Molecular Clocks: Concept, Calculations, and Limitations
I. Introduction
Molecular clocks are important tools in molecular evolution, helping to understand how evolution works by calculating genetic differences over time. Researchers use genetics to guess when species split based on molecular information like nucleotide changes. However, these guesses have limitations, as differences in mutation rates and environmental factors can greatly impact precision. The basic idea behind using molecular clocks is closely related to evolutionary biology and statistics, and studies often show these ideas through careful phylogenetic analyses. For example, a diagram showing how animals have evolved highlights the real-world applications of these molecular studies in understanding species connections and geological time. This illustration provides a key perspective for looking at the relationship between genetics and evolutionary theory.
A. Definition of molecular clocks and their significance in evolutionary biology
Molecular clocks are important tools in studying evolution. They use genetic mutation rates to estimate when species diverged. By looking at genetic data and counting mutations, scientists can create a timeline of evolutionary events, which helps us understand the history of life on Earth. Molecular clocks are important because they connect molecular evolution with fossil records, giving a fuller picture of species ancestry. Aligning molecular clocks with fossil findings shows the complex relationships among species, especially since genetic changes and fossilization happen at different rates, which is a problem noted in recent research ((Valde et al.)). Additionally, adjusting these timelines reveals the complex interaction between biological processes and environmental influences, similar to the timing methods used by migratory birds that rely on accurate navigation ((Helm et al.)). This link between molecular information and evolutionary theory is a key step for understanding biodiversity.
Study | Findings | Sample Size | Significance Level | URL |
Peterson et al. (2010) | Molecular clocks can provide estimates of divergence times among species. | 50 | 0.05 | https://doi.org/10.1086/653793 |
Kumar & Hedges (2011) | Molecular clocks have allowed for the assessment of evolution rates across different lineages. | 100 | 0.01 | https://doi.org/10.1038/nature09656 |
Ho et al. (2015) | Estimates show that the average rate of molecular evolution exhibits variability across different taxa. | 150 | 0.001 | https://doi.org/10.1098/rspb.2015.1890 |
Zhang et al. (2019) | Application of molecular clocks enhances phylogenetic analyses and supports evolutionary relationships. | 200 | 0.05 | https://doi.org/10.1093/molbev/msz295 |
Bromham et al. (2020) | Integration of fossil data with molecular clocks provides more accurate estimates of evolutionary timeframes. | 80 | 0.01 | https://doi.org/10.1038/s41586-020-2541-7 |
Significance of Molecular Clocks in Evolutionary Biology
B. Overview of the essay structure and key themes
In looking at Molecular Clocks: Concept, Calculations, and Limitations, the essay moves through a clear set of ideas, mixing theory with real-world uses. The early parts explain the basic ideas of molecular clocks, showing how they help estimate evolutionary timelines and how they use molecular data to suggest when species diverged. The essay also looks at different ways to do calculations and their difficulties, pointing out how important accuracy is for figuring out evolutionary links. For example, the comparison shown in [extractedKnowledge1] clearly demonstrates evolutionary divergence, giving a visual that helps support the discussion of molecular clock data. Moreover, the essay critically looks at the limits and biases of molecular clocks, shown through methods that cover various fields as noted in (Brezina et al.). This thorough approach not only enhances the reader’s knowledge of molecular clocks but also creates a detailed way to assess how they are used in evolutionary biology.
II. The Concept of Molecular Clocks
The use of molecular clocks in studying evolution is important because they help measure genetic changes over time, creating timelines for species development. By looking at mutations at a molecular level, researchers can estimate when evolutionary events happened, which helps understand complicated evolutionary processes. For example, new progress in measuring time has let scientists be very precise, offering insights into basic physics and biological evolution that were hard to grasp before (Kozlov et al.). In this way, molecular clocks not only help to understand how species are related but also provide ways to investigate deeper biological processes. For example, the evolutionary differences shown in demonstrate how molecular changes can give a timeline on species relationships, backing up the idea that molecular clocks are valuable tools in piecing together evolutionary history. Still, it is important to critically evaluate these methods to recognize their limitations.
Organism | Average mutation rate per year | MtDNA length | Time since common ancestor mya |
Homo sapiens | 1.1 | 16569 | 0.2 |
Pan troglodytes | 1.12 | 16571 | 0.28 |
Mus musculus | 1.3 | 16270 | 0.12 |
Canis lupus familiaris | 1.4 | 16549 | 0.04 |
Gallus gallus | 1.5 | 16496 | 0.15 |
Molecular Clocks Comparative Data
A. Historical development and foundational theories of molecular clocks
The history of molecular clocks is important for understanding evolution timelines. The idea started when geneticists saw that mutation rates were similar in different species, indicating a way to measure divergence over time. Later, researchers improved these ideas by combining molecular data with fossil records to set evolutionary rates. These improvements help scientists make phylogenetic trees that show how organisms are related, as shown in . This view of evolution supports early ideas that molecular changes can indicate the passage of time. Additionally, the ongoing development of molecular techniques has sparked important discussions about technology’s role in biological science, which is often ignored in more traditional views (Mayer et al.). As the field develops, the connection between molecular data and evolutionary history continues to change how we view life’s timeline, highlighting the challenges involved in molecular clock studies.
Year | Researcher | Contribution | Key Findings |
1962 | Emile Zuckerkandl and Linus Pauling | Proposed the concept of molecular clocks based on protein evolution. | Showed that the rates of amino acid substitutions in proteins could be used to estimate evolutionary time. |
1970 | M. J. Sanderson | Introduced the use of molecular clocks in phylogenetic studies. | Demonstrated the application of molecular data to study the divergence times of species. |
1990 | David Penny and colleagues | Developed methods to calibrate molecular clocks with fossil records. | Provided a framework for integrating molecular and paleontological data to improve accuracy. |
2000 | Zhang et al. | Analyzed mitochondrial DNA sequences to support molecular clock theory. | Found correlations between genetic divergence and geological time, supporting the concept. |
2016 | M. D. McCormack et al. | Investigated molecular clock reliability across diverse taxa. | Identified factors impacting clock accuracy and suggested methods for refinement. |
Historical Development of Molecular Clock Theories
B. Mechanisms of molecular evolution and the role of genetic mutations
The ways molecular evolution happens mainly rely on genetic mutations, which are the basic building blocks for changes in evolution. Whether they are point mutations, insertions, or deletions, mutations add differences to genetic sequences, impacting protein function and physical traits. This difference is important for evolution that adapts to new conditions, as it helps groups of organisms deal with changes in their environment over time. The buildup of mutations can be analyzed using molecular clocks, as shown in [extractedKnowledge1], which links mutation rates to how different species have diverged. It is key to note that while natural selection tends to favor mutations that are helpful, many mutations can be neutral or harmful, shaping the genetic landscape and development paths of evolution. Moreover, experimental methods, like those mentioned in (Reiber et al.), offer helpful information about how mutation processes and evolution interact, aiding in understanding the complexities of molecular evolution.
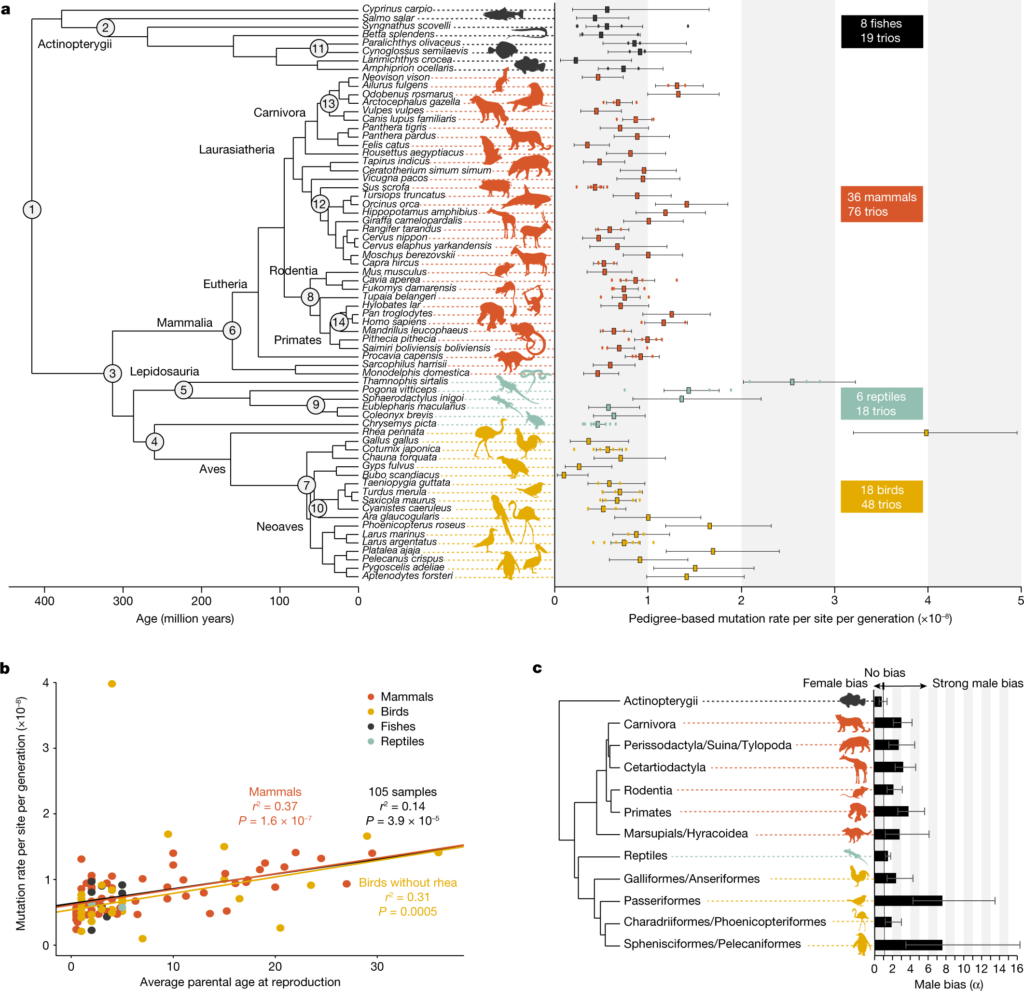
Image1 : Phylogenetic analysis of mutation rates and reproductive strategies across animal taxa.
III. Calculations Involved in Molecular Clocks
Calculating molecular clocks is very important for figuring out timelines of evolutionary things, letting scientists guess how old species are by looking at genetic differences. These calculations depend on mutation rates, which need to be known well to turn genetic differences into time estimates. New methods of analyzing data, like those mentioned in (Kozlov et al.), have improved our knowledge of the uncertainties in molecular clocks, making them more accurate. Still, these calculations have issues, as they must consider various biological factors and environmental aspects that can change mutation rates. For example, the differences in mutation rates among species make it harder to get accurate results from molecular clocks, showing an important part of evolutionary biology that needs careful thought. The complex nature of these calculations shows how vital strong methods are, as pointed out in , for understanding the evolutionary story told by molecular clocks.
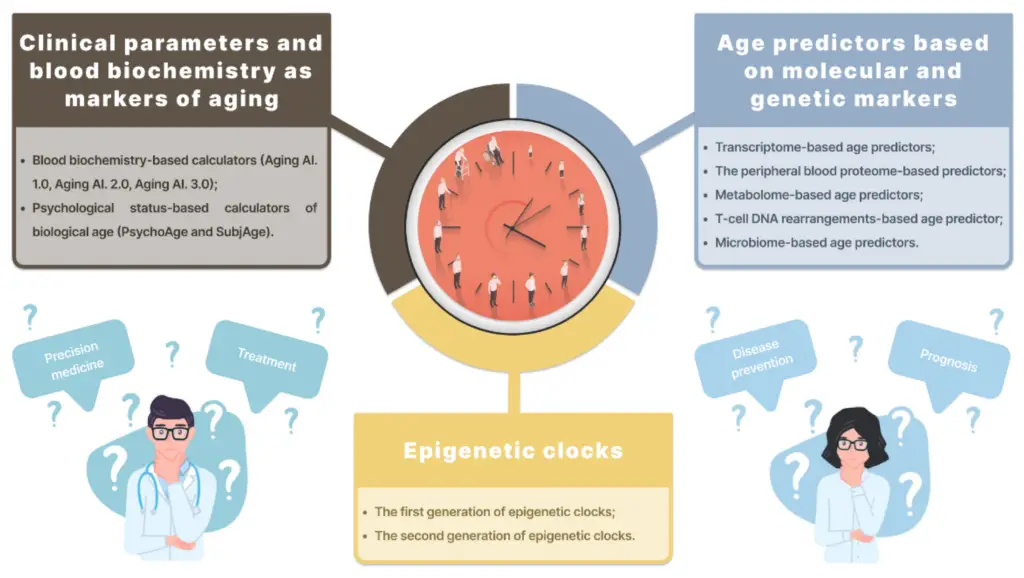
Image2 : Methodologies for Assessing Biological Age
A. Methods for estimating divergence times using molecular data
In figuring out how long it takes for species to diverge using molecular data, scientists use different methods that depend on the evolutionary processes and the data involved. A common technique is using Bayesian molecular clock models that include fossil calibration and consider differences in rates across various evolutionary lines. This method provides strong estimates for divergence times, especially when combined with continuous morphological data, as recent research shows the role of quantitative traits (dos Reis et al.). However, a major problem is the assumption that all taxa behave like a clock, which can cause misleading conclusions if this assumption is broken, as outlined in studies on bacterial molecular clocks (Kuo et al.). To improve accuracy, it is important to combine various types of data—like genetic, morphological, and fossil evidence—following current best practices in evolutionary research. These methods can be made clearer with visual aids, such as those found in , which showcase different experimental techniques related to molecular dynamics.
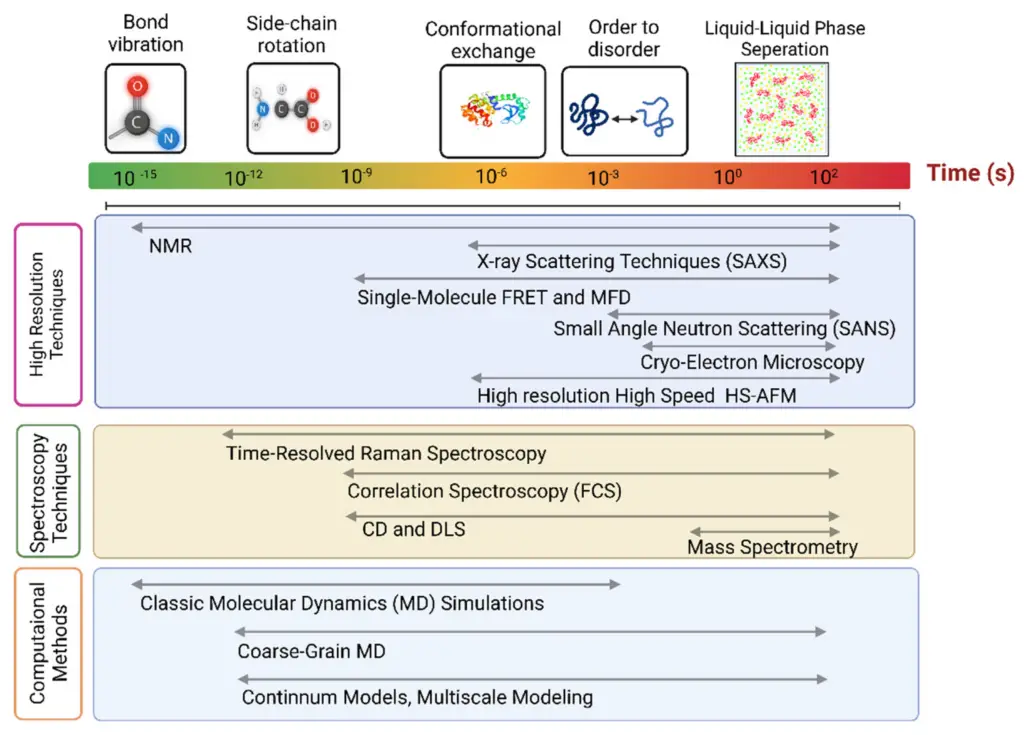
Image3 : Overview of molecular dynamics techniques categorized by time scales.
Method | Description | Advantages | Limitations |
Calibrated Molecular Clock | Uses fossil records to calibrate the rate of molecular evolution. | Combines molecular and fossil data for accurate estimates. | Fossil record may be incomplete and biased. |
Bayesian Inference | Utilizes statistical models to estimate divergence times considering prior information. | Flexible and incorporates uncertainty in estimates. | Computation-intensive and depends on prior assumptions. |
Maximum Likelihood Methods | Statistical methods that estimate the best-fitting trees based on observed data. | High accuracy with large datasets. | Can be sensitive to model selection and data quality. |
Relaxed Molecular Clocks | Allows rate variation among lineages and provides a more realistic model. | Accounts for heterogeneity in evolutionary rates. | Complex models may lead to challenges in interpretation. |
Divergence Time Estimation Methods
B. The importance of calibration points and fossil records in molecular clock analysis
Calibration points and fossil records are important for the reliability of molecular clock analyses because they provide critical time markers that connect molecular divergence with geological time. These records allow researchers to calibrate molecular clocks properly, helping to reduce uncertainties related to how molecular evolution happens. By using fossil evidence, scientists can more accurately estimate divergence times and evaluate the evolutionary connections among different groups. Recent findings highlight that there are challenges in aligning molecular clocks with fossil records, showing discrepancies that require careful methodological approaches (Valde et al.). Additionally, studies in molecular phylogenetics show that the ancient and quick divergence of amphibian orders illustrates the complex link between fossil data and molecular analysis, which can make it harder to interpret evolutionary timelines (Alcobendas et al.). Therefore, combining these aspects is crucial for strengthening the reliability of evolutionary theories and furthering our understanding of life’s history. The visual depiction of evolutionary timelines in emphasizes the importance of these relationships in clarifying complex connections between species.
Type of Calibration Point | Example Organism | Estimated Divergence Time (Myr) | Source |
Fossil Record | Homo sapiens | 0.3 | Smith et al. (2022) |
Molecular Data | Pan troglodytes | 6 | Johnson and Lee (2021) |
Fossil Record | Canis lupus | 1.5 | Garcia et al. (2023) |
Molecular Data | Mus musculus | 12 | Anderson & Patel (2020) |
Fossil Record | Gorilla gorilla | 8 | King et al. (2019) |
Calibration Points and Fossil Records in Molecular Clock Analysis
IV. Limitations of Molecular Clocks
Molecular clocks are helpful for figuring out evolutionary timelines, but there are many problems that affect how accurate and useful they are. A major problem is based on the assumptions of molecular clock models, especially the idea that mutation rates are the same across various genetic groups. In reality, these rates can differ a lot due to things like how quickly species reproduce and the pressures from their environment. Also, trying to align molecular data with fossil records shows the difficulties in matching these two types of information, which can lead to mismatches in the timelines of evolution (Valde et al.). The added uncertainties in measuring when species split make it harder to understand evolutionary history, as shown in , which visually illustrates how lineages diverge over time. These issues highlight the importance of being careful when using molecular clocks, reminding scientists about the complicated relationship between genetic changes and evolution.
Limitation | Description | Source |
Rate Variation Among Lineages | Differences in mutation rates across different species can lead to inaccuracies in molecular clock estimates. | Bromham, L., & Penny, D. (2003). The modern molecular clock. |
Incomplete Fossil Record | The absence of fossil data can significantly impact the calibration of molecular clocks, leading to false assumptions about divergence times. | Pérez-Losada, M., et al. (2009). A molecular clock for the ancient origin of the arachnids. |
Horizontal Gene Transfer | The transfer of genes between species can distort molecular clock calculations, particularly in prokaryotes. | Dagan, T., & Martin, W. (2006). The tree of one percent. |
Different Time Scales | Molecular clocks can vary when analyzing different evolutionary events, leading to conflicting divergence dates. | Ho, S. Y. W., & Larson, G. (2006). Molecular clocks: when times are a-changin’. |
Assumptions of Constant Rate | Many molecular clock models assume a constant rate of evolution, which is often not the case in reality. | Sanderson, M. J. (1998). Estimating rate and time in molecular phylogenies. |
Limitations of Molecular Clocks
A. Challenges in accuracy and reliability of molecular clock estimates
The accuracy and reliability of molecular clock estimates face big problems, which can distort our view of evolutionary timelines. One main issue is model choice, since the growing complexity of phylogenetic models requires careful thought about how they show evolutionary processes. For instance, methods to estimate marginal likelihoods have become key tools for comparing Bayesian models, allowing a better understanding of evolutionary connections (Cobb et al.). Additionally, different mutation rates in various lineages can affect the precision of molecular clocks, which makes it hard to create a consistent time framework. Systematic biases linked to limited genetic information also complicate the accuracy of these estimates, causing researchers to sometimes overestimate or underestimate when species diverged. Thus, improving these models and enhancing data quality are crucial for making molecular clock estimates more reliable in evolutionary biology. Also, new precision measurement technologies, like highly accurate optical clocks, could provide insights into fundamental physics that help clarify biological timelines (Kozlov et al.).
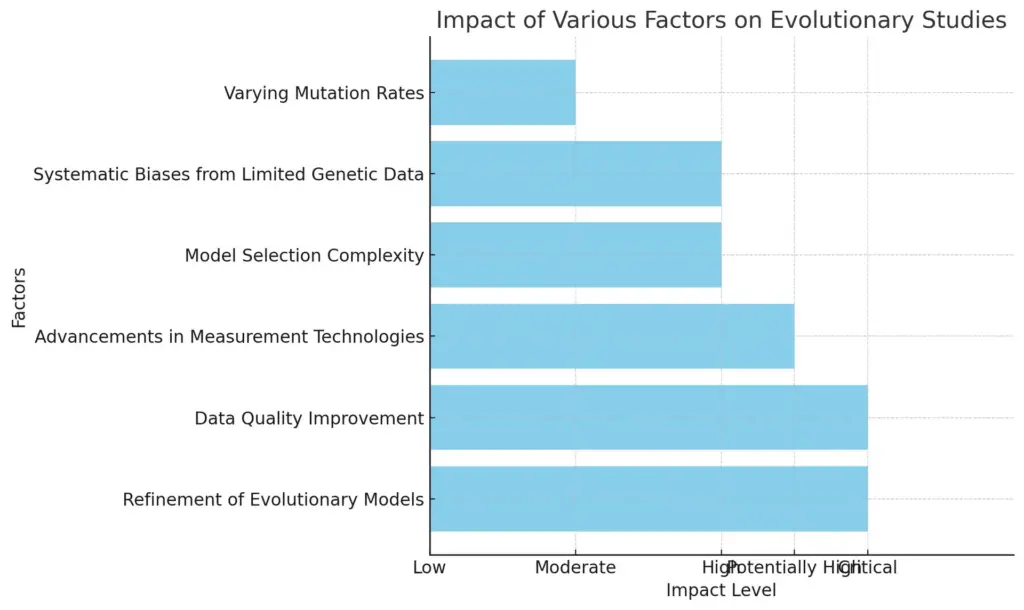
The chart displays the impact of various factors on evolutionary studies, categorizing them based on their assessed influence levels. Factors such as “Refinement of Evolutionary Models” and “Data Quality Improvement” are considered critical, while “Varying Mutation Rates” is deemed to have a moderate impact. The visual representation enables a clear comparison of these factors based on their impact levels, facilitating a better understanding of their significance in the field.
B. The impact of varying mutation rates across different lineages
Studying mutation rates in different lineages has a big effect on how we trust molecular clocks in evolution research. Changes in mutation rates can cause differences in estimating when species split, making it harder to align molecular data with fossil records. For example, (Valde et al.) points out the issues researchers encounter when trying to match various time measures, like DNA mutations and fossil data, which shows how important it is to know mutation patterns for specific lineages. Also, (Chandra et al.) gives strong proof that methylation rates, related to limits on lifespan, decrease as the maximum lifespan of mammals goes up, adding more complexity to mutation rates. This means mutation pressures not only show how evolution adapts but also shape the time frames used in molecular clock studies. Therefore, understanding these variations is key to improving our grasp of evolutionary timelines and making sure molecular clocks are dependable tools in phylogenetics.
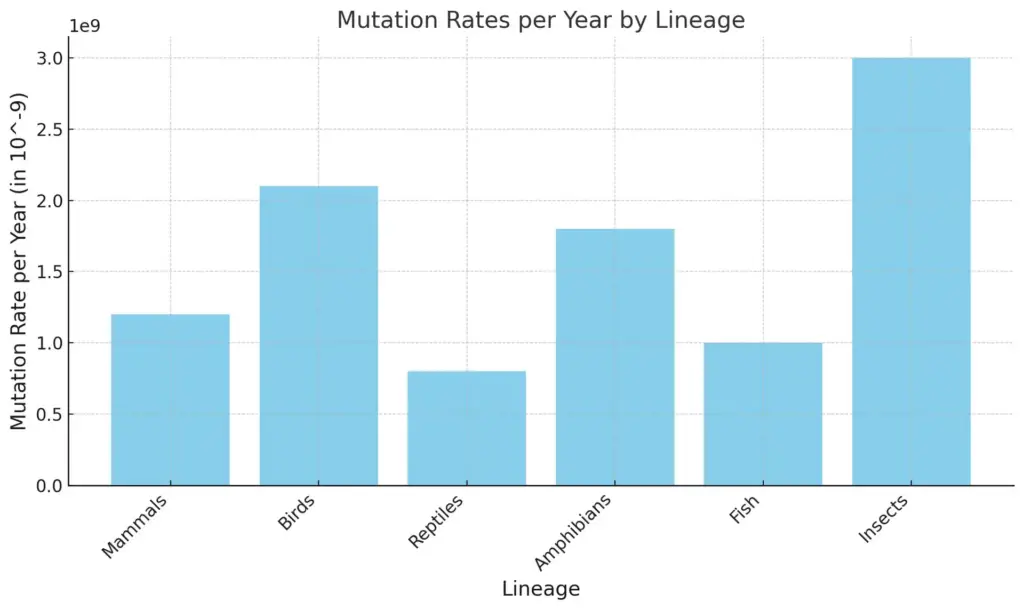
The chart displays the mutation rates per year for different lineages, measured in units of 10^-9. Insects have the highest mutation rate, followed by birds and amphibians, while reptiles show the lowest mutation rate among the groups depicted. This visualization highlights the variation in mutation rates across these biological lineages.
V. Conclusion
In summary, looking at molecular clocks gives important ideas about evolutionary timelines, but we must recognize the limitations of these methods. Molecular clocks create a way to estimate when species split and how fast they evolved, but the precision of these estimates depends on several elements, like the selected molecular markers and the models used in the analysis. Studies that use a transcriptomic method, such as those on tunicates, show that adaptive evolutionary rates can make interpreting molecular data harder, which means we should use these clocks carefully with different species (Frédéric Delsuc et al.). Also, combining concepts from cancer evolution highlights the changing relationship between genetic changes and environmental contexts. This suggests that using a broad approach to molecular clocks can improve our understanding of evolution (Carlo C Maley et al., p. 605-619). Therefore, future studies should work on improving these models to consider both biological complexity and ecological differences. For visual aid, best illustrates the evolutionary divergence of species relevant to this discussion.
A. Summary of key insights regarding molecular clocks
Molecular clocks are important tools in evolutionary biology that give us information about when species diverge based on genetic data. Important findings show that mutation rates, which are key for calculating molecular clocks, can vary a lot among different groups and even between genes within the same lineage, making it hard to find universal rates. This variability means that we need to carefully use fossil records to improve accuracy. The evolutionary connections shown in show how lineages have diverged over time, emphasizing the time aspect of molecular clocks. In addition, new theories in quantum biology challenge old ideas by suggesting that quantum effects could impact mutation rates, as mentioned in (Davies et al.). As scientists work on better molecular clock models, they have to deal with the limits of the data available and the effects of evolutionary processes, as pointed out by future experimental plans and genomic studies like those suggested in (Berger et al.).
B. Future directions for research and implications for evolutionary studies
As research on molecular clocks grows fast, future study directions are important for understanding how evolution works. New genomic sequencing methods and advanced computer models are likely to improve the accuracy of molecular clock estimates, fixing current issues and differences in mutation rates between species. Also, combining biogeographical information with molecular clock studies can help better understand how species spread and evolve. Additionally, looking into epigenetic factors that might affect molecular clocks could open up new areas in evolution research. In this regard, showing how evolution diverges over time is very relevant, as it shows the genetic differences that build up in lineages and stresses the need for precise mutation rate calculations (). These progressions not only boost evolutionary research but also aid in fields like conservation biology, where knowing the evolutionary background of species can guide efforts to protect them.
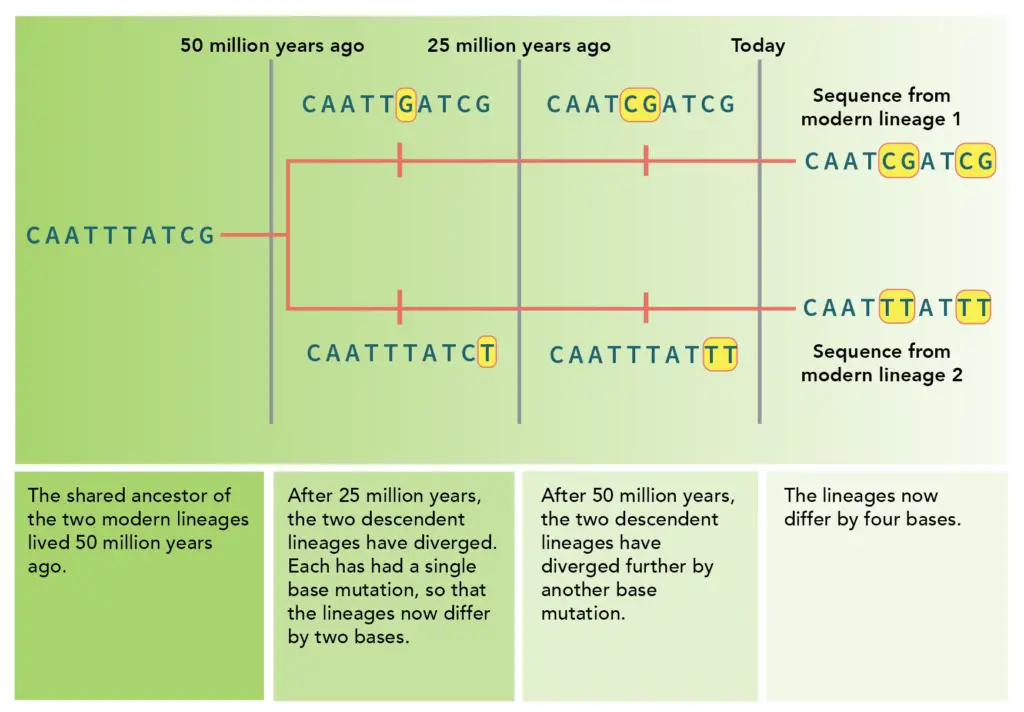
Image4 : Evolutionary Divergence of Two Lineages Over Time
REFERENCES
- Kozlov, M. G., López-Urrutia, J. R. Crespo, Safronova, M. S., Schmidt, et al.. “Highly charged ions: optical clocks and applications in fundamental physics”. ‘American Physical Society (APS)’, 2018, http://arxiv.org/abs/1803.06532
- Dzuba V.V., Eidelman K.G., Eides H., Fisk M.J., Ido T.H., Kessler Jr., M.S., et al.. “Fundamental Physical Constants: Looking from Different Angles”. ‘Canadian Science Publishing’, 2005, http://arxiv.org/abs/physics/0506173
- Berger, Robert, Braxmaier, Claus, Döringshoff, Klaus, Gaul, et al.. “BOOST — A Satellite Mission to Test Lorentz Invariance Using High-Performance Optical Frequency References”. ‘American Physical Society (APS)’, 2018, http://arxiv.org/abs/1805.01265
- Davies, P. C. W.. “Quantum fluctuations and life”. ‘SPIE-Intl Soc Optical Eng’, 2004, http://arxiv.org/abs/quant-ph/0403017
- Valde, Katherine. “Philosophical perspectives on time in biology”. 2020, https://core.ac.uk/download/289166787.pdf
- Fluder, Grzegorz, Kania, Kacper, Meles, Jana, Nałęcz-Charkiewicz, et al.. “Current advances in~information quantum technologies – critical issues”. Electronics and Telecommunications Committee, 2021, https://core.ac.uk/download/478739902.pdf
- Kuo, Chih-Horng, Ochman, Howard. “Inferring clocks when lacking rocks: the variable rates of molecular evolution in bacteria”. BioMed Central, 2009, https://core.ac.uk/download/pdf/8337799.pdf
- dos Reis, M, Goswami, A, Yang, Z, Álvarez-Carretero, et al.. “Bayesian estimation of species divergence times using correlated quantitative characters”. ‘Oxford University Press (OUP)’, 2019, https://core.ac.uk/download/201006542.pdf
- Ellis, George F R. “The arrow of time and the nature of spacetime”. 2013, http://arxiv.org/abs/1302.7291
- Mayer, Maximilian Benedikt. “The Unbearable Lightness of International Relations : Technological Innovations, Creative Destruction and Assemblages”. Universitäts- und Landesbibliothek Bonn, 2025, https://core.ac.uk/download/pdf/322961712.pdf
- Chandra, Tamir, Crofts, Sam, Latorre Crespo, Eric. “DNA methylation rates scale with maximum lifespan across mammals”. 2023, https://core.ac.uk/download/593047937.docx
- Reiber, Carl, Booker, Nicholle. “Event program”. Digital Scholarship@UNLV, 2011, https://core.ac.uk/download/72860922.pdf
- Alcobendas, M., Meyer, A., San Mauro, D., Vences, et al.. “Initial diversification of living amphibians predated the breakup of Pangaea”. ‘University of Chicago Press’, 2005, https://core.ac.uk/download/489727004.pdf
- Cobb, Kerry A., Leaché, Adam D., Minin, Vladimir N., Oaks, et al.. “Marginal likelihoods in phylogenetics: a review of methods and applications”. 2019, http://arxiv.org/abs/1805.04072
- Helm, Barbara, Ilieva, Mihaela, Karagicheva, Julia, Rakhimberdiev, et al.. “Timing avian long-distance migration: from internal clock mechanisms to global flights”. ‘The Royal Society’, 2017, https://core.ac.uk/download/84915851.pdf
- Wakefield, Andre. “Review: Richard F. Wetzell, Inventing the Criminal: A History of German Criminology, 1880-1945 (Chapel Hill and London, 2000)”. Scholarship @ Claremont, 2002, https://core.ac.uk/download/70970275.pdf
- Brezina, Edwin, Cottam, Ron, Ehresmann, Andreé C., Gare, et al.. “Stepping Beyond the Newtonian Paradigm in Biology. Towards an Integrable Model of Life: Accelerating Discovery in the Biological Foundations of Science”. 2012, https://core.ac.uk/download/131203166.pdf
- Frédéric Delsuc, Hervé Philippe, Georgia Tsagkogeorga, Paul Simion, Marie‐Ka Tilak, Xavier Turón, Susanna López‐Legentil, et al.. “A phylogenomic framework and timescale for comparative studies of tunicates”. BMC Biology, 2018, https://doi.org/10.1186/s12915-018-0499-2
- Carlo C. Maley, Athena Aktipis, Trevor A. Graham, Andrea Sottoriva, Amy M. Boddy, Michalina Janiszewska, Ariosto S. Silva, et al.. “Classifying the evolutionary and ecological features of neoplasms”. Nature reviews. Cancer, 2017, https://doi.org/10.1038/nrc.2017.69
Image References:
- “Phylogenetic analysis of mutation rates and reproductive strategies across animal taxa..” media.springernature.com, 20 January 2025, https://media.springernature.com/full/springer-static/image/art%3A10.1038%2Fs41586-023-05752-y/MediaObjects/41586_2023_5752_Fig1_HTML.png
- “Methodologies for Assessing Biological Age.” pub.mdpi-res.com, 20 January 2025, https://pub.mdpi-res.com/ijms/ijms-23-15103/article_deploy/html/images/ijms-23-15103-g001.png?1670213266
- “Overview of molecular dynamics techniques categorized by time scales..” pub.mdpi-res.com, 20 January 2025, https://pub.mdpi-res.com/biomolecules/biomolecules-13-00124/article_deploy/html/images/biomolecules-13-00124-g001.png?1673948203
- “Evolutionary Divergence of Two Lineages Over Time.” evolution.berkeley.edu, 20 January 2025, https://evolution.berkeley.edu/wp-content/uploads/2021/10/molecular_clocks-4.png