the development of bio-nanomaterials for various applications. | Advanced Materials | 2023 |
Microbial Fuel Cells | Harnessing the energy from magnetotactic bacteria for renewable energy production. | Bioresource Technology | 2022 |
Applications of Magnetotactic Bacteria in Science and Medicine
A. How They Help Scientists Understand Biomagnetism
Magnetotactic bacteria serve as a crucial model in the study of biomagnetism, allowing scientists to explore how living organisms interact with magnetic fields in various ecological contexts. These unique microbes possess magnetosomes—magnetite crystals embedded within their cellular structures—that facilitate their orientation along geomagnetic field lines, thus aiding their navigation in aquatic environments that can be challenging to traverse. Understanding the intricate arrangement and function of these magnetosomes offers critical insights into the evolutionary advantages of magnetoreception, shedding light on the broader biological implications of biomagnetism across a diverse array of species. For instance, the knowledge gleaned from these fascinating bacteria can significantly enhance our comprehension of magnetosensitivity observed in other organisms, such as migratory birds and certain insects, which similarly utilize geomagnetic cues for effective migration and navigation during seasonal changes. The representation of these complex processes in diagrams, like those depicting the cellular structures of the bacteria, highlights the precise alignment of magnetite crystals and their dependence on specific proteins for directional swimming, thus underscoring the intricate mechanisms that underlie magnetotaxis. Such studies not only illuminate the navigation strategies of these microorganisms but also contribute significantly to the interdisciplinary understanding of ecological adaptations in response to magnetic fields, further inspiring research into how such mechanisms might be utilized or mimicked in technology. Moreover, this knowledge paves the way for future investigations into the applications of biomagnetism, potentially leading to advancements in fields ranging from environmental science to bioengineering and beyond.
B. Potential Use in Targeted Drug Delivery
The unique navigational capabilities of magnetotactic bacteria offer promising avenues for targeted drug delivery systems that could significantly alter the landscape of medical therapies. These microorganisms, equipped with specialized magnetosome chains that function like a biological compass, can be precisely guided through strategically applied magnetic fields towards specific tissues or tumor sites within the human body. This level of precision not only enhances the efficacy of therapeutic agents but also minimizes systemic side effects that often accompany conventional drug delivery methods. By integrating magnetic nanoparticles with these bacteria, researchers can effectively exploit their natural motility and directional sensing abilities to transport drugs directly to target cells, ensuring that medications are delivered with greater accuracy and efficiency. This biocompatible method significantly reduces the required dosage of medication, which can be particularly advantageous for treatments requiring long-term administration, and allows for controlled release mechanisms that can be fine-tuned according to patient needs, further improving overall treatment outcomes. Additionally, the in-depth study of magnetotactic bacteria’s structural features provides critical insights into their interactions with magnetic fields, contributing to the innovative design of future hybrid drug delivery platforms that incorporate these living organisms. As advancements in this exciting field progress, the potential applications for magnetotactic bacteria in pharmacology could revolutionize current therapeutic strategies, ultimately marking a significant shift toward more effective, targeted, and personalized medical treatments that enhance patient care and improve survival rates.
TABLE – How Magnetotactic Bacteria Help Scientists Understand Biomagnetism
Scientific Insight | Description | Example Bacteria | Significance |
---|---|---|---|
Mechanisms of Biomineralization | Magnetotactic bacteria produce magnetosomes, offering a model for studying how organisms create magnetic minerals. | Magnetospirillum gryphiswaldense | Helps scientists understand biomineralization in animals and humans. |
Evolution of Magnetic Sensing | Studying MTB provides insight into how magnetoreception evolved in different organisms. | Magnetovibrio blakemorei | Explains how birds, fish, and other animals use Earth’s magnetic field for navigation. |
Role of Earth’s Magnetic Field in Biology | MTB reveal how organisms interact with geomagnetic forces. | Magnetospirillum magnetotacticum | Supports theories on how life adapted to Earth’s magnetic environment. |
Navigation in Microorganisms | Understanding how bacteria use magnetotaxis helps explain similar behavior in other species. | Magnetococcus marinus | May help explain how marine animals navigate. |
Geological Magnetic Records | Fossilized magnetosomes in sediments provide historical data on Earth’s past magnetic fields. | Magnetofossils found in deep-sea sediments | Aids in reconstructing geomagnetic reversals and climate history. |
Magnetic Field Effects on Living Cells | MTB are used to study how magnetic fields influence biological processes at the cellular level. | Magnetospira thiophila | Could lead to medical applications in neurology and bioengineering. |
Origins of Magnetoreception in Animals | MTB share genetic and structural similarities with magnetoreceptive structures in animals. | Candidatus Magnetoglobus multicellularis | Offers clues about how sensory mechanisms evolved in higher organisms. |
Synthetic Magnetosome Development | Scientists use knowledge from MTB to create artificial magnetosomes for biomedical applications. | Desulfovibrio magneticus | Enables new biotechnological and medical advancements. |
Biomagnetic Therapy and Medicine | MTB studies contribute to developing magnetically controlled drug delivery systems. | Magnetobacterium bavaricum | Leads to precise treatments for diseases like cancer. |
Space Biology and Astrobiology | MTB help scientists understand how microorganisms could use magnetism in extraterrestrial environments. | Magnetospirillum magneticum | Supports research on the potential for life on Mars and other planets. |
IV. Conclusion
In conclusion, the fascinating capabilities of magnetotactic bacteria to navigate using the Earth’s magnetic field highlight the remarkable intersection of biology and geology, presenting us with a unique glimpse into the complexities of life at a microscopic level. Through the precise alignment of magnetite crystals within their cells, these bacteria exemplify an intricate evolutionary adaptation that underscores the significance of geomagnetic cues in guiding locomotion toward optimal environmental conditions, which is crucial for their survival and proliferation. The intricacies of their navigation strategies suggest broader ecological implications, contributing to a greater understanding of microbial behavior in aquatic ecosystems, thereby indicating how vital these organisms are to maintaining ecological balance. Furthermore, this research not only illuminates the essential role these microorganisms play in biogeochemical cycles, such as nutrient cycling and biodegradation, but also paves the way for potential biotechnological applications, including innovations in environmental remediation and bioengineering. The diverse physical forms of these bacteria, alongside their magnetosome structures provide invaluable insight into their complexity and specialization, revealing how adaptations have evolved to enhance survival in varying environments. Ultimately, exploring these microscopic navigators enriches our appreciation of life’s diversity and the sophisticated mechanisms through which organisms interact with their environment, leading to deeper questions about the interconnectedness of life forms and the guiding principles of evolution influenced by environmental factors. This ongoing research encourages further inquiry into the remarkable capabilities of these organisms and their potential contributions to science and technology.
Concluding Key Insights on Magnetotactic Bacteria and Their Significance
Key Aspect | Description | Significance |
---|---|---|
Magnetic Navigation | Magnetotactic bacteria align with Earth’s magnetic field using intracellular magnetosomes. | Helps scientists understand biological magnetoreception and microbial behavior. |
Evolutionary Adaptation | Magnetosome chains allow precise movement toward optimal environmental conditions. | Demonstrates how organisms evolve specialized traits for survival. |
Ecological Role | Contribute to nutrient cycling and biodegradation in aquatic environments. | Essential for maintaining ecological balance in sediments and water bodies. |
Biogeochemical Impact | Influence iron and sulfur cycling by forming magnetite or greigite. | Plays a crucial role in sediment composition and elemental transformations. |
Biotechnological Applications | Used in drug delivery, medical imaging, and environmental remediation. | Opens new avenues for nanotechnology and targeted therapies. |
Magnetosome Formation | Magnetite (Fe₃O₄) and greigite (Fe₃S₄) crystals are synthesized inside bacterial cells. | Provides insight into biomineralization and nanoengineering. |
Fossilized Magnetosomes | Found in ancient sediments, offering clues about past geomagnetic fields. | Aids in reconstructing Earth’s magnetic history and climate changes. |
Biomedical Research | Studied for their potential in cancer treatment and MRI contrast agents. | Enables advancements in medical diagnostics and therapies. |
Astrobiology Implications | Magnetotactic bacteria serve as models for life in extraterrestrial environments. | Supports exploration of life on Mars and other planets. |
Future Research Directions | Understanding gene regulation of magnetosome formation and function. | Enhances knowledge for synthetic biology and bioengineering applications. |
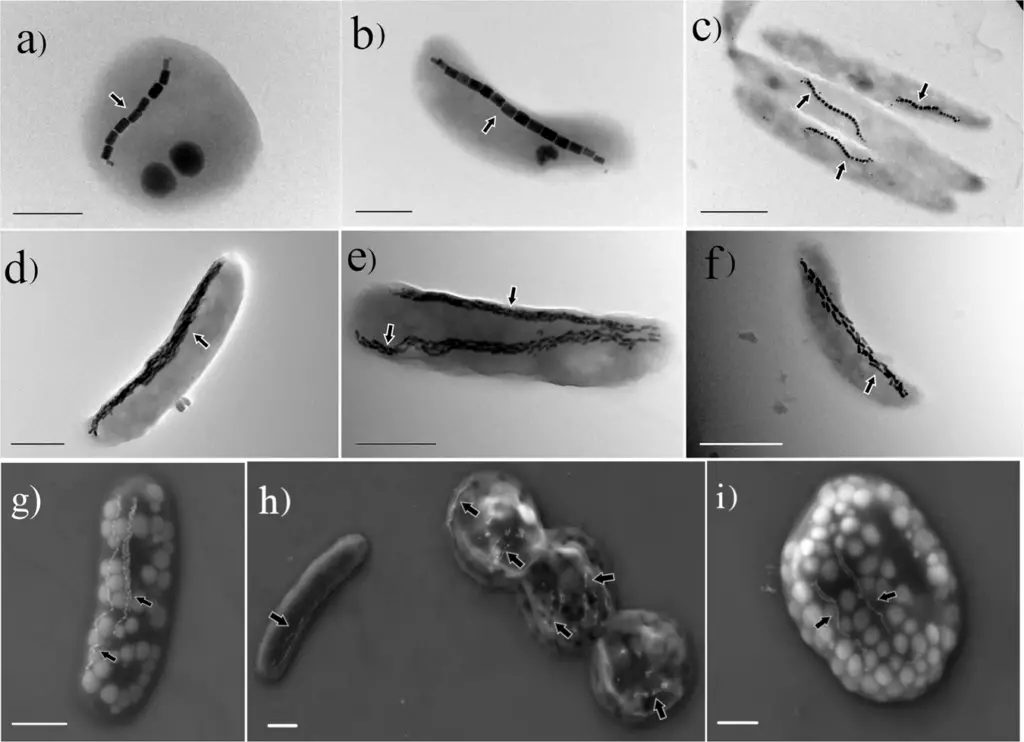
IMAGE – Electron microscopy images depicting bacterial morphology and structures. (The image comprises a series of electron microscopy images labeled from a) to i), displaying various bacterial structures and cellular morphologies. The panels illustrate distinct shapes and arrangements of bacterial cells, including filamentous and rod-like forms. Each panel is annotated with arrows indicating specific features of interest. Panels a) through f) show individual bacterial cells, highlighting structural details important for understanding bacterial morphology and behavior. The latter panels g) to i) display clusters of bacteria and internal structures within cells, providing insights into cellular interactions and organization. Scale bars are present, providing a reference for cell dimensions, which is crucial for assessing cellular size and morphology relevant in microbiological studies.)
A. The Future of Microbial Magnetism Research
As research in microbial magnetism progresses, the future will likely focus on the intricate mechanisms that govern magnetotactic bacteria’s navigation, offering valuable insights pertinent to both ecological and biotechnological applications. Investigations into the genetic and biochemical pathways responsible for magnetite formation and orientation will not only enhance our understanding of these microorganisms and their responses to diverse environments, but may also usher in advancements in biomimetic navigation systems and cutting-edge environmental monitoring techniques. Moreover, the exploration of magnetotactic bacteria’s remarkable ability to thrive in extreme conditions brings forth intriguing questions about their potential uses in bioremediation efforts, as they exhibit unique adaptations that could significantly inform the design of new microbial agents aimed at pollutant degradation and environmental clean-up. Highlighting the significance of this advancing field, researchers effectively illustrate the striking alignment of magnetite crystals within cells, a phenomenon that plays a crucial role in facilitating navigation along the Earth’s magnetic field. This captivating visual representation not only succinctly encapsulates the fundamental processes at play but also underscores the promise of future research that will further elucidate the capabilities and diverse applications of these remarkable organisms. As investigations continue to uncover the complexities of microbial magnetism, we can anticipate new avenues of exploration that will intertwine biology with technology, potentially transforming various sectors including environmental science, renewable energy, and even medicine, thereby reinforcing the importance of magnetotactic bacteria in our quest for sustainable solutions and innovative applications.
At glance about the future of microbial magnetism research:
- Deciphering Genetic Mechanisms – Unraveling the genes responsible for magnetosome formation to enable genetic engineering of magnetic properties in other organisms.
- Synthetic Magnetosomes – Developing artificial magnetosomes for biomedical applications, such as targeted drug delivery and improved MRI contrast agents.
- Microbial Magnetism in Space – Studying how magnetotactic bacteria respond to microgravity and extraterrestrial environments to understand potential life beyond Earth.
- Environmental Bioremediation – Using magnetotactic bacteria to remove heavy metals and pollutants from water bodies and contaminated soil.
- Magnetically Controlled Therapeutics – Engineering bacteria with magnetosomes to deliver drugs with precise spatial control inside the human body.
- Evolutionary Insights – Investigating how magnetotactic bacteria evolved and their connection to magnetoreception in higher organisms, such as birds and fish.
- Magnetofossils in Geology – Analyzing ancient magnetosomes to reconstruct Earth’s past geomagnetic field changes and climate history.
- Biosensors for Pathogen Detection – Utilizing magnetosome-based biosensors for rapid detection of harmful bacteria, toxins, or pollutants in medical and environmental settings.
- Integration with Nanotechnology – Combining magnetotactic bacteria with nanomaterials for advanced bioengineering, energy storage, and data storage applications.
- CRISPR-Based Enhancements – Using gene-editing technologies to enhance magnetosome production and improve microbial magnetism for industrial and scientific uses.
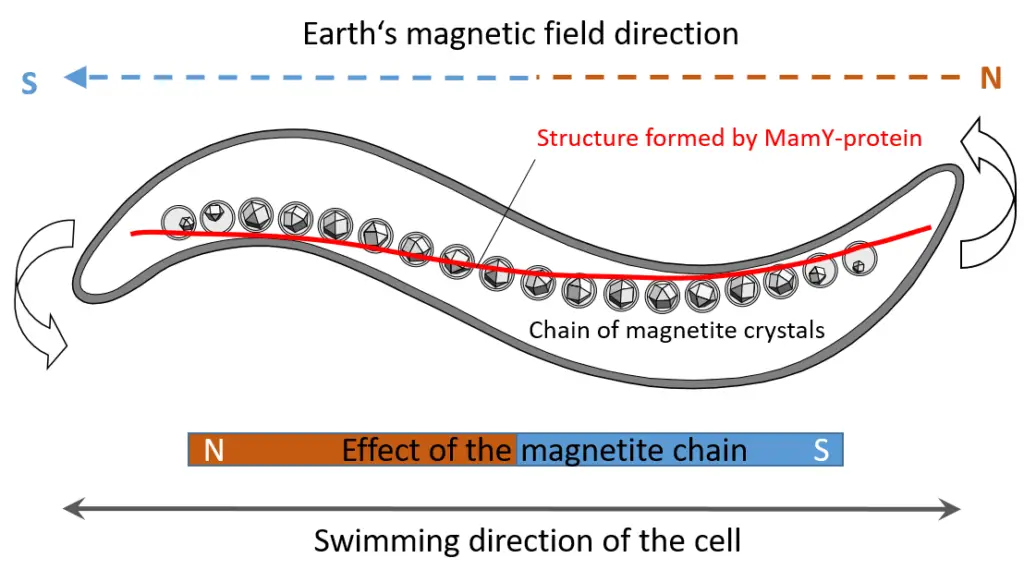
Image : Magnetic Field Orientation and Magnetite Chain in Cellular Motion (The image illustrates the structure and function of magnetite crystals within a cell, emphasizing their alignment along the Earth’s magnetic field. It features a graphical representation of a chain of magnetite crystals, showing the orientation and effect of the MamY protein in facilitating this alignment. The diagram also highlights the swimming direction of the cell, enabling a clearer understanding of how these crystals interact with the magnetic field to guide cellular movement. Notably, arrows indicate the Earth’s magnetic field orientation, contributing to the scientific context of magnetotaxis in microorganisms.)
REFERENCES
- Zach Obront. ‘The Scribe Method.’ The Best Way to Write and Publish Your Non-Fiction Book, Tucker Max, Lioncrest Publishing, 4/15/2021
- Ruiyong Zhang. ‘Insights in Microbiological Chemistry and Geomicrobiology : 2022/2023.’ Frontiers Media SA, 7/29/2024
- Shyam Kanhaiya. ‘Ecological Significance of River Ecosystems.’ Challenges and Management Strategies, Sughosh Madhav, Elsevier, 1/12/2022
- McElhinny. ‘The Earth’s Magnetic Field : Its History, Origin, and Planetary Perspective.’ Academic Press, 1/4/1984
- Stephen Lory. ‘The Prokaryotes.’ Firmicutes and Tenericutes, Edward F. DeLong, Springer Berlin Heidelberg, 10/13/2014
- Alistair McCleery. ‘An Introduction to Book History.’ David Finkelstein, Routledge, 3/13/2006
Image References:
- Image: Bacterial Magnetotaxis: Interaction with Geomagnetic Fields and Environmental Factors, Accessed: 2025.https://media.springernature.com/lw685/springer-static/image/art%3A10.1038%2Fs41522-022-00304-0/MediaObjects/41522_2022_304_Fig4_HTML.png
- Image: Electron microscopy images depicting bacterial morphology and structures., Accessed: 2025.https://media.springernature.com/full/springer-static/image/art%3A10.1038%2Fs41522-022-00304-0/MediaObjects/41522_2022_304_Fig1_HTML.png
- Image: Magnetic Field Orientation and Magnetite Chain in Cellular Motion, Accessed: 2025.https://images.zapnito.com/users/275107/posters/ad9fcaff1b816e11e5401f665a276d7e/f8eb6d36-94c5-4757-af42-6dd6e863aca8_large.png