Mass Extinctions: Causes and Evolutionary Consequences
I. Introduction
Mass extinctions are an important part of Earth’s history, where fast and big losses in biodiversity have changed ecosystems and influenced the development of life. These events, marked by a sharp decrease in many species over short geological times, prompt key questions about their causes and the long-term impacts on remaining life forms. The complex interaction of environmental factors like climate change, volcanic eruptions, and asteroid strikes are crucial to understanding these devastating events. Additionally, looking at what happens afterward tells an interesting story: the rapid development and new evolutionary paths that often happen after mass extinctions. This focus on both the causes and effects is central to discussions about mass extinctions, highlighting the strong links between environmental changes and life’s ability to adapt, which will be explained further with different case studies in the upcoming sections.
A. Definition of mass extinctions and their significance in Earth’s history
Mass extinctions are when many species suddenly disappear, causing a big drop in biodiversity within a short time, usually less than two million years. These events have changed life on Earth in major ways, affecting how species evolve and how ecosystems function. The importance of mass extinctions is not just in the immediate effects on living things but also in their long-term influence on evolution and the environment. Extreme changes, like volcanic eruptions or asteroid strikes, have pushed species to adapt. For instance, after the End-Permian and End-Cretaceous extinctions, the loss of dominant species allowed new groups to emerge. Studies show that these severe disruptions create pressures that can result in quick evolutionary changes ((Peter R Grant et al., p. 20160146-20160146)). This often leads to new species filling the gaps left by extinct ones. Additionally, the current loss of biodiversity highlights humanity’s risk amid a sixth mass extinction driven by human activities like habitat destruction, climate change, and pollution. This ongoing problem threatens many species and emphasizes the need to learn from past extinction events and recovery efforts, which is crucial for conservation and the future health of our ecosystems ((Ceballos G et al.)).
B. Overview of this article’s focus on causes and evolutionary consequences
The study of mass extinctions shows important links between changes in the environment and the paths of evolution. In Earth’s past, major extinction events were often caused by a mix of factors like climate changes, ocean acidity, and events from space. For example, new models indicate that these ecological changes can impact how species interact, where they live, and their population numbers, which can change the structure of ecosystems (Chowdhury et al.). Analyzing extinction trends gives us clues about the development of complex life, especially regarding which groups of organisms are more resilient or at risk when faced with huge environmental changes (Sepkoski et al.). By combining historical information and ecological models, this article explains not just the immediate reasons behind these disastrous events but also their lasting effects on evolution, influencing the biodiversity we see today. Knowing about these connections is vital for conservation efforts during current biodiversity challenges.
II. Causes of Mass Extinctions
Understanding why mass extinctions happen needs a detailed look at environmental shifts and biological interactions in ecosystems. For example, the relationship between growing human populations and changing climates has greatly disturbed mammal groups, causing selective extinctions, especially during times of sudden dry spells and societal breakdowns in places like ancient Egypt (Dominy et al.). These interruptions show how species vulnerability and community dynamics can be negatively affected by significant alterations, highlighting the intricate links between living and non-living factors. Additionally, approaches for managing shared resources, such as investing in public goods, reveal behavioral trends that can either lessen or increase extinction threats within species (Bowles S et al.). Ultimately, these observations show that mass extinctions are not just random occurrences but are connected to broader ecological and evolutionary processes that influence biodiversity over time.
Cause | Event | Date (Million Years Ago) | Percentage of Species Lost | Significant Impact |
Asteroid Impact | Cretaceous-Paleogene Extinction | 66 | 75 | End of Dinosaurs |
Volcanic Activity | Permian-Triassic Extinction | 252 | 96 | Largest extinction event |
Climate Change | End-Triassic Extinction | 201 | 50 | Loss of marine species |
Ocean Anoxia | Late Devonian Extinction | 375 | 70 | Significant loss in marine biodiversity |
Human Activity | Holocene Extinction | Ongoing | Up to 75% | Threat to large mammals and biodiversity |
Here’s a table summarizing the causes of mass extinction events:
Cause | Description | Examples of Mass Extinction Events |
---|---|---|
Volcanic Activity | Large-scale volcanic eruptions release massive amounts of ash, gases (like CO₂ and SO₂), and lava. This can lead to climate change, ocean acidification, and loss of biodiversity. | End-Permian Extinction (“The Great Dying”) |
Asteroid Impacts | Impact events cause widespread destruction, releasing dust and aerosols that block sunlight, leading to a “nuclear winter” effect. This disrupts photosynthesis and collapses ecosystems. | Cretaceous-Paleogene (K-Pg) Extinction |
Climate Change | Rapid changes in temperature (global warming or cooling) disrupt ecosystems. Oceanic anoxia (loss of oxygen in oceans) and acidification are common consequences. | Ordovician-Silurian Extinction, End-Permian Extinction |
Sea-Level Changes | Fluctuations in sea levels reduce habitat availability, especially in shallow marine environments, leading to biodiversity loss. | Late Devonian Extinction, Ordovician-Silurian Extinction |
Anoxia (Oxygen Depletion) | Reduction in oxygen levels in oceans causes the death of marine life, often triggered by nutrient runoff, volcanic activity, or stagnant water. | Late Devonian Extinction |
Methane Release | Release of methane hydrates from ocean sediments due to warming can cause rapid greenhouse gas increases, exacerbating global warming. | End-Permian Extinction |
Supernova or Gamma-Ray Bursts | Rare but possible cosmic events that release high-energy radiation, destroying the ozone layer and causing severe environmental damage. | Hypothetical cause for certain extinctions (not yet well-supported) |
Biological Causes | Proliferation of invasive species, diseases, or competition leading to the collapse of ecosystems. | Late Devonian Extinction |
Human Activity | Overexploitation of resources, habitat destruction, pollution, and climate change driven by anthropogenic activities. | Holocene Extinction (Ongoing) |
A. Natural events: volcanic eruptions and asteroid impacts
Natural events like volcanic eruptions and asteroid strikes play a key role in mass extinctions, acting as triggers for significant changes in ecosystems. For instance, the Cretaceous/Paleogene extinction shows how these events interact, with the Chicxulub asteroid hitting Earth around the same time as volcanic activity from the Deccan Traps. This combination of destructive events caused major environmental shifts; the asteroid impact quickly lowered global temperatures and disrupted habitats, while the following volcanic eruptions led to longer-lasting climate changes, such as rising carbon dioxide levels, which made survival even harder for species that were already in trouble. Studies suggest that the asteroid impact was the main cause of the extinction of non-avian dinosaurs, while the volcanic eruptions may have lessened some of the worst effects of the impact, underscoring the complicated ways in which such events influence evolutionary paths during mass extinctions (Allison et al.), (Fritsche et al.).
Event | Cause | Estimated Extinct Species | Time Period (Million Years Ago) |
Ordovician-Silurian Extinction | Volcanic Eruptions | 85% | 440 |
Late Devonian Extinction | Asteroid Impacts and Volcanic Activity | 75% | 375 |
Permian-Triassic Extinction | Volcanic Eruptions (Siberian Traps) | 96% | 252 |
Triassic-Jurassic Extinction | Volcanic Activity and Climate Change | 80% | 201 |
Cretaceous-Paleogene Extinction | Asteroid Impact | 76% | 66 |
Mass Extinctions Due to Natural Events
B. Climate change: shifts in temperature and sea levels
Climate change is greatly affecting ecosystems worldwide, especially with temperature changes and rising sea levels, causing major evolutionary impacts. As temperatures rise faster than before, species encounter direct physical challenges that change traits related to life history and behavior in their ecosystems. For example, temperature impacts vital development and immune function in different groups, like Odonata, which affects how well they can adapt and survive in quickly changing conditions (Adams J et al.). Additionally, past trends show that climate changes have played a key role in previous mass extinctions, hinting at a repeating connection between climate changes and loss of biodiversity (Stewart et al.). Increasing sea levels make habitat loss worse, especially in coastal areas, making it hard for species to move or adjust to new environments. This complex relationship between climate change and evolutionary challenges endangers current biodiversity and warns of a future with significant ecological disturbance.
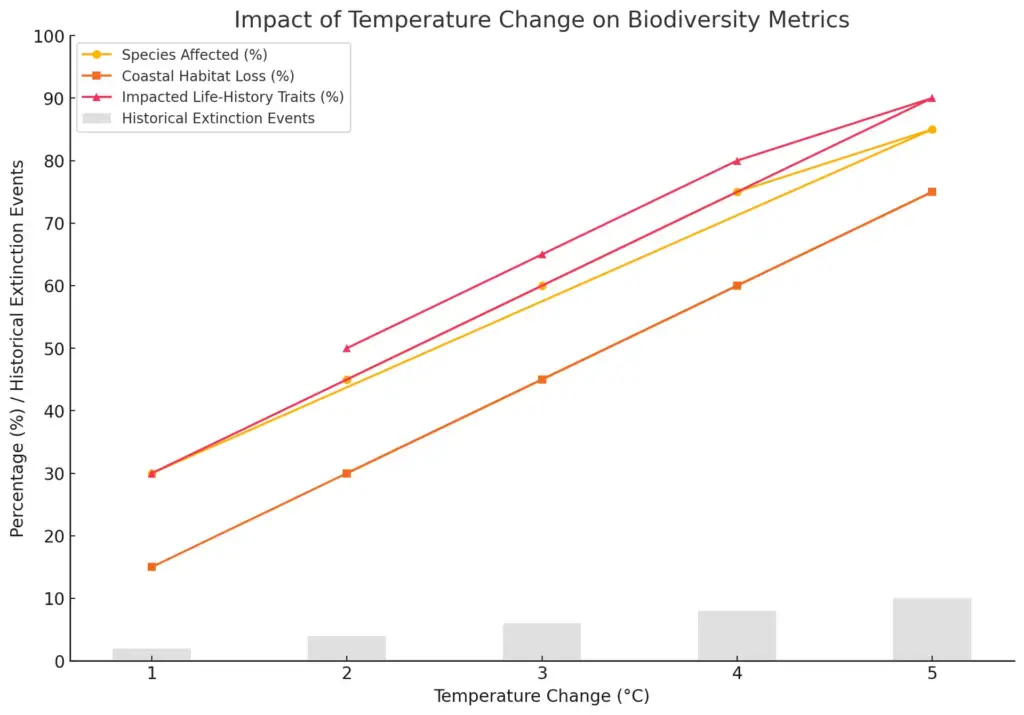
The chart illustrates the impact of temperature change on various biodiversity metrics, including the percentage of species affected, coastal habitat loss, and impacted life-history traits. It also shows the historical extinction events associated with different temperature changes.
III. Human Impact on Mass Extinctions
The effect of human actions on mass extinctions is becoming clearer, with population growth and industrialization making ecological problems worse. Historical records show that the ways human societies interact with their environments have played a crucial role in local extinction events, especially in places like Egypt, where changes in mammal populations can be tracked over 6,000 years. This time frame shows that human-related pressures like habitat loss and climate change are linked with major losses in biodiversity (Dominy et al.). Additionally, the Quaternary period is an important time when climate changes mixed with evolution, making it harder for species to adapt and survive (Stewart et al.). The combined effects of these human actions highlight the urgent need for conservation. The ongoing damage to ecosystems endangers not just current species, but also disturbs the complex web of species relationships essential for ecological balance. These findings emphasize the importance of a unified global effort to lessen human effects on a fragile biosphere.
Year | Estimated Species Lost | Causes | Key Species |
1900 | 100 | Habitat destruction, overexploitation | Passenger pigeon, heath hen |
2000 | 1 | Habitat loss, invasive species, pollution | Golden toad, Pinta Island tortoise |
2020 | 150 | Climate change, habitat degradation | Amur leopard, Yangtze giant softshell turtle |
2023 | 200 | Climate change, deforestation, agriculture | Western black rhinoceros, Javan tiger |
Human Impact on Mass Extinctions Data
A. Habitat destruction and its role in species decline
The fast rate of losing habitats is a key reason for the drop in biodiversity, which affects ecosystems worldwide. Factors like city growth, cutting down forests, and expanding farms have broken down many ecosystems, causing a significant loss in species and genetic variety. The effects of losing habitats are severe; it disturbs ecological relationships, reduces available resources, and increases the risks for many species. This situation fits with the idea of the Evil Quartet—a term by Jared Diamond that includes habitat loss along with other reasons for extinction like overhunting and invasive species. Edward O. Wilson’s acronym HIPPO, which stands for Habitat destruction, Invasive species, Pollution, Population growth, and Overharvesting, highlights the importance of habitat loss in today’s biodiversity crisis. As biodiversity keeps decreasing, the need for strong laws to tackle habitat destruction becomes more critical (Chen et al.), (Chen et al.).
Habitat Destruction in Species Decline: This table highlights various forms of habitat destruction, their causes, examples of affected habitats, impacted species, biodiversity consequences, and challenges in restoration.
Type of Habitat Destruction | Key Causes | Examples of Affected Habitats | Impacted Species | Consequences for Biodiversity | Restoration Challenges |
---|---|---|---|---|---|
Deforestation | Logging, agriculture, urban expansion | Tropical rainforests, temperate forests | Orangutans, jaguars, forest birds | Loss of forest cover reduces species diversity; disrupts carbon cycles and local climates. | Soil degradation, long recovery periods, monoculture plantations often replace native biodiversity. |
Wetland Drainage | Urbanization, agriculture, damming rivers | Marshes, swamps, mangroves | Frogs, waterbirds, estuarine fish | Loss of breeding grounds and water filtration services; disrupts aquatic food webs. | Reversing hydrological changes, controlling invasive species. |
Coral Reef Degradation | Climate change, overfishing, pollution | Coral reefs | Coral species, reef fish, sea turtles | Coral bleaching reduces reef complexity; disrupts marine biodiversity and coastal protection. | Restoring corals requires stable temperatures; slow growth rates hinder rapid recovery. |
Grassland Conversion | Agriculture, overgrazing | Prairies, savannas | Prairie dogs, bison, ground-nesting birds | Reduced plant and animal diversity; increased erosion and loss of soil fertility. | Reestablishing native grasslands is costly; competition with agricultural interests. |
Urbanization | Infrastructure development, population growth | All terrestrial and aquatic habitats | Urban-sensitive species like wolves, amphibians | Habitat fragmentation and edge effects; decline in species requiring large territories. | Creating wildlife corridors, green spaces, and limiting urban sprawl. |
Mining and Quarrying | Resource extraction, habitat removal | Mountains, riverbeds, forests | Salamanders, cave species, endemic flora | Destruction of landscapes; contamination of surrounding environments with heavy metals and sediments. | Restoring mined areas is challenging due to soil infertility and water contamination. |
Desertification | Overgrazing, climate change | Arid and semi-arid regions | Desert tortoises, xerophytic plants | Loss of productive ecosystems, reduced species adapted to specific arid environments. | Requires extensive reforestation, water management, and combating soil erosion. |
Coastal Habitat Loss | Tourism, industrialization, aquaculture | Beaches, dunes, estuaries | Shorebirds, marine invertebrates | Decline in species reliant on coastal zones for feeding and reproduction; disruption of marine-freshwater interfaces. | Restoration of coastal ecosystems is hindered by ongoing human activity and rising sea levels due to climate change. |
B. Pollution and its effects on biodiversity
Looking at pollution as an important reason for biodiversity loss shows its harmful effects, especially in mass extinction events. Pollution from chemical runoff, plastic waste, or air pollution harms ecosystems and the connections within them, weakening the stability needed for species to survive. This environmental damage is not new; past evidence links pollution-related issues to big changes in biodiversity, especially during the Quaternary period when climate change and changes in ecological communities were linked with evolution, causing many species to go extinct or adapt (Stewart et al.). Today, current dangers make the chances of a sixth mass extinction worse, particularly in biodiversity-rich places like Africa. Here, socio-economic issues connect with ecological health, indicating that global environmental changes caused by pollution threaten the survival of both species and human health (PIEVANI).
Pollution and Its Effects on Biodiversity: This table highlights the types, sources, and effects of pollution on ecosystems and biodiversity, supported by examples of real-world impacts.
Type of Pollution | Major Sources | Affected Ecosystems | Effects on Biodiversity | Examples |
---|---|---|---|---|
Air Pollution | Industrial emissions, vehicle exhaust, deforestation | Terrestrial, freshwater, marine | Acid rain damages forests; smog reduces photosynthesis; impacts respiratory health in animals | Decline in lichen diversity due to air pollution; bird deaths from smog exposure |
Water Pollution | Industrial waste, agricultural runoff, oil spills | Freshwater, marine | Eutrophication causes algal blooms, hypoxia; toxic substances harm aquatic species | Mass fish deaths due to pesticide runoff; coral bleaching from chemical contamination |
Soil Pollution | Pesticides, heavy metals, industrial spills | Terrestrial, agricultural | Reduces soil fertility; harms soil microorganisms; bioaccumulation in food chains | Decline in earthworm populations; crop toxicity impacting herbivores |
Plastic Pollution | Improper waste disposal, microplastics | Marine, freshwater | Entanglement of animals; ingestion causes starvation and internal injuries | Sea turtles ingesting plastic bags; microplastic accumulation in fish tissues |
Noise Pollution | Urbanization, industrial activity, transportation | Terrestrial, marine | Disrupts communication, mating, and navigation in animals | Marine mammals affected by ship noise; bird migration patterns altered by urban noise |
Thermal Pollution | Industrial discharges, power plants | Freshwater, marine | Alters temperature-sensitive habitats; reduces oxygen levels; affects reproduction in aquatic species | Fish kills near industrial plants due to thermal stress |
Light Pollution | Urban lighting, industrial zones | Terrestrial, marine | Disrupts nocturnal animal behavior; interferes with plant and animal reproductive cycles | Sea turtle hatchlings disoriented by artificial lights; altered flowering in plants |
Chemical Pollution | Pesticides, herbicides, industrial chemicals | Terrestrial, aquatic | Toxic bioaccumulation; endocrine disruption in wildlife; mutations in sensitive species | Decline in pollinator populations due to neonicotinoid pesticides; frog deformities in wetlands |
IV. Evolutionary Consequences of Mass Extinctions
The effects of mass extinctions on evolution are significant, impacting biodiversity and the complexity of ecosystems for a long time after the events. After major extinction events, like the Permian-Triassic or Cretaceous-Paleogene, the species that survive often diversify quickly, occupying the ecological spaces left by those that went extinct. This process encourages adaptive radiation, where species develop unique traits in response to new environmental conditions. For example, (Stewart et al.) points out how shifts in climate and changes in ecological communities during the Quaternary led to evolutionary changes that affected both the evolution of species and their extinction. Additionally, the link between species survival and their ability to colonize habitats is clear in metacommunity dynamics, as outlined in (Allhoff et al.). In this context, a species’ chance of survival is tied to its interactions in local ecosystems and its capacity to move between different areas, showing that mass extinctions can create complex evolutionary paths that alter relationships within and across communities.
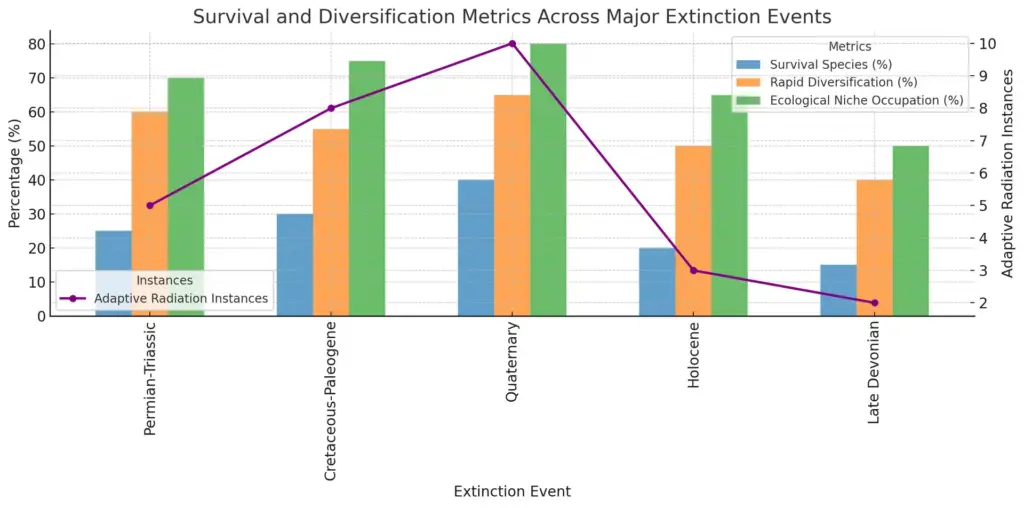
This chart illustrates the survival and diversification metrics across major extinction events. It compares the percentage of survival species, rates of rapid diversification, and ecological niche occupation, along with the instances of adaptive radiation, highlighting significant trends and differences among these events.
Here’s a supporting table summarizing the Evolutionary Consequences of Mass Extinctions: This table outlines the timing, causes, consequences, and evolutionary impacts of the five major mass extinction events, illustrating how each shaped the trajectory of life on Earth.
Mass Extinction Event | Time Period | Key Causes | Major Consequences | Evolutionary Impact |
---|---|---|---|---|
Ordovician-Silurian | ~444 million years ago | Glaciation, sea level drop | Loss of ~85% of marine species | Opened ecological niches for the evolution of jawed fish and the first terrestrial plants. |
Late Devonian | ~375-359 million years ago | Ocean anoxia, climate changes | ~75% of species lost, especially marine organisms | Delayed the diversification of fish and coral ecosystems; allowed early amphibians to gain ecological prominence. |
Permian-Triassic | ~252 million years ago | Volcanism, methane release | Known as the “Great Dying”; ~96% of marine species and ~70% of terrestrial vertebrates lost | Triggered the rise of early dinosaurs and modern insect lineages; adaptive radiation of surviving taxa due to vacated ecological roles. |
Triassic-Jurassic | ~201 million years ago | Volcanism, climate change | ~80% of species lost, including many large amphibians and marine reptiles | Enabled the dominance of dinosaurs and the rise of early mammals. |
Cretaceous-Paleogene | ~66 million years ago | Asteroid impact, volcanism | Extinction of non-avian dinosaurs, ~75% of all species lost | Facilitated the diversification of mammals and birds; flowering plants also diversified rapidly. |
A. Adaptive radiation and the emergence of new species
Adaptive radiation usually happens after mass extinction events, acting as an important way for new species to produce in open ecological roles. When main groups die out, less competition lets the remaining species quickly change, fitting into different environmental conditions. This process shows up in the fossil record as evolutionary branching, where separate lineages come from common ancestors, showing how organisms can use various resources and succeed in new habitats. These adaptive methods can result in key evolutionary species, whose loss changes whole ecological systems, as shown in recent studies on evolutionary domains of attraction (EDAs) that look into species interaction patterns ((Dieckmann et al.)). In the end, learning about adaptive radiation helps us understand how biodiversity grows and reinforces the idea that mass extinctions, although harmful, can spark evolutionary changes and strength in shifting environments ((ADRAIN et al.)).
B. Long-term ecological impacts and changes in biodiversity
The long-term effects of mass extinctions on ecology have a big impact on biodiversity, leading to major changes in species types and how ecosystems work. For example, when invasive species are removed, it can help native populations bounce back. A study showed that when barred owls, which are invasive, were taken out, the threatened northern spotted owl showed positive growth (J Wiens et al.). This example highlights the complicated relationships between different species, as the effects of one species disappearing can influence whole communities and food chains. Furthermore, the different impacts of long-term environmental changes, like those seen during the COVID-19 lockdowns, reveal the complicated links between human behavior, resource use, and species survival. The drop in pollution during lockdowns led to a short-term recovery of city ecosystems, indicating that even brief human disruptions can trigger important ecological changes (C Battisti, p. 146-150). Therefore, grasping these dynamics is essential for developing effective conservation methods to reduce biodiversity loss.
V. Conclusion
When looking at the various findings about mass extinctions, it is clear that environmental changes and how organisms respond play a crucial role in shaping life on Earth. Each mass extinction not only leads to a significant loss of biodiversity but also opens up pathways for surviving species to adapt and evolve. Mathematical models and computer simulations have improved our understanding of how species change in reaction to sudden environmental changes, as noted in (Chowdhury et al.). Additionally, investigating specific examples, like the differences in mass and brightness of young stars, highlights possible flaws in theoretical models of evolution that could affect our knowledge of species development after extinction events, as shown in (Baraffe I et al.). Therefore, researching mass extinctions is more than just an interest in history; it encourages a thorough investigation of how evolution works and what it means for conserving biodiversity in the future.
A. Summary of key points regarding causes and consequences
It is important to know why mass extinctions happen and what they lead to for understanding their big effects on evolution. Some main reasons like climate change, ocean issues, and volcanic eruptions have caused species to go extinct in the past, showing how environment changes and living things relate to each other. For example, (Stewart et al.) shows that climate change plays a twofold role in shaping ecosystems because it can both cause extinction and be a result of evolution. Moreover, (Allhoff et al.) points out that the survival behaviors of species are significant during tough environmental changes, as those that have strong local survival tactics usually endure better. All these factors highlight the complicated connections in ecosystems and the need for organisms to adapt, which in turn affects patterns of biodiversity and the rise of new species after extinction events. This detailed look at these issues shows how causes and effects are linked in learning about life’s evolution on Earth.
B. Reflection on the importance of understanding mass extinctions for future conservation efforts
Knowing about mass extinctions is important for making good conservation plans for the future because these events show how environment changes and species survival connect. By looking at past extinction trends and reasons behind them—like climate change, loss of habitats, and interactions between species—conservationists can better see and lower current dangers to biodiversity. For example, lessons learned from the Permian and Cretaceous-Paleogene extinctions show how certain groups of organisms are weak to big environmental shifts, stressing the need for specific conservation actions. Additionally, looking at past extinctions can help create forward-thinking actions to strengthen ecosystem stability, which helps species adjust to today’s climate issues. With human activities speeding up extinction rates, including knowledge from these past events into conservation policies is vital for protecting biodiversity and supporting lasting ecosystems. Therefore, understanding the effects of mass extinctions is key for not just knowing history but also saving the future of our planet’s ecological system.
Here’s a supporting table that summarizes key aspects of mass extinctions and their relevance to future conservation efforts:
Mass Extinction Event | Time Period | Primary Causes | Impact on Biodiversity | Lessons for Conservation |
---|---|---|---|---|
Ordovician-Silurian | ~445 million years ago | Glaciation, sea-level changes | ~85% of species lost, particularly marine species | Importance of monitoring climate and oceanic changes for marine conservation. |
Late Devonian | ~375-360 million years ago | Ocean anoxia, eutrophication, climate change | ~75% of species lost, especially marine life | Need to manage nutrient loading in aquatic ecosystems and address ocean deoxygenation. |
Permian-Triassic (The Great Dying) | ~252 million years ago | Volcanic eruptions, methane release, climate change | ~96% of marine species, ~70% of terrestrial vertebrates lost | Highlighting vulnerability of ecosystems to rapid and extreme climatic and chemical shifts. |
Triassic-Jurassic | ~201 million years ago | Volcanic activity, climate change | ~80% of species lost, paving the way for dinosaurs to dominate | Recognizing how rapid environmental changes can facilitate major ecological turnovers. |
Cretaceous-Paleogene (K-Pg) | ~66 million years ago | Asteroid impact, volcanic activity, climate change | ~76% of species lost, including non-avian dinosaurs | Need for robust responses to sudden, catastrophic events that disrupt ecosystems globally. |
REFERENCES
- Chowdhury, Debashish, Stauffer, Dietrich. “Evolutionary ecology in-silico: Does mathematical modelling help in understanding the “generic” trends?”. 2005, https://core.ac.uk/download/pdf/291500966.pdf
- Sepkoski, J. John, Jr.. “The fossil record of evolution: Data on diversification and extinction”. 2025, https://core.ac.uk/download/pdf/42820290.pdf
- Baraffe I., Basri G., Delfosse X., Gibor Basri, Mohanty S., Potter D., Ray Jayawardhana, et al.. “Measuring Fundamental Parameters of Substellar Objects. II: Masses and Radii”. ‘University of Chicago Press’, 2004, http://arxiv.org/abs/astro-ph/0403269
- Dominy, Nathaniel J., Gross, Thilo, Guimarães Jr., Paulo R., Koch, et al.. “Collapse of an ecological network in Ancient Egypt”. ‘Proceedings of the National Academy of Sciences’, 2014, https://core.ac.uk/download/33131266.pdf
- Stewart, John R.. “Understanding evolutionary processes during past Quaternary climatic cycles: Can it be applied to the future?”. Centre for Ecology & Hydrology, INBO, IMEDA, CSIC-UIB, 2010, https://core.ac.uk/download/4898204.pdf
- ADRAIN, ANG, BAKER, BAMBACH, BENTON, BONNER, BRIGGS, et al.. “Declining Volatility, a General Property of Disparate Systems: From Fossils, to Stocks, to the Stars”. ‘Wiley’, 2013, http://arxiv.org/abs/1206.1824
- Dieckmann, U., Johansson, J.. “Evolutionary responses of communities to extinctions”. IR-09-075, 2009, https://core.ac.uk/download/33900548.pdf
- PIEVANI. “Ecology and justice in Africa”. DiSLL – Università degli studi di Padova, 2019, https://core.ac.uk/download/287854629.pdf
- Allhoff, Korinna T., Drossel, Barbara, Jones, David, Rogge, et al.. “Interplay of spatial dynamics and local adaptation shapes species lifetime distributions and species-area relationships”. ‘Springer Science and Business Media LLC’, 2018, http://arxiv.org/abs/1804.07110
- Bowles S, Gigerenzer G, Hamilton WD, Juan F. Poyatos, Kagel JH, Levin SA, Matteo Cavaliere, et al.. “Plasticity facilitates sustainable growth in the commons”. 2012, https://core.ac.uk/download/43712603.pdf
- C. Battisti. “Not only jackals in the cities and dolphins in the harbours: less optimism and more systems thinking is needed to understand the long-term effects of the COVID-19 lockdown”. Biodiversity, 2021, https://www.semanticscholar.org/paper/9b574fefbc0e50cb27f496f300627a4ece2bc0f7
- J. Wiens, Katie M. Dugger, J. M. Higley, Damon B. Lesmeister, A. Franklin, Keith A. Hamm, G. White, et al.. “Invader removal triggers competitive release in a threatened avian predator”. Proceedings of the National Academy of Sciences of the United States of America, 2021, https://www.semanticscholar.org/paper/82cdbddfd570493a4631c9dd4fbb7474fc9891d2
- Chen, James M.. “The Fragile Menagerie: Biodiversity Loss, Climate Change, and the Law”. Digital Repository @ Maurer Law, 2018, https://core.ac.uk/download/232679507.pdf
- Chen, Jim. “Across the Apocalypse on Horseback: Imperfect Legal Responses to Biodiversity Loss”. Washington University Open Scholarship, 2005, https://core.ac.uk/download/233188153.pdf
- Allison, P, Chiarenza, AA, Farnsworth, A, Lunt, et al.. “Asteroid impact, not volcanism, caused the end-Cretaceous dinosaur extinction”. ‘Proceedings of the National Academy of Sciences’, 2020, https://core.ac.uk/download/323206426.pdf
- Fritsche, Thomas. “The Impact at the Cretaceous/Tertiary Boundary”. DigitalCommons@Cedarville, 2020, https://core.ac.uk/download/351114185.pdf
- Gerardo Ceballos, Paul R. Ehrlich, Rodolfo Dirzo. “Biological annihilation via the ongoing sixth mass extinction signaled by vertebrate population losses and declines”. Proceedings of the National Academy of Sciences, 2017, https://doi.org/10.1073/pnas.1704949114
- Peter R. Grant, B. Rosemary Grant, Raymond B. Huey, Marc T. J. Johnson, Andrew H. Knoll, Johanna Schmitt. “Evolution caused by extreme events”. Philosophical Transactions of the Royal Society B Biological Sciences, 2017, https://doi.org/10.1098/rstb.2016.0146
- Adams J., Alcamo J., Angelibert S., Aoki T., Arendt J. D., Askew R. R., Atkinson D., et al.. “The impacts of environmental warming on Odonata: a review”. ‘Informa UK Limited’, 2008, https://core.ac.uk/download/13505024.pdf