Pathogenic Bacteria: Types, Transmission, and How They Cause Disease
Table of Contents
I. Introduction to Bacterial Pathogenicity
Bacterial pathogenicity is a crucial area of study within microbiology that explores the complex mechanisms by which bacteria cause disease in their hosts. This field of research is essential because it helps scientists unravel the specific biological processes involved in infection. Understanding these mechanisms entails examining various factors that contribute to pathogenicity, including the bacterium’s ability to adhere to host tissues, evading the immune response, and acquiring essential nutrients necessary for survival and proliferation. For instance, the image depicting the infection process of Shigella effectively illustrates these mechanisms by showcasing how this pathogen utilizes a sophisticated type III secretion system to inject virulence factors directly into host cells. This process leads to invasion of the host’s cellular environment and triggers a cascade of events that culminate in disease development. Such diagrams not only highlight the intricate interactions between bacteria and host cells but also reveal how specific bacterial strains can induce varying disease outcomes depending on their unique virulence attributes. Consequently, probing bacterial pathogenicity is not solely about understanding how infections occur; it also elucidates the underlying biological processes that give rise to infectious diseases in humans. This knowledge is pivotal as it fosters the development of targeted therapeutic strategies, which could significantly enhance treatment options for bacterial infections, as well as inform effective public health interventions aimed at controlling outbreaks. Through this exploration of bacterial pathogenicity, researchers can better comprehend the multifaceted nature of bacterial infections and their wider implications for human health, paving the way for new insights into disease prevention and management.
Pathogen | Type | Transmission | Diseases | Prevalence (% of infections) |
Escherichia coli (E. coli) | Enteric pathogen | Contaminated food/water | Gastroenteritis, Urinary tract infections | 10-15% |
Streptococcus pneumoniae | Bacterial pneumonia | Respiratory droplets | Pneumonia, Meningitis | 24% |
Salmonella spp. | Enteric pathogen | Contaminated food/water | Salmonellosis | 12-15% |
Staphylococcus aureus | Opportunistic pathogen | Direct contact, contaminated surfaces | Skin infections, Food poisoning | 20% |
Clostridium difficile | Opportunistic pathogen | Antibiotic-associated disruption of gut flora | C. difficile infection (CDI) | 10-20% |
Bacterial Pathogens and Their Effects
A. What Makes a Bacterium Harmful?
In understanding what makes a bacterium harmful, it is crucial to examine the mechanisms by which these pathogens invade host organisms and evade the immune system. Pathogenic bacteria possess various virulence factors, including adhesins, toxins, and specialized secretion systems that play a vital role in their ability to adhere to host cells, ultimately leading to infection and disease. For instance, the Shigella species utilizes a sophisticated type III secretion system to inject effector proteins directly into host cells. This manipulation alters key host cell functions, enabling the bacteria to enter the interior of the cells and contributing to cell death, which can severely affect tissue integrity . These adaptive strategies not only facilitate the establishment of infections but also allow for substantial damage to the host organism, as they trigger inflammatory responses that may exacerbate the overall harm experienced by the host. Additionally, other complicating factors such as antibiotic resistance make treatment difficult and further increase the severity of bacterial infections. This phenomenon is particularly evident in certain bacteria known to exchange antibiotic-resistant genes through horizontal gene transfer, thereby spreading their resistance capabilities to other strains and species . Thus, the combination of effective invasion tactics and immune evasion mechanisms fundamentally characterizes harmful bacteria. This highlights the intricate and multifaceted nature of pathogenicity, opening up avenues for research and potential therapeutic interventions in microbiological studies, as understanding these processes is essential for developing effective strategies to combat bacterial infections.
Bacterium | Transmission | Disease Caused | Virulence Factor | Incidence Rate (per 100,000) |
Escherichia coli (E. coli) | Contaminated food or water | Gastroenteritis | Shiga toxin | 2.2 |
Staphylococcus aureus | Skin-to-skin contact, contaminated surfaces | Skin infections, pneumonia | Protein A, polysaccharide capsule | 14.6 |
Streptococcus pneumoniae | Respiratory droplets | Pneumonia, meningitis | Capsule, pneumolysin | 10.5 |
Clostridium botulinum | Improperly canned or preserved foods | Botulism | Botulinum toxin | 0.0003 |
Mycobacterium tuberculosis | Airborne particles | Tuberculosis | Mycolic acid in cell wall | 2.5 |
Characteristics of Pathogenic Bacteria
B. Routes of Bacterial Transmission
Bacterial transmission occurs through several established routes, each facilitating the spread of pathogenic bacteria and contributing to disease outbreaks. One primary route is direct contact, wherein bacteria are transmitted through physical touch or body fluids, such as in the case of skin infections or sexually transmitted infections. This type of transmission highlights the importance of personal hygiene and safe practices in both healthcare and daily life, as the presence of bacteria on hands or skin can lead to infections if proper precautions are not taken. Additionally, airborne transmission serves as a significant mechanism, allowing bacteria to spread through respiratory droplets generated when an infected individual coughs or sneezes. This method is particularly relevant for pathogens like Streptococcus pneumoniae, responsible for respiratory infections, and emphasizes the necessity of proper ventilation and the wearing of masks in crowded or enclosed spaces to help curtail the dissemination of harmful bacteria. Another notable route is via vectors, which are living organisms, often insects, that carry bacteria from one host to another, thus amplifying the potential for disease spread. Common examples include mosquitoes and ticks, which can transfer bacteria after feeding on an infected host. Lastly, indirect transmission can occur through contaminated objects or surfaces, including utensils, doorknobs, and medical equipment, emphasizing the critical role of hygiene practices in breaking transmission cycles. Regular cleaning and sanitizing of these surfaces are essential measures in preventing infection. Understanding these varied routes of bacterial transmission is vital for implementing effective public health strategies to mitigate bacterial infections and protect community health, ensuring a proactive approach in safeguarding populations from communicable diseases.
Routes of Bacterial Transmission
Route of Transmission | Description | Examples of Bacteria | Common Diseases |
---|---|---|---|
Direct Contact | Spread through physical contact, such as touching, kissing, or sexual contact | Staphylococcus aureus, Treponema pallidum | Skin infections, Syphilis |
Indirect Contact | Transmission via contaminated surfaces (fomites) such as doorknobs, medical instruments, or clothing | Clostridium difficile, Methicillin-resistant Staphylococcus aureus (MRSA) | Hospital-acquired infections, Gastrointestinal infections |
Droplet Transmission | Spread through respiratory droplets from coughing, sneezing, or talking (short-range) | Streptococcus pneumoniae, Bordetella pertussis | Pneumonia, Whooping cough |
Airborne Transmission | Transmission via tiny aerosolized particles that remain suspended in the air over long distances | Mycobacterium tuberculosis, Legionella pneumophila | Tuberculosis, Legionnaires’ disease |
Waterborne Transmission | Spread through contaminated water sources, often linked to poor sanitation | Vibrio cholerae, Salmonella typhi | Cholera, Typhoid fever |
Foodborne Transmission | Occurs through ingestion of contaminated food, often due to improper handling or storage | Escherichia coli, Listeria monocytogenes | Food poisoning, Listeriosis |
Vector-Borne Transmission | Spread through insects or arthropods such as mosquitoes, fleas, or ticks | Yersinia pestis (fleas), Borrelia burgdorferi (ticks) | Plague, Lyme disease |
Vertical Transmission | Transmission from mother to child during pregnancy, childbirth, or breastfeeding | Treponema pallidum, Listeria monocytogenes | Congenital syphilis, Neonatal listeriosis |
Zoonotic Transmission | Spread from animals to humans through direct contact, bites, or handling animal products | Brucella spp., Bacillus anthracis | Brucellosis, Anthrax |
Iatrogenic Transmission | Transmission due to medical procedures, including contaminated instruments or transfusions | Pseudomonas aeruginosa, Hepatitis B virus (though viral, can co-infect with bacteria) | Surgical infections, Post-surgical wound infections |
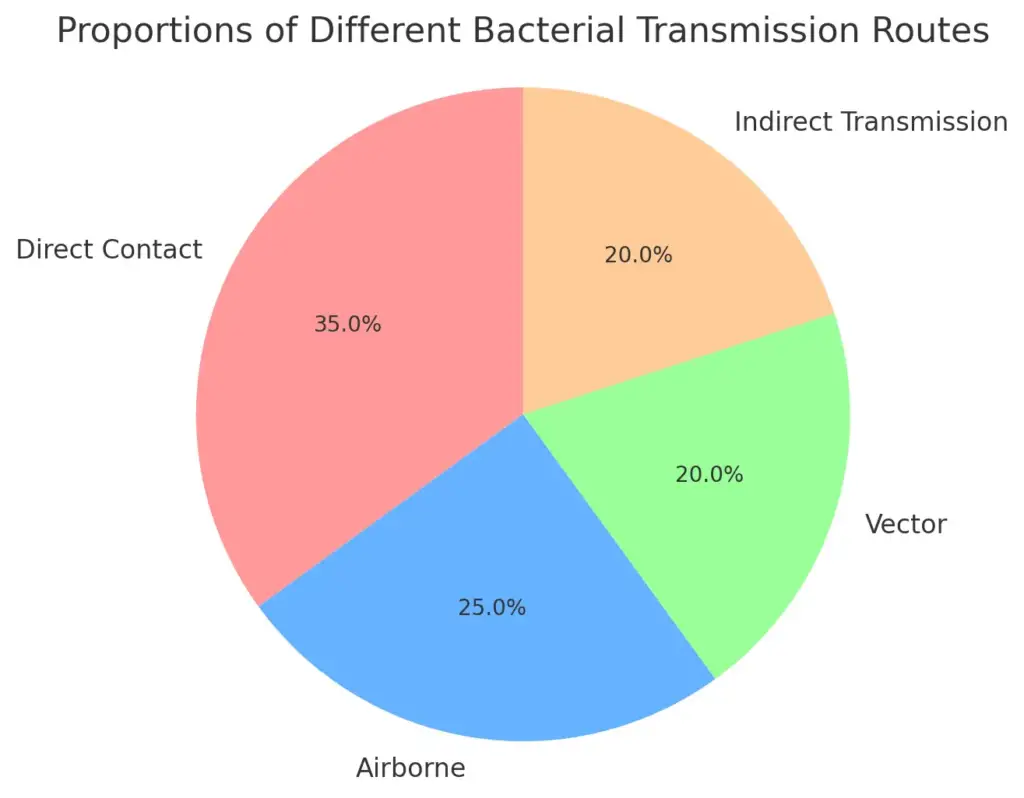
This pie chart illustrates the proportions of different bacterial transmission routes. Direct contact represents the primary method of transmission, accounting for 35% of cases, followed by airborne transmission at 25%. Both vector and indirect transmission equally contribute to 20%, highlighting the need for targeted public health interventions.
II. Common Types of Pathogenic Bacteria
Pathogenic bacteria are responsible for a myriad of infectious diseases that can significantly impact human health, leading to both acute and chronic conditions. Among the most notorious types are Salmonella, which is commonly associated with foodborne illnesses characterized by diarrhea, fever, and abdominal cramps, and Shigella, known for causing severe dysentery with symptoms that may include intense diarrhea, fever, and abdominal pain that can lead to dehydration in vulnerable populations. Both bacteria engage in complex transmission methods; for instance, Salmonella is often transmitted through contaminated food or water, particularly undercooked poultry, eggs, or produce, while Shigella spreads primarily via fecal-oral routes, often as a result of poor sanitation and hygiene practices, underscoring the critical importance of hygiene and food safety practices in disease prevention. Other notable pathogens include Streptococcus pneumoniae, responsible for a range of respiratory infections, including pneumonia, meningitis, and otitis media (ear infections), and Escherichia coli, particularly certain strains like E. coli O157:H7, some of which can lead to severe gastrointestinal disease, including bloody diarrhea and hemolytic uremic syndrome. Understanding these common pathogenic bacteria, their transmission pathways, and their mechanisms of causing disease is crucial for developing effective public health strategies to mitigate their impact. The intricate process of Shigella invasion in host cells, underscoring the complex mechanisms that facilitate the pathogenesis of this harmful bacterium, while also emphasizing the need for continued research and public education to combat these prevalent infectious threats to health.
Bacteria | Transmission | Diseases | Key Statistics |
Escherichia coli | Fecal-oral route | Gastroenteritis, urinary tract infections | Infection rates can exceed 265,000 cases annually in the U.S. |
Staphylococcus aureus | Direct contact, contaminated surfaces | Skin infections, pneumonia, bloodstream infections | Over 80,000 invasive procedures occur each year, leading to more than 11,000 deaths. |
Streptococcus pneumoniae | Respiratory droplets | Pneumonia, meningitis, middle ear infections | Causes more than 150,000 hospitalizations annually in the U.S. |
Salmonella | Contaminated food and water | Salmonellosis, typhoid fever | Estimated 1.35 million infections occur annually in the U.S. |
Mycobacterium tuberculosis | Airborne droplets | Tuberculosis | Approximately 9,000 new cases in the U.S. each year. |
Common Types of Pathogenic Bacteria
A. Respiratory Pathogens (Tuberculosis, Pneumonia)
Respiratory pathogens, particularly those causing tuberculosis (TB) and pneumonia, represent significant public health challenges worldwide due to their complex modes of transmission and the severity of the diseases they cause. Tuberculosis, which is primarily caused by the bacterium Mycobacterium tuberculosis, is transmitted through aerosolized droplets produced when an infected individual coughs or sneezes, allowing the bacterium to infiltrate the respiratory systems of susceptible individuals in close proximity. This mode of transmission is particularly concerning in densely populated areas, where the risk of spread can dramatically increase. Pneumonia, on the other hand, can stem from a variety of bacterial pathogens, including but not limited to Streptococcus pneumoniae and Klebsiella pneumoniae. These infections often develop following a viral illness that compromises the first-line immune defenses of the body, making individuals more vulnerable to secondary infections. The rapid transmission of these pathogens through respiratory droplets, combined with contributing factors such as crowded living conditions, poor ventilation, and the presence of weakened immune systems, facilitates outbreaks of pneumonia and tuberculosis. Understanding the complex dynamics of these pathogens, including their epidemiology and antimicrobial resistance patterns, is crucial for developing effective prevention and control strategies. The diagram depicting the transmission pathways of respiratory pathogens highlights the routes of transmission and underscores the importance of addressing both environmental and social determinants in curbing the spread of these infections. By recognizing and mitigating risk factors, healthcare systems can enhance their response and improve health outcomes for affected populations.
Detailed Table on Respiratory Pathogens (Tuberculosis & Pneumonia)
Feature | Tuberculosis (TB) | Pneumonia |
---|---|---|
Causative Agent | Mycobacterium tuberculosis (M. tuberculosis) | Multiple bacterial causes, including Streptococcus pneumoniae, Haemophilus influenzae, Klebsiella pneumoniae, Mycoplasma pneumoniae, Legionella pneumophila |
Mode of Transmission | Airborne transmission via inhalation of droplet nuclei from coughing, sneezing, or talking | Droplet transmission (coughing, sneezing), direct contact, aspiration of bacteria, or ventilator-associated infections |
Primary Site of Infection | Lungs (especially alveoli), but can spread to other organs (extrapulmonary TB) | Lungs, affecting alveoli and bronchioles |
Incubation Period | 2–12 weeks (can remain latent for years) | 1–10 days, depending on the pathogen |
Symptoms | Persistent cough (often with blood-tinged sputum), weight loss, night sweats, fever, fatigue, chest pain | Fever, chills, cough (with mucus or pus), difficulty breathing, chest pain, fatigue |
Types | Latent TB (asymptomatic, non-contagious), Active TB (symptomatic, contagious), Extrapulmonary TB (affects other organs) | Bacterial pneumonia (caused by bacteria), Viral pneumonia, Fungal pneumonia, Aspiration pneumonia, Hospital-acquired pneumonia |
Risk Factors | Weak immune system (HIV, malnutrition, diabetes), crowded living conditions, exposure to active TB cases | Elderly, infants, chronic illness, weakened immune system, smoking, hospitalization, exposure to contaminated respiratory secretions |
Diagnosis | Tuberculin skin test (TST), Interferon-gamma release assays (IGRAs), Chest X-ray, Sputum smear microscopy, Culture, PCR-based tests (GeneXpert) | Chest X-ray, Sputum culture, Blood tests, PCR, Urine antigen tests, Bronchoscopy (in severe cases) |
Treatment | First-line anti-TB drugs: Isoniazid, Rifampicin, Ethambutol, Pyrazinamide (usually for 6 months) | Antibiotics: Streptococcus pneumoniae – Penicillin, macrolides, fluoroquinolones; Mycoplasma pneumoniae – Macrolides, tetracyclines; Klebsiella pneumoniae – Carbapenems, cephalosporins |
Drug Resistance | Multidrug-resistant TB (MDR-TB) and Extensively Drug-resistant TB (XDR-TB) are major concerns | Antibiotic resistance increasing in some strains (MRSA pneumonia, multidrug-resistant Klebsiella pneumoniae) |
Prevention | BCG vaccine, early detection and treatment, proper ventilation, use of masks in high-risk areas | Vaccination (Pneumococcal vaccine, Hib vaccine, Influenza vaccine), good hygiene, smoking cessation |
Complications | Pulmonary fibrosis, lung cavities, extrapulmonary TB (meningitis, bone TB), respiratory failure, death | Septicemia, respiratory failure, lung abscess, pleural effusion, multi-organ failure |
Mortality Rate | ~10% for active TB cases if untreated; MDR-TB and XDR-TB have higher fatality rates | Varies: Community-acquired pneumonia (CAP): 5–15%; Hospital-acquired pneumonia (HAP): Up to 50% in severe cases |
Global Burden | One of the top 10 causes of death worldwide (~10 million cases annually, ~1.3 million deaths in 2022) | Leading infectious cause of death, particularly in children and elderly (millions of cases annually) |
B. Gastrointestinal Pathogens (Salmonella, E. coli)
Gastrointestinal pathogens such as Salmonella and E. coli are significant contributors to foodborne illnesses worldwide, posing substantial risks to public health that cannot be overstated. Transmission typically occurs through the consumption of contaminated food or water, highlighting the importance of proper food handling and sanitation practices in both home and commercial kitchens. Public awareness and education on these practices are vital to mitigate the risk factors associated with these pathogens. Once ingested, these bacteria have the capability to adhere to the intestinal lining effectively, employing a variety of sophisticated virulence factors to invade host cells and evade the immune response put up by the human body. For instance, Salmonella utilizes specialized secretion systems to inject proteins into host cells that manipulate crucial cellular processes, thus facilitating its survival and replication within the harboring organism. Similarly, certain strains of E. coli, particularly those classified as Enterohemorrhagic E. coli (EHEC), release potent toxins that can lead to severe gastrointestinal distress, including diarrhea and, in some cases, life-threatening complications such as hemolytic uremic syndrome. Understanding the intricate mechanisms of these pathogens is essential for developing effective prevention strategies and treatment protocols tailored to counteract their effects. Furthermore, the transmission pathways illustrated in authoritative studies provide critical insights into controlling the spread of these pathogens and safeguarding public health from gastrointestinal diseases, reinforcing the need for rigorous monitoring and rapid response systems when outbreaks occur.
Detailed Table on Gastrointestinal Pathogens (Salmonella & E. coli)
Feature | Salmonella | Escherichia coli (E. coli) |
---|---|---|
Causative Agent | Salmonella enterica (includes S. Typhi, S. Paratyphi, S. Typhimurium, S. Enteritidis) | Escherichia coli (includes multiple pathogenic strains such as EPEC, ETEC, EHEC, STEC, EAEC, DAEC) |
Mode of Transmission | Fecal-oral route, contaminated food (poultry, eggs, dairy, seafood), contaminated water, direct contact with infected animals | Fecal-oral route, contaminated food (undercooked meat, raw vegetables, unpasteurized dairy), contaminated water, person-to-person contact |
Primary Site of Infection | Small intestine (enteric fever), Large intestine (gastroenteritis) | Small intestine (ETEC, EPEC), Large intestine (EHEC, STEC) |
Incubation Period | 6 hours – 7 days (varies by strain) | 1–10 days, depending on strain |
Symptoms | Fever, abdominal cramps, diarrhea (can be bloody in severe cases), nausea, vomiting, dehydration | Watery or bloody diarrhea, abdominal cramps, nausea, vomiting, fever (varies by strain) |
Types | Typhoidal Salmonella (S. Typhi, S. Paratyphi) – Causes typhoid fever, systemic infection | Enteropathogenic E. coli (EPEC) – Infant diarrhea; Enterotoxigenic E. coli (ETEC) – Traveler’s diarrhea; Enterohemorrhagic E. coli (EHEC/STEC) – Bloody diarrhea, hemolytic uremic syndrome (HUS); Enteroaggregative E. coli (EAEC) – Persistent diarrhea |
Risk Factors | Consuming contaminated food or water, poor sanitation, weakened immune system, travel to endemic areas | Contaminated food/water, poor hygiene, close contact with infected individuals, young children and elderly at higher risk |
Diagnosis | Stool culture, blood culture (for typhoid fever), PCR, Widal test (for typhoid) | Stool culture, PCR, serotyping (for EHEC/STEC), urine tests (for HUS cases) |
Treatment | Non-typhoidal Salmonella: Supportive care (hydration, electrolytes); Typhoidal Salmonella: Antibiotics (Ciprofloxacin, Azithromycin, Ceftriaxone) | Supportive care (fluid replacement); antibiotics for invasive infections (EPEC, ETEC: Fluoroquinolones, Macrolides); EHEC/STEC: No antibiotics (risk of HUS) |
Drug Resistance | Emerging resistance in typhoid strains (Multidrug-resistant S. Typhi and extensively drug-resistant S. Typhi) | Some E. coli strains resistant to fluoroquinolones, cephalosporins, and carbapenems |
Prevention | Hand hygiene, proper food handling, vaccination (Typhoid vaccine), safe drinking water | Proper food preparation, handwashing, avoiding raw or unpasteurized foods, safe water sources |
Complications | Dehydration, bacteremia, reactive arthritis, chronic carrier state (typhoid fever) | Hemolytic Uremic Syndrome (HUS) (EHEC), kidney failure, sepsis in immunocompromised patients |
Mortality Rate | Typhoid fever: ~10% if untreated, <1% with treatment; Non-typhoidal Salmonella: Low mortality but significant morbidity | Generally low but higher for STEC (EHEC) due to HUS-related complications |
Global Burden | ~11–20 million cases of typhoid fever annually, ~150,000 deaths; non-typhoidal Salmonella causes ~93 million cases of gastroenteritis per year | ETEC causes ~280 million cases of diarrhea annually; STEC (EHEC) causes ~2.8 million cases globally |
C. Skin and Wound Infections (MRSA, Streptococcus)
Skin and wound infections caused by pathogenic bacteria, particularly Methicillin-resistant Staphylococcus aureus (MRSA) and various species of Streptococcus, pose significant public health challenges that are increasingly alarming due to their rising prevalence and resistance to standard treatment modalities. MRSA, a particularly troublesome variant of Staphylococcus aureus, is notorious for its remarkable ability to evade the effects of standard antibiotics, which complicates treatment efforts and escalates the risk of severe health complications if these infections are left unmanaged or inadequately treated. In the case of Streptococcus, infections can lead to serious health conditions such as cellulitis and necrotizing fasciitis—conditions that, if not addressed promptly and effectively, may escalate into systemic infections that pose a risk of severe morbidity and mortality. The transmission of these formidable bacteria often occurs through direct skin-to-skin contact or by way of contaminated surfaces and objects, a situation that can be exacerbated by poor hygiene practices and close living quarters. A comprehensive understanding of the mechanisms underlying virulence and transmission of these pathogens, accompanied by the implementation of effective preventive strategies, is absolutely critical to managing these infections and improving patient outcomes. For a more thorough visual representation of the clinical manifestations associated with these infections, the image detailing the mechanisms of skin and wound infections would prove invaluable, as it encapsulates the intricate complexity of bacterial interactions within host tissues and underscores the urgent need for awareness and action in combating these public health threats.
Detailed Table on Skin and Wound Infections (MRSA & Streptococcus)
Feature | Methicillin-Resistant Staphylococcus aureus (MRSA) | Streptococcus (Group A & B Streptococci) |
---|---|---|
Causative Agent | Staphylococcus aureus (resistant to methicillin and other beta-lactam antibiotics) | Group A Streptococcus (GAS): Streptococcus pyogenes Group B Streptococcus (GBS): Streptococcus agalactiae |
Mode of Transmission | Direct contact with infected wounds, contaminated hands, surfaces, medical equipment | Direct contact with infected wounds, respiratory droplets (for GAS), maternal transmission (for GBS) |
Primary Site of Infection | Skin, wounds, surgical sites, bloodstream, lungs | Skin, throat, soft tissues, bloodstream, neonatal infections (GBS) |
Incubation Period | 1–10 days, varies by strain | GAS: 1–5 days GBS: Variable, can be early-onset (within 7 days) or late-onset (after a few weeks) in newborns |
Symptoms | Red, swollen, painful skin lesions, pus-filled abscesses, fever; in severe cases: necrotizing fasciitis, pneumonia, sepsis | GAS: Red, painful sores, impetigo, cellulitis, necrotizing fasciitis, toxic shock syndrome GBS: Skin infections, cellulitis, life-threatening sepsis in newborns |
Types | Healthcare-associated MRSA (HA-MRSA): Acquired in hospitals Community-associated MRSA (CA-MRSA): Acquired outside hospitals Livestock-associated MRSA (LA-MRSA): Found in animals and transmitted to humans | GAS: Causes strep throat, scarlet fever, impetigo, and severe skin infections GBS: Common in newborn infections, pregnant women, and elderly individuals |
Risk Factors | Hospitalization, invasive procedures (surgeries, catheters), weakened immune system, crowded settings (prisons, gyms) | Open wounds, poor hygiene, diabetes, weakened immune system, childbirth (for GBS) |
Diagnosis | Wound culture, blood culture, PCR, susceptibility testing | Throat swab (for GAS), blood culture, wound culture, rapid antigen detection test (for strep throat) |
Treatment | Vancomycin, Daptomycin, Linezolid, Clindamycin, TMP-SMX (depends on resistance profile) | GAS: Penicillin, Amoxicillin, Clindamycin (for severe infections) GBS: Penicillin, Ampicillin, Clindamycin (for penicillin allergy) |
Drug Resistance | High resistance to beta-lactam antibiotics, some strains resistant to Vancomycin (VRSA) | Generally sensitive to penicillin, but increasing macrolide resistance in some strains |
Prevention | Hand hygiene, proper wound care, decontaminating surfaces, screening high-risk hospital patients | Good hygiene, wound care, treating strep throat promptly, screening pregnant women for GBS |
Complications | Cellulitis, bloodstream infections, osteomyelitis, pneumonia, toxic shock syndrome, necrotizing fasciitis | GAS: Rheumatic fever, glomerulonephritis, toxic shock syndrome, flesh-eating disease GBS: Neonatal sepsis, meningitis, pneumonia |
Mortality Rate | Severe MRSA infections: ~10-30% | Severe GAS infections: ~25-35% (necrotizing fasciitis) Neonatal GBS infections: ~5% |
Global Burden | A major public health issue, causing >100,000 deaths per year globally | GAS: ~700 million infections per year, ~500,000 deaths GBS: A leading cause of neonatal sepsis and meningitis worldwide |
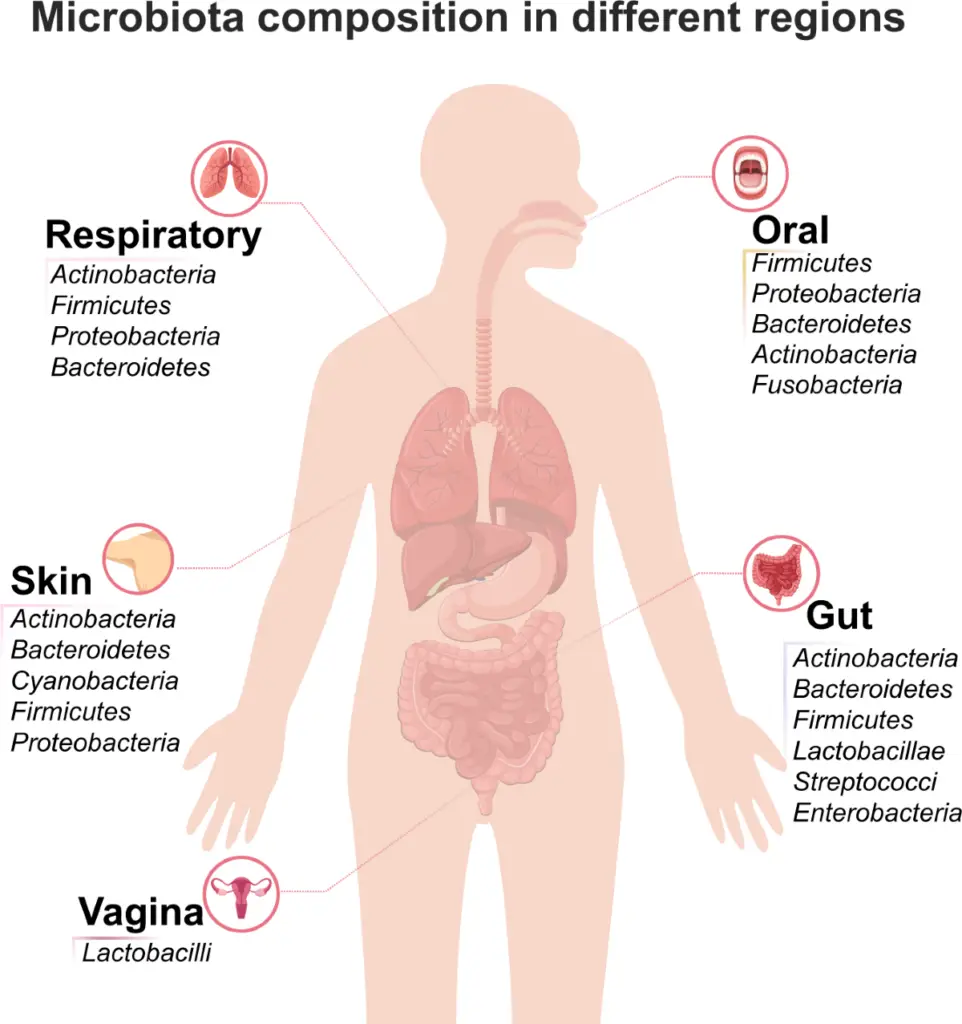
IMAGE – Microbiota Composition in Human Body Regions (The image presents a visual representation of the microbiota composition across various regions of the human body, including the respiratory system, oral cavity, skin, gut, and vagina. Each section is labeled with the dominant bacterial phyla or genera found in that particular area. For the respiratory system, Actinobacteria, Firmicutes, Proteobacteria, and Bacteroidetes are highlighted. In the oral cavity, Firmicutes, Proteobacteria, Bacteroidetes, Actinobacteria, and Fusobacteria are noted, while the skin showcases Actinobacteria, Bacteroidetes, Cyanobacteria, Firmicutes, and Proteobacteria. The gut is indicated to contain Actinobacteria, Bacteroidetes, Firmicutes, Lactobacillae, Streptococci, and Enterobacteria, and the vagina is comprised primarily of Lactobacilli. This diagram is relevant for studies in microbiology, human health, and the interactions between human microbiota and various bodily systems.)
III. How Bacteria Cause Disease
Bacterial pathogens employ a wide array of intricate mechanisms to invade host organisms and cause disease, reflecting their remarkable adaptability and cunning in overcoming host defenses. One critical strategy involves the secretion of virulence factors, which are proteins or toxins that not only facilitate colonization but also inflict damage to host tissues. For instance, the type III secretion system exemplifies how bacteria, such as Shigella, inject effector proteins directly into the cytoplasm of host cells, effectively disrupting normal cellular functions and thus promoting bacterial survival and replication within a hostile environment. These virulence factors can manipulate various signaling pathways within host cells, rendering them more susceptible to infection. Additionally, bacterial pathogens may exploit the intricacies of host immune responses, employing diverse strategies to evade detection or actively manipulate host defenses to their own advantage. These tactics often involve altering the host’s immune signaling processes or disguising themselves to appear innocuous. Such interactions underscore the complex and often antagonistic relationship between pathogenic bacteria and their hosts, illustrating how even brief encounters with organisms that may initially seem benign can lead to profound health consequences and significant disease outbreaks. Understanding these bacterial mechanisms not only sheds critical light on the underlying processes of infectious diseases but also informs public health strategies. This knowledge is essential to mitigate infections and formulate effective treatments for bacterial diseases, ultimately enhancing our ability to combat these formidable pathogens and protect public health on a broader scale.
How Bacteria Cause Disease: 10 Key Mechanisms
- Toxin Production (Exotoxins)
- Some bacteria release powerful protein toxins that damage tissues and disrupt body functions.
- Examples: Clostridium botulinum (Botulism), Corynebacterium diphtheriae (Diphtheria), Staphylococcus aureus (Toxic Shock Syndrome).
- Endotoxins (LPS in Gram-negative Bacteria)
- Lipopolysaccharides (LPS) in the outer membrane of Gram-negative bacteria trigger severe inflammation and septic shock.
- Examples: Escherichia coli (Septic shock), Salmonella typhi (Typhoid fever), Neisseria meningitidis (Meningitis).
- Direct Invasion of Host Cells
- Some bacteria enter host cells to multiply and spread, damaging tissues in the process.
- Examples: Listeria monocytogenes (Listeriosis), Mycobacterium tuberculosis (Tuberculosis), Shigella spp. (Dysentery).
- Immune System Evasion
- Bacteria develop ways to escape immune detection, such as producing protective capsules or changing surface proteins.
- Examples: Mycobacterium tuberculosis (Tuberculosis), Streptococcus pneumoniae (Pneumonia), Neisseria gonorrhoeae (Gonorrhea).
- Biofilm Formation
- Some bacteria form biofilms—sticky, protective layers that make them resistant to antibiotics and immune attacks.
- Examples: Pseudomonas aeruginosa (Chronic wounds), Staphylococcus epidermidis (Catheter infections), Helicobacter pylori (Gastric ulcers).
- Antigenic Variation
- Some bacteria frequently change their surface proteins to avoid being recognized by the immune system.
- Examples: Neisseria gonorrhoeae (Gonorrhea), Borrelia burgdorferi (Lyme Disease), Trypanosoma brucei (Sleeping Sickness).
- Interference with Host Signaling
- Certain bacteria inject toxins or effector proteins into host cells, disrupting normal cellular communication.
- Examples: Yersinia pestis (Plague), Escherichia coli (Traveler’s diarrhea), Salmonella spp. (Salmonellosis).
- Inducing Host Cell Death (Apoptosis or Necrosis)
- Some bacteria trigger programmed cell death, helping them spread while causing tissue damage.
- Examples: Bacillus anthracis (Anthrax), Legionella pneumophila (Legionnaires’ disease), Shigella spp. (Dysentery).
- Exploiting Host Nutrients
- Bacteria steal essential nutrients, such as iron, from the host to support their growth.
- Examples: Mycobacterium tuberculosis (Tuberculosis), Vibrio cholerae (Cholera), Neisseria meningitidis (Meningitis).
- Triggering Excessive Immune Response (Cytokine Storms)
- Some bacterial infections overstimulate the immune system, leading to tissue damage and organ failure.
- Examples: Streptococcus pyogenes (Sepsis, Toxic Shock Syndrome), Staphylococcus aureus (MRSA), Neisseria meningitidis (Meningococcal Sepsis).
These mechanisms explain how bacteria invade the body, evade the immune system, and cause disease.
A. The Role of Toxins (Endotoxins vs. Exotoxins)
Toxins play a crucial role in the pathogenicity of bacteria, classified primarily into two categories: endotoxins and exotoxins. Endotoxins, which are integral components of the Gram-negative bacterial cell wall, are released upon cell death and provoke systemic inflammatory responses, often leading to severe physiological effects such as fever and septic shock. When endotoxins enter the bloodstream, they can trigger an overactive immune response, which may result in widespread inflammation and can be life-threatening if not managed quickly. In contrast, exotoxins are actively secreted by both Gram-positive and Gram-negative bacteria and can exert potent effects on specific host cells or tissues. For instance, exotoxins like those produced by Clostridium botulinum can inhibit neurotransmitter release at neuromuscular junctions, resulting in paralysis and potentially fatal respiratory failure. The diversity of exotoxins, in terms of their biochemical mechanisms and the range of cells they affect, highlights the adaptability and cunning of bacterial pathogens. Understanding the mechanisms of these toxins is essential for developing targeted therapeutic strategies and vaccines, which can mitigate the effects of these harmful substances. The diagram referring to the mechanisms of bacterial proteins and their influence on immune response modulation effectively illustrates how these toxins interact within the host, exacerbating disease severity and complicating treatment efforts even further. Thus, distinguishing between endotoxins and exotoxins is vital for comprehending the complex dynamics of bacterial infections and their implications for public health, as this knowledge can inform effective clinical practices and potential interventions to protect populations from serious bacterial diseases.
Toxin Type | Source | Characteristics | Immune Response | Effects |
Endotoxins | Gram-negative bacteria (e.g., Escherichia coli) | Part of the bacterial cell wall (lipopolysaccharides) | Triggers systemic inflammatory response | Fever, septic shock, and potential organ failure |
Exotoxins | Gram-positive bacteria (e.g., Streptococcus, Staphylococcus) | Secreted by living bacteria, proteins | Can stimulate strong immune responses | Varied, including neurotoxic (e.g., tetanus) and enterotoxins (e.g., cholera) |
A-B Toxins | Various bacteria (e.g., Clostridium botulinum) | Consist of an active component (A) and a binding component (B) | Can be neutralized by specific antibodies | Inhibit protein synthesis or cellular processes |
Toxin Types and Their Effects
B. How Bacteria Evade the Immune System
Bacteria have developed sophisticated strategies to evade the host immune system, significantly enhancing their pathogenicity and survival in various environments. One common mechanism involves the secretion of specific proteins that effectively disrupt immune signaling pathways and modulate the host’s responses to the invaders. For instance, certain pathogens, such as Shigella, employ a complex type III secretion system that allows them to inject virulence factors directly into host cells, enabling them to hijack cellular processes and evade detection by the immune system. This intricate method of manipulation not only helps the bacteria to thrive within their host but also aids in their ability to escape the surveillance of immune cells. Additionally, many bacteria possess complex surface structures, including polysaccharide capsules, which hinder phagocytosis by immune cells. These capsules act as a protective cloak, effectively masking the pathogen from immune recognition and allowing for continued replication and survival in a hostile environment. These evasive tactics not only facilitate bacterial survival but also contribute to the persistent and chronic nature of infections that can ensue. Understanding the diverse mechanisms that bacteria utilize to evade the immune system is crucial for developing effective therapeutic strategies against bacterial diseases. For a relevant illustration, a comprehensive study is provided in [citation], which details Shigella’s invasion process and highlights the intricate interplay between various bacterial mechanisms and the immune response. This understanding is vital for enhancing our overall knowledge of pathogenicity in bacterial infections and can guide future research aimed at combating these resilient and adaptive microorganisms.
How Bacteria Evade the Immune System: A Detailed Table
Mechanism | Description | Examples of Bacteria | Diseases Caused |
---|---|---|---|
Capsule Formation | Some bacteria produce a thick polysaccharide capsule that prevents phagocytosis by immune cells. | Streptococcus pneumoniae, Neisseria meningitidis, Haemophilus influenzae | Pneumonia, Meningitis, Sepsis |
Antigenic Variation | Bacteria frequently change their surface proteins to evade immune recognition. | Neisseria gonorrhoeae, Borrelia burgdorferi, Trypanosoma brucei | Gonorrhea, Lyme Disease, Sleeping Sickness |
Biofilm Formation | Bacteria form protective communities (biofilms) that shield them from immune attacks and antibiotics. | Pseudomonas aeruginosa, Staphylococcus epidermidis, Helicobacter pylori | Chronic wounds, Catheter infections, Gastric ulcers |
Intracellular Survival | Some bacteria hide inside host cells to avoid detection and destruction by immune cells. | Mycobacterium tuberculosis, Listeria monocytogenes, Salmonella spp. | Tuberculosis, Listeriosis, Typhoid fever |
Inhibition of Phagosome-Lysosome Fusion | Certain bacteria prevent the fusion of phagosomes with lysosomes, avoiding destruction inside immune cells. | Mycobacterium tuberculosis, Legionella pneumophila, Chlamydia trachomatis | Tuberculosis, Legionnaires’ disease, Chlamydia |
Molecular Mimicry | Some bacteria mimic host molecules to evade immune detection. | Treponema pallidum, Streptococcus pyogenes, Helicobacter pylori | Syphilis, Rheumatic fever, Gastric ulcers |
Secretion of Immunosuppressive Proteins | Certain bacteria release proteins that interfere with immune signaling and cell function. | Yersinia pestis, Escherichia coli (EPEC), Salmonella spp. | Plague, Traveler’s diarrhea, Salmonellosis |
Destruction of Immune Cells | Some bacteria produce toxins that kill or disable immune cells like macrophages and neutrophils. | Staphylococcus aureus (PVL toxin), Streptococcus pyogenes (Streptolysin O), Bacillus anthracis | MRSA infections, Strep throat, Anthrax |
Iron Scavenging | Bacteria use siderophores to steal iron from the host, essential for their growth and survival. | Mycobacterium tuberculosis, Neisseria meningitidis, Vibrio cholerae | Tuberculosis, Meningitis, Cholera |
Superantigen Production | Some bacteria overstimulate the immune system, leading to immune exhaustion and tissue damage. | Staphylococcus aureus (Toxic Shock Syndrome Toxin), Streptococcus pyogenes | Toxic Shock Syndrome, Scarlet fever |
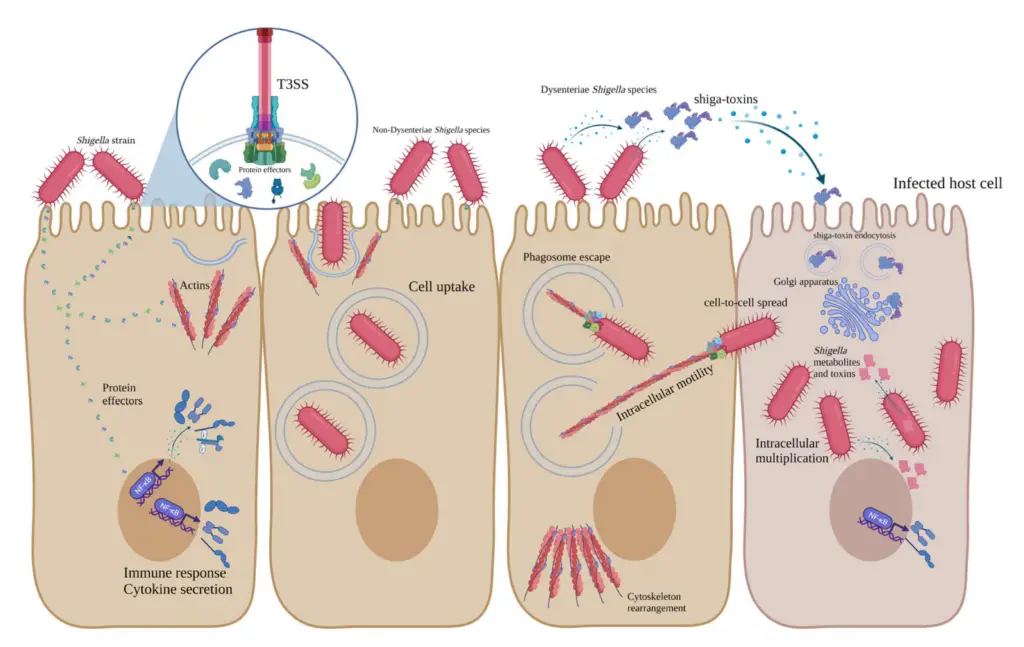
Image : Schematic representation of Shigella infection stages and mechanisms of host cell invasion. (The image depicts a detailed schematic of the infection process of Shigella bacteria within a host cell. It illustrates various stages of the Shigella invasion, starting with the injection of protein effectors through a type III secretion system (T3SS), followed by cell uptake, phagosome escape, and intracellular motility. Each stage is labeled with specific processes associated with Shigella, such as immune response activation, cytokine secretion, and intracellular multiplication. Additionally, the image highlights the differential effects of various Shigella strains, including dysentery-causing species and non-dysenteriae strains, demonstrating the complexity and pathogenicity of the bacteria within the host’s cellular environment. This illustration is relevant for studies in microbiology, immunology, and infectious diseases, providing a comprehensive overview of bacterial pathogenesis and host interactions.)
IV. How to Prevent and Treat Bacterial Infections
Preventing and treating bacterial infections involves a multifaceted approach that encompasses hygiene, vaccination, and antibiotic stewardship, all of which are critical for maintaining health in both individual and community contexts. Good hygiene practices, such as regular and thorough handwashing with soap and water, can significantly reduce the risk of bacterial transmission, especially in communal settings like schools, hospitals, and workplaces where germs can easily spread. Additionally, vaccines play a crucial role in preventing infections caused by specific bacteria, thereby reducing overall disease incidence and associated complications, such as severe illness and hospitalization. In clinical settings, the judicious use of antibiotics is imperative for effectively combatting infections while also preventing the emergence of antibiotic-resistant strains, which pose a significant challenge to modern medicine. Patients must also be educated on the importance of completing prescribed antibiotic courses to ensure that pathogenic bacteria are thoroughly eliminated and to prevent resistance from developing. Furthermore, adopting lifestyle factors such as maintaining adequate nutrition, engaging in regular physical activity, and practicing effective stress management can bolster the immune system’s ability to fend off infections more effectively. Collectively, these strategies not only empower individuals to safeguard their health but also contribute to a robust framework for the prevention and treatment of bacterial infections. This comprehensive approach reflects an integrated response that is essential for safeguarding public health, ultimately enhancing community well-being and resilience against the threat of bacterial diseases.
How to Prevent and Treat Bacterial Infections
Aspect | Prevention | Treatment |
---|---|---|
Hygiene Practices | Regular handwashing, personal cleanliness, and proper food handling reduce bacterial transmission. | Maintaining hygiene during treatment prevents further infections and complications. |
Vaccination | Immunization against bacterial diseases (e.g., TB, tetanus, diphtheria) prevents infections. | Some bacterial infections (e.g., tetanus, pertussis) require booster shots for continued immunity. |
Safe Food and Water | Proper cooking, refrigeration, and safe drinking water reduce foodborne infections. | Rehydration and supportive care are essential in treating foodborne bacterial infections. |
Antibiotics | Not used for prevention unless in high-risk cases (e.g., post-surgical prophylaxis). | Antibiotics like penicillin, cephalosporins, or macrolides treat bacterial infections, but must be used properly to prevent resistance. |
Avoiding Close Contact with Infected Individuals | Social distancing and protective measures help reduce the spread of contagious bacterial infections. | Isolation may be required for highly infectious diseases like tuberculosis. |
Wound Care | Proper cleaning and disinfection of cuts and wounds prevent bacterial infections. | Wound infections may require antibiotics, debridement, or drainage if severe. |
Probiotics and Healthy Diet | Strengthening gut microbiota can prevent infections like Clostridium difficile-associated diarrhea. | Probiotics may help restore healthy gut flora after antibiotic treatment. |
Hospital Infection Control | Sterilization, proper sanitation, and limited antibiotic use in hospitals prevent resistant bacterial infections. | Hospital-acquired infections like MRSA require specialized antibiotic therapy. |
Addressing Antibiotic Resistance | Responsible antibiotic use, avoiding self-medication, and completing prescribed courses prevent resistance. | Infections caused by antibiotic-resistant bacteria may require alternative or combination therapies. |
Public Health Measures | Mass vaccination, public awareness campaigns, and clean water initiatives reduce bacterial disease outbreaks. | Public health policies support surveillance, early diagnosis, and outbreak containment. |
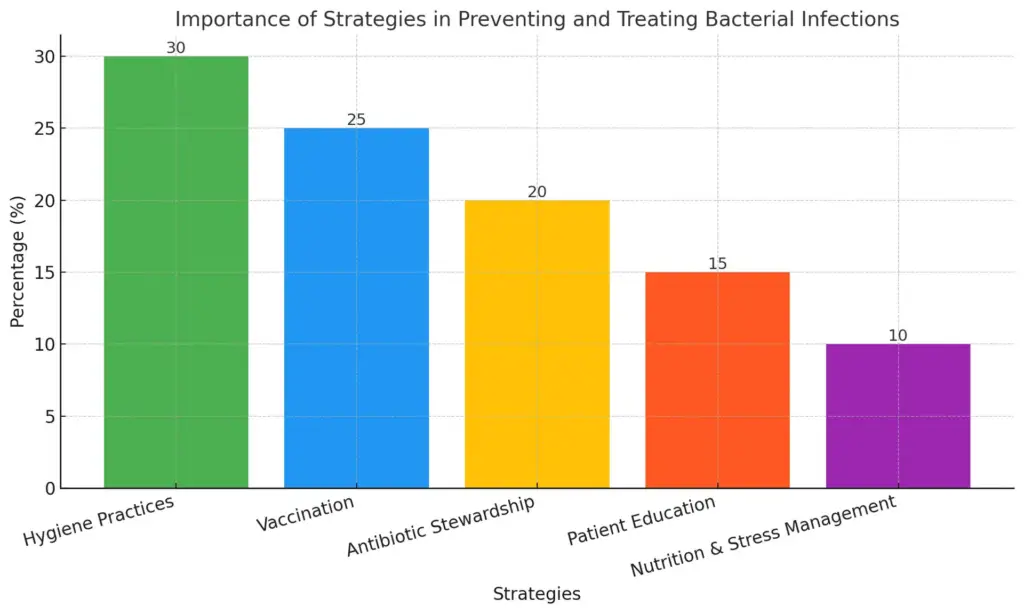
This bar chart represents the importance of various strategies in preventing and treating bacterial infections. Good hygiene practices are the most effective at 30%, followed by vaccination at 25%. Antibiotic stewardship and patient education play crucial roles at 20% and 15% respectively, while nutrition and stress management are essential but rank lower at 10%. This data emphasizes the multifaceted approach required for effective public health strategies.
A. The Role of Hygiene and Vaccination
Hygiene and vaccination are critical components in controlling the spread of pathogenic bacteria and mitigating the impact of infectious diseases that threaten public health on a global scale. Effective hygiene practices, such as regular handwashing with soap and water and the safe handling and preparation of food, significantly reduce transmission routes for harmful bacteria like Salmonella and Shigella, thereby preventing outbreaks and protecting both individual and community health in a comprehensive manner. Vaccination serves as a proactive defense mechanism, equipping the immune system to recognize and combat specific pathogens, which in turn diminishes susceptibility to diseases that can arise from various bacterial infections. For instance, vaccines can bolster public immunity against potentially life-threatening diseases like tetanus and diphtheria, both of which are caused by dangerous bacterial toxins. The interplay between stringent hygiene practices and effective vaccination programs emphasizes the importance of adopting a multi-faceted approach in public health strategies aimed at promoting wellness and preventing disease outbreaks. This dynamic synergy not only enhances individual immunity, allowing people to better fend off illness, but also plays a crucial role in contributing to herd immunity, which creates a safer and healthier environment for the population at large. The complexities of these interactions are well-visualized in the Stages of Emergence diagram, which underscores the transformational processes involved in pathogen transmission and the necessary community health measures required to address them. Ultimately, a holistic focus on both hygiene and vaccination is essential for fostering a resilient public health infrastructure and safeguarding communities against the ever-evolving threats posed by infectious diseases.
The Role of Hygiene and Vaccination in Disease Prevention
Aspect | Hygiene | Vaccination |
---|---|---|
Definition | Practices that reduce exposure to pathogens and limit their spread. | The administration of weakened or inactivated pathogens to stimulate immune response and build immunity. |
Primary Goal | Prevent direct and indirect transmission of infectious agents. | Train the immune system to recognize and combat specific pathogens. |
Methods | Handwashing, sanitization, proper food handling, clean water supply, personal hygiene, disinfection of surfaces. | Live-attenuated vaccines, inactivated vaccines, toxoid vaccines, subunit vaccines, mRNA vaccines. |
Effectiveness | Reduces pathogen load in the environment and limits outbreaks. | Provides long-term protection against specific diseases by creating immunological memory. |
Examples of Diseases Prevented | Diarrheal diseases, respiratory infections, skin infections, foodborne illnesses. | Measles, Polio, Influenza, Tetanus, Tuberculosis, COVID-19. |
Impact on Public Health | Decreases infection rates, reduces healthcare burden, improves quality of life. | Eradicates or significantly reduces infectious diseases, prevents epidemics and pandemics. |
Role in Herd Immunity | Indirectly protects others by limiting pathogen transmission. | Directly contributes to herd immunity by reducing the number of susceptible individuals. |
Challenges | Requires consistent personal and community adherence, accessibility to clean resources. | Vaccine hesitancy, logistical challenges in distribution, need for booster doses in some cases. |
Global Impact | Essential in developing and developed countries to control disease spread. | Has led to the eradication of smallpox and near-eradication of polio, and continues to save millions of lives annually |
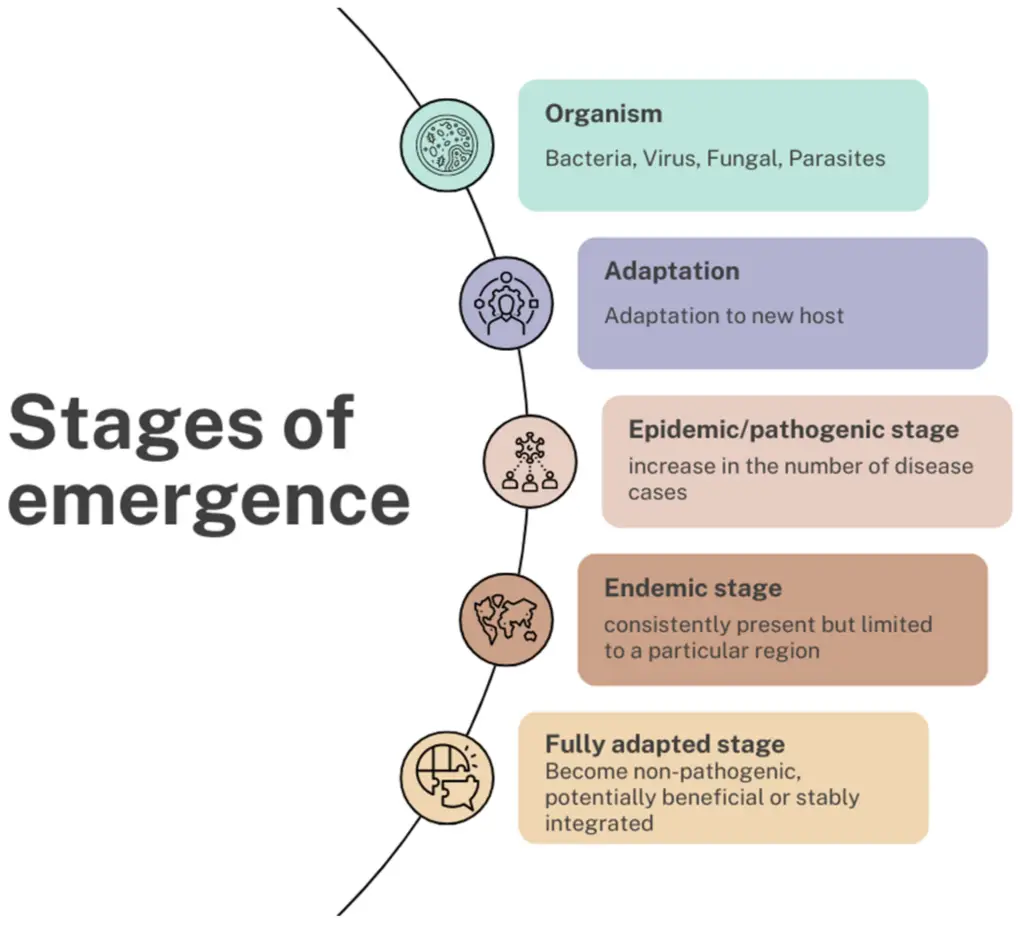
Image : Stages of Emergence of Infectious Diseases (The image titled ‘Stages of Emergence’ provides a visual representation of the various stages involved in the emergence of infectious diseases. It is sectioned into five distinct stages: ‘Organism,’ detailing the types of pathogens such as bacteria, viruses, fungi, and parasites; ‘Adaptation,’ which highlights the process of pathogens adjusting to new hosts; ‘Epidemic/pathogenic stage,’ describing the increase in disease cases; ‘Endemic stage,’ indicating a consistent but limited presence of a disease in a specific region; and ‘Fully adapted stage,’ illustrating how pathogens can become non-pathogenic or potentially beneficial. Each stage is represented using distinct colors and icons, facilitating understanding of the progression of infectious diseases.)
B. Antibiotics and Their Limitations
Antibiotics have undeniably revolutionized the treatment of bacterial infections, effectively reducing morbidity and mortality rates associated with various pathogens. However, their limitations pose significant challenges to public health that cannot be overlooked. One of the most pressing issues is the emergence of antibiotic resistance, which occurs when bacteria evolve mechanisms to withstand the effects of drugs designed to eliminate them. Mutation and horizontal gene transfer are due to how bacteria can adapt and survive in the presence of these treatments. Additionally, the misuse and overuse of antibiotics contribute to this growing problem, as unnecessary prescriptions and incomplete courses of treatment encourage resistant strains to flourish. This is compounded by the increasing prevalence of infections that do not respond to standard antibiotic therapy. Furthermore, antibiotics typically target specific characteristics of bacterial pathogens, rendering them less effective against virulent strains that employ evasion tactics, as seen in the resistance patterns of certain bacteria. As a result, even common infections have become harder to treat due to the emergence of superbugs. Therefore, a multifaceted approach, including responsible prescribing practices, public awareness campaigns, and ongoing research into alternative therapies, is essential to mitigate these limitations and sustain the efficacy of antibiotics in combating pathogenic bacteria. This includes promoting patient education on the importance of completing prescribed antibiotic courses and understanding when antibiotics are not an appropriate treatment option. Without such comprehensive strategies, the advancements made through antibiotics could be threatened, ultimately compromising the future of infectious disease treatment and public health.
Antibiotics and Their Limitations
Aspect | Description | Examples | Limitations |
---|---|---|---|
Definition | Antibiotics are drugs that kill or inhibit the growth of bacteria. | Penicillin, Tetracycline, Ciprofloxacin | Ineffective against viruses and fungi. |
Types | Broad-spectrum (affects many bacteria) and narrow-spectrum (targets specific bacteria). | Broad: Tetracycline, Narrow: Penicillin G | Broad-spectrum antibiotics can disrupt normal microbiota. |
Mechanism of Action | Targets bacterial cell wall, protein synthesis, DNA replication, or metabolism. | Beta-lactams (cell wall inhibitors), Macrolides (protein synthesis inhibitors) | Some bacteria develop resistance by modifying targets. |
Common Uses | Treat bacterial infections like pneumonia, UTIs, strep throat, and tuberculosis. | Amoxicillin for strep throat, Rifampin for TB | Misuse leads to resistance and reduced effectiveness. |
Resistance Development | Bacteria evolve mechanisms to survive antibiotic exposure. | MRSA (Methicillin-resistant Staphylococcus aureus), MDR-TB | Overuse and misuse accelerate resistance. |
Side Effects | Can cause allergic reactions, digestive issues, or secondary infections. | Rash from Penicillin, diarrhea from Clindamycin | Long-term use may damage gut microbiota. |
Superbugs | Resistant bacteria that no longer respond to multiple antibiotics. | Klebsiella pneumoniae (CRE), Pseudomonas aeruginosa | Infections become difficult or impossible to treat. |
Limitations in Viral Infections | Antibiotics do not work against viral diseases like flu, colds, or COVID-19. | Unnecessary use for viral infections leads to antibiotic resistance. | |
Need for Alternative Treatments | Phage therapy, probiotics, and new antibiotic discovery are being explored. | Bacteriophage therapy for drug-resistant infections | Research and development of new antibiotics are slow and expensive. |
Global Health Concern | WHO and CDC emphasize antibiotic stewardship to prevent resistance. | Awareness campaigns, prescription regulations | Poor regulation in some countries leads to antibiotic overuse. |
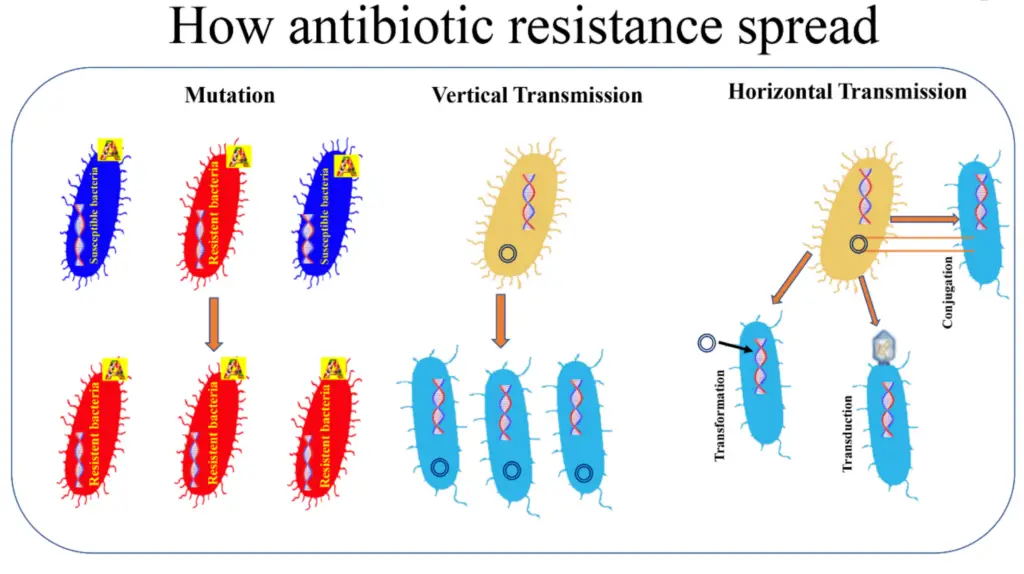
Image : Mechanisms of Antibiotic Resistance Spread (The image illustrates the mechanisms through which antibiotic resistance can spread among bacteria. It is divided into three sections: Mutation, Vertical Transmission, and Horizontal Transmission, each demonstrating different processes. Mutation shows susceptible bacteria evolving into resistant strains, while Vertical Transmission depicts the passing of resistant traits from one generation to the next. The Horizontal Transmission section highlights genetic exchange methods, such as conjugation, transformation, and transduction, which facilitate the sharing of resistance traits between different bacterial cells. This diagram serves as a valuable educational tool for understanding the complexities of antibiotic resistance in microbiology.)
V. The Need for Continuous Surveillance of Pathogenic Bacteria
The continuous surveillance of pathogenic bacteria is critically important in the ongoing fight against infectious diseases, as it facilitates early detection of emerging threats and allows for the systematic monitoring of antibiotic resistance patterns. In our current era, where bacterial pathogens are rapidly evolving and adapting to their environments and the introduction of new antibiotics and therapies, incorporating a strategic approach to surveillance can significantly mitigate potential outbreaks and health crises. For instance, understanding the transmission dynamics of various pathogens indicates how foodborne bacteria can infiltrate human populations, posing severe health risks and complicating existing health systems. Moreover, such an approach helps identify reservoirs and transmission vectors, which facilitates targeted interventions and the development of effective public health policies aimed at controlling spread. The necessity for ongoing monitoring is further underscored by the complexities of microbial interactions which demonstrate how pathogens can evade the immune response and potentially exacerbate infections, making treatment more challenging. By continuously gathering and analyzing data on pathogenic bacteria, health authorities can implement preventive measures that are timely and informed, which in turn helps protect public health and informs clinical practices. This proactive approach ultimately strives to reduce the burden of infectious diseases on society, ensuring healthier communities and minimizing healthcare costs. The quest to understand and track the evolution of these pathogens is not only a scientific endeavor but also a vital public health necessity that cannot be overlooked.
- Early Detection of Outbreaks
- Surveillance helps identify bacterial disease outbreaks before they become widespread.
- Example: Early monitoring of Salmonella outbreaks in food supplies prevents mass infections.
- Tracking Antibiotic Resistance
- Regular surveillance identifies emerging antibiotic-resistant bacterial strains.
- Example: Monitoring MRSA (Methicillin-resistant Staphylococcus aureus) in hospitals helps control its spread.
- Preventing Epidemics and Pandemics
- Identifying pathogenic bacteria early helps implement containment measures.
- Example: Tracking Vibrio cholerae in water sources prevents cholera outbreaks.
- Ensuring Food and Water Safety
- Monitoring bacteria in food processing and water supplies prevents foodborne illnesses.
- Example: Regular testing for E. coli and Listeria in food industries enhances public health safety.
- Improving Vaccine Development
- Continuous bacterial surveillance informs the need for new or updated vaccines.
- Example: Changes in Streptococcus pneumoniae serotypes guide pneumococcal vaccine updates.
- Understanding Bacterial Evolution
- Studying bacterial mutations and genetic changes helps predict future threats.
- Example: Evolution of Mycobacterium tuberculosis strains affects TB treatment strategies.
- Enhancing Hospital Infection Control
- Surveillance in healthcare settings reduces nosocomial (hospital-acquired) infections.
- Example: Monitoring Clostridioides difficile infections in hospitals improves sanitation protocols.
- Guiding Antibiotic Stewardship Programs
- Helps tailor antibiotic use policies to reduce resistance and improve treatment success.
- Example: Surveillance of Neisseria gonorrhoeae resistance patterns informs treatment guidelines.
- Assessing Environmental and Wildlife Reservoirs
- Surveillance of bacterial pathogens in animals and ecosystems prevents zoonotic disease transmission.
- Example: Monitoring Yersinia pestis in rodents helps prevent plague outbreaks.
- Strengthening Global Public Health Preparedness
- International bacterial surveillance networks improve global response to emerging threats.
- Example: WHO’s Global Antimicrobial Resistance Surveillance System (GLASS) tracks resistant bacteria worldwide.
REFERENCES
- Board on Health Promotion and Disease Prevention. ‘The Future of the Public’s Health in the 21st Century.’ Institute of Medicine, National Academies Press, 2/1/2003
- PenZen Summaries. ‘Summary of ALIEN Thinking – [Review Keypoints and Take-aways].’ by Mocktime Publication, 11/29/2022
- Pedro Escoll. ‘Bacterial Evasion of the Host Immune System.’ Caister Academic Press, 1/1/2017
- Committee on Damp Indoor Spaces and Health. ‘Damp Indoor Spaces and Health.’ National Academies Press, 8/31/2004
- Institute of Medicine. ‘U.S. Health in International Perspective.’ Shorter Lives, Poorer Health, National Research Council, National Academies Press, 4/12/2013
- Health and Medicine Division. ‘Global Health Impacts of Vector-Borne Diseases.’ Workshop Summary, National Academies of Sciences, Engineering, and Medicine, National Academies Press, 10/21/2016
- Mark Schneegurt. ‘Microbiology by OpenStax.’ Nina Parker, Open Stax Textbooks, 2/6/2023
- R. Hakenbeck. ‘Bacterial Cell Wall.’ J.-M. Ghuysen, Elsevier, 2/9/1994
- Devon Quick. ‘Anatomy & Physiology.’ Lindsay Biga, Oregon State Open Educational Resources, 9/26/2019
- Samuel Reid. ‘Academic Writing Skills 2 Student’s Book.’ Peter Chin, Cambridge University Press, 12/15/2011
- Alistair McCleery. ‘An Introduction to Book History.’ David Finkelstein, Routledge, 3/13/2006
Image References:
- Image: Microbiota Composition in Human Body Regions, Accessed: 2025.https://media.springernature.com/lw1200/springer-static/image/art%3A10.1038%2Fs41392-022-00974-4/MediaObjects/41392_2022_974_Fig1_HTML.png
- Image: Schematic representation of Shigella infection stages and mechanisms of host cell invasion., Accessed: 2025.https://pub.mdpi-res.com/ijms/ijms-24-02448/article_deploy/html/images/ijms-24-02448-g001.png?1674823316
- Image: Stages of Emergence of Infectious Diseases, Accessed: 2025.https://www.mdpi.com/genes/genes-15-00908/article_deploy/html/images/genes-15-00908-g001.png
- Image: Mechanisms of Antibiotic Resistance Spread, Accessed: 2025.https://www.mdpi.com/pathogens/pathogens-10-01310/article_deploy/html/images/pathogens-10-01310-g001.png
- Image: Mechanisms of bacterial invasion and immune response modulation, Accessed: 2025.https://media.springernature.com/lw685/springer-static/image/art%3A10.1038%2Fs41579-023-00865-7/MediaObjects/41579_2023_865_Fig1_HTML.png
- Image: Transmission pathways of Salmonella and its virulence factors, Accessed: 2025.https://www.mdpi.com/antibiotics/antibiotics-13-00076/article_deploy/html/images/antibiotics-13-00076-g001.png