Photosynthetic Bacteria: How Cyanobacteria Helped Shape Earth’s Atmosphere
Table of Contents
I. Introduction to Photosynthetic Bacteria
Photosynthetic bacteria, particularly cyanobacteria, play a pivotal role in understanding the early development of life on Earth and the evolution of its atmosphere. Emerging roughly 3.5 billion years ago, these remarkable microorganisms possess the unique ability to convert sunlight into chemical energy through photosynthesis, a complex biological process that not only sustains their growth but also significantly influences global atmospheric conditions. As primary producers, cyanobacteria contributed to the accumulation of oxygen in Earth’s atmosphere, initiating the Great Oxidation Event, a monumental shift that enabled the proliferation of aerobic organisms capable of utilizing oxygen for metabolic processes. This transformation marked a critical turning point in Earth’s biosphere, fundamentally altering the composition of the atmosphere and paving the way for the emergence and diversification of more complex life forms, such as multicellular plants and animals. The intricate relationship between photosynthetic bacteria and Earth’s environmental systems is further emphasized by geological timelines that meticulously chart significant milestones of such developments, such as the fossilization evidence of stromatolites formed by ancient cyanobacterial mats, which illustrate their influence on Earth’s environmental trajectory over millions of years. Understanding photosynthetic bacteria not only sheds light on the past but also provides insights into ecological processes and climate regulation in our current era. Their ability to thrive in diverse environments, from extreme heat and cold to highly saline conditions, highlights the adaptability of life on Earth, making photosynthetic bacteria a remarkable subject of study in evolutionary biology and ecological research.
Type | Photosynthesis Process | Approximate Age | Role in Atmosphere | Impact on Life |
Cyanobacteria | Oxygenic photosynthesis | 2.7 billion years | Oxygen production | Enabled aerobic organisms |
Green Sulfur Bacteria | Anoxygenic photosynthesis | 1 billion years | Contributed to sulfur cycle | Used by some anaerobic organisms |
Purple Bacteria | Anoxygenic photosynthesis | 1.5 billion years | Limited oxygen contribution | Ecological niches in anaerobic environments |
Key Facts About Photosynthetic Bacteria
A. How Photosynthesis Works in Bacteria
In understanding how photosynthesis operates in bacteria, particularly cyanobacteria, it is essential to explore the complex biochemical pathways that facilitate this remarkable process. Photosynthesis in cyanobacteria involves the intricate absorption of sunlight through specialized pigments, primarily chlorophyll a, which enables these microorganisms to convert solar energy into chemical energy with remarkable efficiency. Unlike plants, which rely on more varied mechanisms, cyanobacteria perform oxygenic photosynthesis, a process that uniquely releases oxygen as a byproduct while simultaneously fixing carbon dioxide into a range of organic compounds. This fundamental mechanism occurs within thylakoid membranes, which are crucial structures where both light-dependent and light-independent reactions take place. The engagement of these pathways not only fuels the metabolic processes within cyanobacteria but also contributes significantly to the atmospheric changes that shaped early Earth’s environment. The efficiency of this photosynthetic process not only sustained the growth and proliferation of diverse cyanobacterial communities but also played a pivotal role in the Great Oxidation Event, a monumental period that dramatically altered the composition of the planet’s atmosphere, leading to the accumulation of atmospheric oxygen. This transformative phase laid the groundwork for the evolution of aerobic organisms and the complex ecosystems we see today. By examining these interconnected processes, we can gain a deeper appreciation for the significant role that cyanobacteria continue to play in the biosphere.
Bacteria Type | Photosynthesis Type | Primary Pigments | Cellular Location of Photosynthesis | Global Contribution to Oxygen Production (%) |
Cyanobacteria | Oxygenic | Chlorophyll a and Carotenoids | Thylakoid membranes | 20-30 |
Green Sulfur Bacteria | Anoxygenic | Chlorosomes | Cytoplasmic membranes | 0 |
Purple Bacteria | Anoxygenic | Bacteriochlorophylls | Cytoplasmic membranes | 0 |
Photosynthesis in Bacteria
B. The Role of Bacteria in Producing Oxygen
The emergence of cyanobacteria marked a pivotal moment in Earth’s history, fundamentally altering the planet’s atmosphere through oxygen production. These remarkable photosynthetic bacteria, which thrive in a diverse array of environments, utilized sunlight to perform an essential biochemical process. By converting carbon dioxide and water into glucose while simultaneously releasing oxygen as a byproduct, they significantly enhanced the levels of oxygen in the atmosphere. This vital process, known as oxygenic photosynthesis, not only contributed to a gradual increase in atmospheric oxygen concentrations but also laid the crucial groundwork for more complex life forms to begin to evolve and thrive. The role of cyanobacteria can be further contextualized within the extensive geological timeline, particularly during the Precambrian era, which illustrates significant milestones in both the evolution of life and the transformation of the atmosphere. As these microorganisms proliferated and occupied various ecological niches, they formed a variety of extensive mats and stromatolite structures, which played a fundamental role in shaping marine ecosystems and influencing biogeochemical cycles essential for life. Ultimately, their oxygen-producing capabilities enabled what is now referred to as the Great Oxidation Event, a major transformation in Earth’s environment that had far-reaching consequences for the planet’s climatic conditions. This monumental shift not only paved the way for the emergence of aerobic life but also set the stage for the incredibly diverse biosphere we observe today, which is characterized by a complex interplay of living organisms and their environments.
The Role of Bacteria in Producing Oxygen
Bacterial Group | Example Species | Mechanism of Oxygen Production | Ecological Importance |
---|---|---|---|
Cyanobacteria | Prochlorococcus | Photosynthesis using water as an electron donor, releasing oxygen. | Major contributor to global oxygen production, especially in oceans. |
Cyanobacteria | Synechococcus | Performs oxygenic photosynthesis in marine environments. | Helps regulate atmospheric oxygen levels. |
Cyanobacteria | Anabaena | Forms symbiotic relationships with plants and fixes nitrogen while producing oxygen. | Supports aquatic ecosystems and enhances soil fertility. |
Cyanobacteria | Nostoc | Produces oxygen through photosynthesis in terrestrial and freshwater environments. | Provides oxygen to enclosed ecosystems like rice paddies. |
Cyanobacteria | Trichodesmium | Marine nitrogen-fixing cyanobacterium that also releases oxygen. | Supports marine food webs and contributes to global oxygen cycling. |
Cyanobacteria | Oscillatoria | Generates oxygen in freshwater and marine ecosystems. | Plays a role in primary production and carbon fixation. |
Cyanobacteria | Gloeocapsa | Produces oxygen through photosynthesis in extreme environments. | Survives in desert crusts and polar regions, influencing local oxygen levels. |
Endosymbiotic Cyanobacteria (Chloroplast Ancestors) | Ancient cyanobacteria that evolved into chloroplasts | Basis for oxygen production in plants and algae. | Led to the Great Oxygenation Event and sustains Earth’s biosphere. |
Photosynthetic Bacteria (Non-Cyanobacteria) | Chloroflexus | Performs anoxygenic photosynthesis but contributes to ecosystem oxygen cycling. | Found in microbial mats and supports diverse microbial communities. |
Stromatolite-Forming Bacteria | Microbial mat cyanobacteria | Layered biofilms that trap sediments and produce oxygen. | Created some of the earliest oxygenated environments on Earth. |
II. Cyanobacteria: The Original Oxygen Producers
Cyanobacteria, often referred to as the original oxygen producers, played a pivotal role in transforming Earth’s primordial atmosphere into one conducive to life as we know it today. Through the remarkable process of photosynthesis, these microorganisms not only harnessed sunlight to convert carbon dioxide and water into glucose and oxygen but also initiated a groundbreaking shift in the planet’s chemical composition, setting the stage for subsequent biological evolution. The oxygen they released into both the ocean and atmosphere during the Great Oxidation Event significantly altered the conditions for life on Earth, paving the way for aerobic organisms to not only survive but thrive in a previously inhospitable environment. As depicted in the geological timeline, the emergence of cyanobacteria marked a critical juncture in Earth’s history, coinciding with a variety of significant geological and biological changes. This milestone was instrumental in facilitating the development of more complex life forms, including multicellular organisms, and laid the foundation for the biosphere as we understand it today. Moreover, the extensive presence of cyanobacteria in ancient oceans contributed to the formation of vast stromatolite structures, which serve as some of the oldest records of life on our planet. Thus, the contributions of cyanobacteria highlight a transformative era and serve as a poignant reminder of the intricate interconnectedness of life and the environment throughout Earth’s evolutionary narrative. By shaping the atmosphere and influencing the course of biological evolution, cyanobacteria are rightly recognized as one of the most fundamental contributors to the planet’s long and complex history of life.
Cyanobacteria: The Original Oxygen Producers
- First Organisms to Produce Oxygen – Cyanobacteria were the first life forms to perform oxygenic photosynthesis.
- Pioneers of the Great Oxygenation Event – Around 2.4 billion years ago, they triggered a massive increase in atmospheric oxygen.
- Photosynthesis Process – They use sunlight, water, and carbon dioxide to produce oxygen and organic matter.
- Evolution of Complex Life – Their oxygen production made Earth habitable for aerobic organisms.
- Ancestors of Plant Chloroplasts – Cyanobacteria were engulfed by early eukaryotic cells, leading to the evolution of plants.
- Survive in Extreme Environments – Found in oceans, freshwater, deserts, hot springs, and even polar ice.
- Primary Producers in Aquatic Ecosystems – Form the base of many food chains, supporting diverse life forms.
- Influence on Climate and Atmosphere – Contribute to carbon sequestration and maintain oxygen balance.
- Nitrogen Fixation – Convert atmospheric nitrogen into forms usable by plants and other organisms.
- Formation of Stromatolites – Fossilized microbial mats created by ancient cyanobacteria provide evidence of early life.
- Regulators of Oceanic Oxygen Levels – Marine cyanobacteria like Prochlorococcus and Synechococcus contribute significantly to Earth’s oxygen supply.
- Play a Role in Harmful Algal Blooms – Some species can overgrow in nutrient-rich waters, producing toxins.
- Important for Biotechnology – Used in biofuel production, bioplastics, and as a protein source.
- Potential for Space Exploration – Could be used in life-support systems to generate oxygen on Mars.
- Key to Understanding Early Earth – Studying them helps scientists learn how life evolved under primitive conditions.
- Help Maintain Ecosystem Balance – Regulate carbon and nitrogen cycles in natural environments.
- Survival Through Evolution – Have adapted to diverse habitats for over 3 billion years.
- Source of Bioactive Compounds – Produce compounds with antimicrobial and pharmaceutical applications.
- Influence on Earth’s Geological History – Helped shape minerals and rock formations through biogeochemical processes.
- Essential for Sustaining Life Today – Continue to be crucial oxygen producers, supporting modern ecosystems.
A. How Cyanobacteria Created Earth’s Oxygen Atmosphere
Cyanobacteria, often referred to as blue-green algae due to their distinctive pigment, played a pivotal role in transforming Earth’s atmosphere through their remarkable photosynthetic activities, which began approximately 2.7 billion years ago. These microorganisms harnessed sunlight to initiate a chemical process that converted carbon dioxide and water into glucose, a vital source of energy, and, critically, oxygen as a byproduct of this conversion. This pioneering process not only significantly elevated the levels of atmospheric oxygen but also culminated in the Great Oxidation Event, marking a major turning point in Earth’s evolutionary history. Prior to this pivotal event, the atmosphere was largely anoxic, meaning it lacked significant levels of oxygen, which in turn limited the complexity and diversity of life forms that could thrive. The oxygen produced by cyanobacteria didn’t just support the emergence and evolution of aerobic organisms, which require oxygen to survive; it also facilitated the development of the ozone layer. This protective layer serves as a shield, blocking harmful ultraviolet radiation from the sun, thus creating a more stable environment for life to flourish. The intricate relationship between cyanobacteria and oxygen production is vividly captured in various scientific studies and visual representations, which illustrate the timeline of crucial events that include the emergence of these microorganisms and their far-reaching impact on Earth’s complex atmosphere. Such illustrations and research shed light on the essential role cyanobacteria played, not just in oxygenating the atmosphere, but also in paving the way for the biodiversity we witness today.
B. The Great Oxygenation Event and Its Impact on Life
The Great Oxygenation Event (GOE), occurring approximately 2.4 billion years ago, marks a pivotal turning point in Earth’s evolutionary history, primarily driven by the metabolic activities of cyanobacteria. These photosynthetic microorganisms played an essential and transformative role in altering the planet’s atmosphere by producing oxygen as a byproduct of their photosynthetic processes. This release of oxygen into the atmosphere was not merely a slight increase but rather a dramatic and unprecedented escalation that fundamentally shifted the chemical composition of the air. Consequently, this substantial increase in atmospheric oxygen facilitated significant biological and ecological shifts, leading to the emergence of aerobic respiration, which proved to be far more efficient than the previously dominant anaerobic processes. As a result, this catalytic event enabled a wider variety of more complex and diverse life forms to begin to emerge in various ecological niches, setting the stage for the numerous evolutionary advancements that would follow. Moreover, the GOE induced dramatic environmental changes, causing ripple effects that influenced biogeochemical cycles and ultimately paved the way for the formation of the ozone layer. This newly formed ozone layer created a protective shield against harmful ultraviolet radiation, allowing life to flourish and evolve further in previously inhospitable environments. Such transformative impacts on life and the environment are well documented in the geological timelines presented in below image, which encapsulates key developments during this era and illustrates the intricate interplay between oxygen production and life diversification across eons, emphasizing the far-reaching consequences of this monumental event in Earth’s history.
How Cyanobacteria Created Earth’s Oxygen Atmosphere
Stage | Time Period | Key Process | Impact on Earth’s Atmosphere | Effects on Life & Environment |
---|---|---|---|---|
Emergence of Cyanobacteria | ~3.5 billion years ago | Cyanobacteria evolve and begin photosynthesis. | No significant oxygen accumulation yet. | Oxygen is rapidly consumed by reacting with iron and other elements. |
Oxygen Production Begins | ~3.0 billion years ago | Cyanobacteria produce oxygen via photosynthesis (splitting water molecules). | Oxygen reacts with dissolved iron in oceans, forming iron oxide deposits. | Formation of banded iron formations (BIFs), a major geological record. |
The Great Oxygenation Event (GOE) | ~2.4–2.3 billion years ago | Oxygen levels increase as iron sinks become saturated. | Free oxygen begins accumulating in the atmosphere. | Mass extinction of many anaerobic microbes (Oxygen Catastrophe). |
Formation of the Ozone Layer | ~2.3–2.0 billion years ago | Increased oxygen leads to ozone (O₃) formation in the upper atmosphere. | Protects Earth from harmful UV radiation. | Allows life to colonize shallow waters and land surfaces. |
Transition to an Oxygen-Rich Atmosphere | ~1.8 billion years ago | Oxygen levels continue to rise, altering atmospheric chemistry. | Oxygen becomes a permanent component of Earth’s air. | Supports the evolution of aerobic (oxygen-using) life forms. |
First Eukaryotic Cells Emerge | ~1.5–1.2 billion years ago | Some bacteria engulf cyanobacteria, forming chloroplasts in early algae. | Oxygen levels stabilize, supporting complex cell structures. | Leads to the evolution of plants and more complex ecosystems. |
Oxygen Levels Continue to Increase | ~800 million years ago | Oxygen reaches near-modern levels due to ongoing photosynthesis. | Supports large, multicellular life forms. | Enables the development of diverse animal and plant species. |
Cambrian Explosion | ~541 million years ago | High oxygen levels enable rapid diversification of animal life. | Oxygen concentrations support larger, more complex organisms. | Marks the rise of major animal phyla and complex ecosystems. |
Modern Oxygen Balance | Present Day | Cyanobacteria, algae, and plants maintain atmospheric oxygen. | Oxygen levels stabilize at ~21% of the atmosphere. | Supports all aerobic life and ecosystems on Earth. |
Future Implications | Billions of years ahead | Changes in oxygen production may occur due to environmental shifts. | Possible decrease if photosynthetic life declines. | Could impact climate, biodiversity, and planetary habitability. |
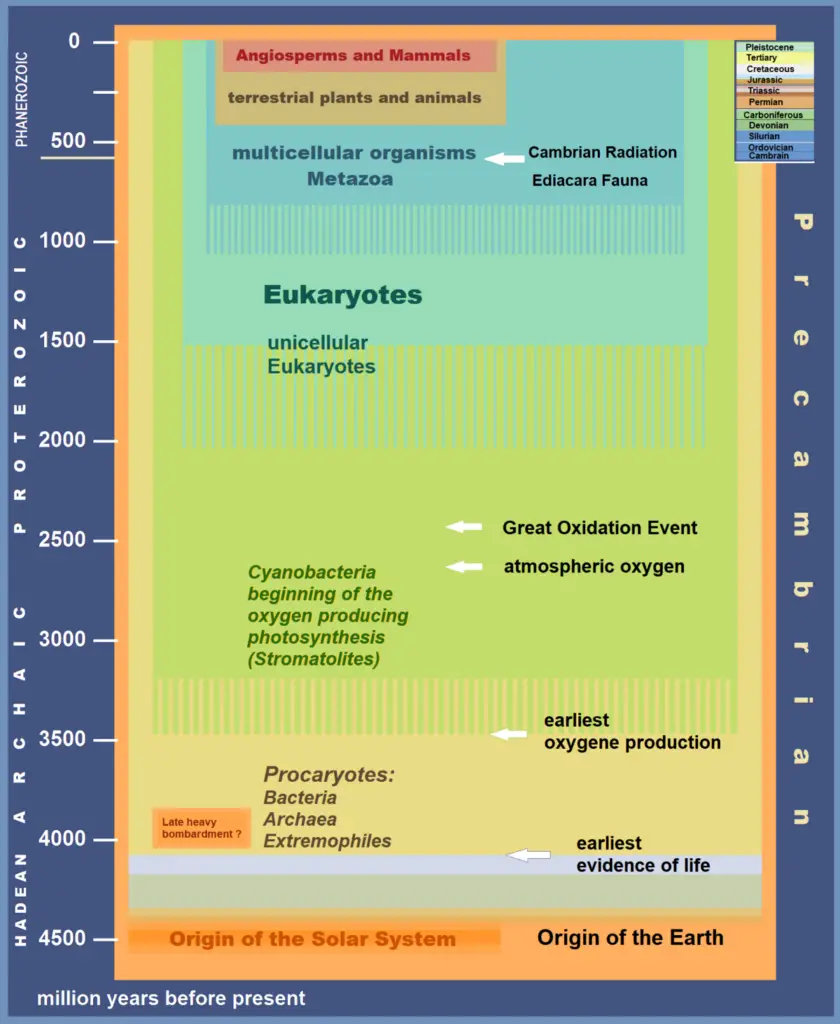
IMAGE – Geological time scale: Precambrian era and major biological milestones. (The image presents a geological time scale focused on the Precambrian era, illustrating major developmental milestones in Earth’s history from approximately 4.5 billion years ago to around 541 million years ago. Key events include the origin of the solar system, the emergence of Prokaryotes (such as bacteria and archaea), the beginning of oxygen production through cyanobacteria, and significant atmospheric changes, notably the Great Oxidation Event. The timeline highlights the transition from unicellular to multicellular life forms, culminating in the Cambrian Radiation and the appearance of Ediacara Fauna. Various eras and epochs are denoted along the vertical axis, with notable geological and biological advancements depicted in relation to time.)
III. Other Types of Photosynthetic Bacteria
While cyanobacteria are often credited with dramatically transforming Earth’s atmosphere through their oxygenic photosynthesis, other types of photosynthetic bacteria also play essential roles in various ecosystems, contributing to both ecological balance and nutrient availability. For instance, green sulfur bacteria and purple non-sulfur bacteria exhibit unique and specialized photosynthetic processes that are vital for organic matter production and nutrient cycling within their respective environments. Green sulfur bacteria thrive in anaerobic conditions, utilizing sulfide instead of water as an electron donor during their photosynthesis, allowing them to flourish in environments where light penetrates but oxygen is scarce. In contrast, purple non-sulfur bacteria demonstrate remarkable versatility by being able to switch between anaerobic photosynthesis and aerobic respiration. This adaptability enables them to thrive in a broader range of environments, capable of adjusting to fluctuations in light and oxygen availability that might arise due to changes in habitat conditions. Additionally, these bacteria not only contribute to energy capture through photosynthesis but also play critical roles in the cycling of essential elements like sulfur and carbon compounds. By capturing light energy and facilitating the cycling of both sulfur and organic compounds, they highlight the significant diversity of photosynthetic methods that exist beyond the realm of cyanobacteria. Their ecological roles underscore the intricate interdependencies within microbial communities, illustrating how these organisms impact both local and global ecosystems. Moreover, as evidenced by the geological time scales depicting their evolution and influence on Earth’s biochemical cycles, this broader perspective enriches our understanding of how various photosynthetic bacteria collectively shape ecological and atmospheric dynamics, revealing the complex interplay of life forms in the biosphere.
Type | Characteristics | Habitat | Example Species |
Cyanobacteria | Oxygenic photosynthesis, produce oxygen, and are essential for nitrogen fixation. | Aquatic environments, soil, and symbiotic relationships. | Synechocystis, Anabaena, Nostoc |
Green Sulfur Bacteria | Anoxygenic photosynthesis, use sulfur compounds as electron donors. | Deep anaerobic environments such as marine sediments. | Chlorobium, Pelodictyon |
Purple Bacteria | Anoxygenic photosynthesis, utilize organic compounds or hydrogen sulfide. | Aquatic environments, soil, and as symbionts. | Rhodospirillum, Rhodobacter |
Heliobacteria | Anoxygenic photosynthesis, capable of fermentative metabolism. | Soil and anaerobic environments. | Heliobacterium chlorum |
Types of Photosynthetic Bacteria
A. Purple and Green Sulfur Bacteria
Purple and green sulfur bacteria play a crucial role in the intricate tapestry of photosynthetic processes that have shaped Earth’s atmosphere, particularly during anoxic conditions prevalent in ancient aquatic environments. These remarkable bacteria utilize light energy to drive the conversion of carbon compounds while employing sulfide as an electron donor instead of water, which distinctly sets them apart from other photosynthetic organisms like cyanobacteria. The unique type of photosynthesis performed by these bacteria results in the production of both elemental sulfur and organic matter. This process is exceptionally important as it contributes significantly to the ecological dynamics of their habitats, fostering diverse biological communities. Their metabolic versatility equipped purple and green sulfur bacteria to thrive in stratified aquatic environments where light could penetrate but where oxygen levels were notably low, especially in the absence of competing organisms like cyanobacteria. This specialized adaptation allowed them to occupy a critical niche within these ecosystems. As a result, they offered valuable insights into early biogeochemical cycles and the evolution of photosynthetic pathways, shedding light on the complex interactions that have occurred over geological time. Understanding how these bacteria fit into the broader picture of Earth’s biological and chemical history is essential for appreciating their long-term impact. Including an image of the geological timeline showcasing significant events in this context, such as the emergence of these bacteria during the Precambrian, can greatly enhance our understanding of their role. This deeper exploration of their evolutionary significance further illustrates how purple and green sulfur bacteria have shaped the planet’s biogeochemistry.
Type | Photosynthetic Pigments | Habitat | Ecosystem Role | Examples |
Purple Sulfur Bacteria | Bacteriochlorophyll a, Carotenoids | Anaerobic environments (e.g., lakes, sediment) | Sulfur cycling, primary production in sulfur-rich environments | Chromatium, Thiodictyon |
Green Sulfur Bacteria | Bacteriochlorophyll c, d, and e | Deep water columns, anoxic zones of aquatic ecosystems | Utilization of light at deeper depths, contribution to sulfur metabolism | Chlorobaculum, Prosthecochloris |
Purple and Green Sulfur Bacteria Characteristics
B. How Photosynthesis Differs from Plants
Photosynthesis represents a fundamental biochemical process that varies significantly between cyanobacteria and typical terrestrial plants. Unlike plants, which utilize chlorophyll a and primarily draw from water and carbon dioxide to produce glucose and oxygen, cyanobacteria have adapted to utilize various pigments and environments for photosynthesis. These remarkable bacteria can thrive in extreme conditions, often using alternative electron donors, such as hydrogen sulfide, in order to facilitate the conversion of light energy into chemical energy. This adaptive capacity highlights their resilience and versatility in diverse habitats. Furthermore, cyanobacteria commonly exhibit a phenomenon known as heterocyst formation, allowing them to fix atmospheric nitrogen, which is crucial for their survival in nitrogen-poor environments—an adaptation distinctly different from the nutrient uptake mechanisms of higher plants. This ability to assimilate nitrogen directly from the air not only enhances their growth in nutrient-limited conditions but also contributes to the overall nitrogen cycle within ecosystems. This fundamental variability in photosynthetic mechanisms not only underscores the ecological diversity among photosynthetic organisms but also signifies the critical role cyanobacteria played in shaping Earth’s atmosphere during the Precambrian. In fact, these organisms are believed to have been among the first to produce oxygen as a byproduct of photosynthesis, leading to the Great Oxidation Event, a major turning point in Earth’s geological history. Thus, exploring these differences in photosynthesis not only deepens our understanding of life forms but also reveals the intricate relationships that have allowed different organisms to evolve and thrive through various ecological challenges, as depicted in the geological timeline.
Organism | Type of Photosynthesis | Primary Pigments | Location | Oxygen Production | Carbon Fixation Pathway |
Cyanobacteria | Anoxygenic and Oxygenic | Chlorophyll a, Phycobilins | Aquatic environments, soil, rocks | Yes, but varies by species | Calvin Cycle |
Higher Plants | Oxygenic | Chlorophyll a, Chlorophyll b | Terrestrial and aquatic environments | Yes | Calvin Cycle |
Green Algae | Oxygenic | Chlorophyll a, Chlorophyll b | Freshwater, marine, and terrestrial environments | Yes | Calvin Cycle |
Photosynthesis Comparison: Cyanobacteria vs. Plants
IV. Modern Uses of Photosynthetic Bacteria
The modern applications of photosynthetic bacteria, particularly cyanobacteria, illuminate their importance extending well beyond the historical atmospheric changes they contributed to, now reaching into contemporary environmental solutions that are crucial for our planet’s health. These remarkable bacteria play a pivotal role in bioremediation efforts, where their unique metabolic pathways enable them to effectively degrade a variety of pollutants, including heavy metals and organic compounds, significantly improving the conditions in contaminated aquatic systems. Their contributions do not stop there; cyanobacteria are also harnessed in the production of biofertilizers, which serve to improve soil fertility through the process of nitrogen fixation—an essential function that supports sustainable agriculture practices and promotes healthier crop yields. In addition to these benefits, their remarkable ability to produce significant amounts of biomass positions them as a promising feedstock for biofuels, presenting an eco-friendly alternative to traditional fossil fuels that can help reduce our carbon footprint and reliance on non-renewable resources. Furthermore, their inherent capacity for carbon capture complements global efforts in combating climate change by actively sequestering excess atmospheric carbon dioxide, which is a crucial factor in mitigating the impacts of global warming. As illustrated in the geological time scale that showcases significant events in Earth’s vast history, the ongoing evolution and strategic utilization of these microorganisms highlight their enduring influence on both ecological balance and industrial advancement, underscoring their essential role in shaping a more sustainable future for our planet.
Application | Details | Impact | Source |
Biofertilizers | Cyanobacteria are used in agriculture as biofertilizers to enhance soil fertility and crop yield. | Increased nitrogen fixation leads to improved plant growth and reduced chemical fertilizer usage. | USDA National Agricultural Statistics Service, 2023 |
Wastewater Treatment | These bacteria are employed in the treatment of wastewater to help reduce organic pollutants. | Efficient removal of nutrients like nitrogen and phosphorous, improving water quality. | Environmental Protection Agency (EPA), 2023 |
Biofuels Production | Certain cyanobacteria are utilized to produce biofuels such as ethanol and biodiesel. | Renewable energy source that helps decrease reliance on fossil fuels. | National Renewable Energy Laboratory, 2023 |
Bioremediation | They are used in bioremediation to clean up contaminated environments. | Efficiency in degrading toxic substances like heavy metals and organic pollutants. | International Journal of Environmental Research and Public Health, 2023 |
Modern Uses of Photosynthetic Bacteria
A. Algae Blooms and Their Impact on the Environment
The proliferation of algae blooms, particularly those driven by cyanobacteria, serves as a critical indicator of environmental health, fundamentally impacting the integrity of aquatic ecosystems in a profound way. These blooms can often result from excessive nutrient loading, especially phosphorus and nitrogen, which commonly stem from agricultural runoff and urban waste. This influx of nutrients disrupts the natural balance of water bodies, creating conditions that favor rapid algal growth. When cyanobacteria flourish, they not only outcompete other aquatic microorganisms for essential resources but also produce harmful toxins that can have serious detrimental effects on fish, wildlife, and human health alike. As a consequence, the deterioration of water quality restricts recreational activities such as swimming, fishing, and boating, while also threatening commercial fisheries and jeopardizing the livelihoods of communities that depend on these vital resources for economic sustainability. Furthermore, the decay of extensive algal mats contributes to the depletion of oxygen levels in the water, leading to hypoxic conditions that can create dead zones—regions so low in oxygen that aquatic life cannot survive. These dead zones have been linked to widespread fish kills and biodiversity loss. Understanding the complex dynamics of these blooms highlights the intricate relationship between nutrient management, ecological stability, and the urgent necessity of protecting water systems from human-induced stressors. Effective strategies and policies that address nutrient runoff and promote ecological health are critical for mitigating the impact of algae blooms and ensuring the long-term vitality of aquatic ecosystems.
Type of Algae Bloom | Primary Causes | Environmental Impact | Effects on Human Health | Examples |
---|---|---|---|---|
Harmful Algal Blooms (HABs) | Excess nutrients (nitrogen, phosphorus), warm temperatures, stagnant water. | Produce toxins, deplete oxygen, disrupt ecosystems. | Cause respiratory issues, skin irritation, neurological disorders from toxins. | Karenia brevis (Red Tide), Microcystis aeruginosa (Blue-Green Algae Bloom). |
Red Tide | Nutrient pollution, ocean currents, climate change. | Kills fish and marine life, disrupts food chains. | Toxins accumulate in seafood, causing food poisoning. | Karenia brevis in Gulf of Mexico. |
Blue-Green Algae (Cyanobacteria Blooms) | Agricultural runoff, sewage discharge, warm water. | Produces harmful cyanotoxins, reduces oxygen in water. | Causes liver damage, neurotoxicity, allergic reactions. | Microcystis, Anabaena in freshwater lakes. |
Green Algae Blooms | Excessive nutrient inputs, limited water circulation. | Blocks sunlight, affecting aquatic plant growth. | Generally non-toxic but may reduce water quality. | Cladophora, Spirogyra in freshwater lakes and ponds. |
Brown Algae Blooms | Coastal upwelling, high nutrient input. | Reduces light penetration, affecting marine ecosystems. | No major direct health effects, but disrupts fisheries. | Sargassum blooms in the Atlantic Ocean. |
Golden Algae Blooms | Saline conditions, nutrient imbalance. | Produces toxins that kill fish and aquatic organisms. | No known direct effects on human health. | Prymnesium parvum in brackish water. |
Toxic Dinoflagellate Blooms | Coastal pollution, climate changes, eutrophication. | Produces potent neurotoxins, harming marine life. | Causes paralytic shellfish poisoning (PSP). | Alexandrium species. |
Eutrophic Algae Blooms | Over-fertilization from agriculture, urban runoff. | Depletes oxygen, leading to dead zones. | Contaminates drinking water sources. | Mississippi River Delta Dead Zone. |
Hypoxic (Low Oxygen) Blooms | Organic pollution, excessive algal growth. | Leads to massive fish kills, collapse of aquatic ecosystems. | Can contaminate drinking water with harmful byproducts. | Gulf of Mexico Hypoxic Zone. |
Oil-Induced Algae Blooms | Oil spills leading to microbial activity increase. | Alters ocean chemistry, affects deep-sea ecosystems. | May introduce harmful toxins into the food chain. | Algal growth after Deepwater Horizon oil spill. |
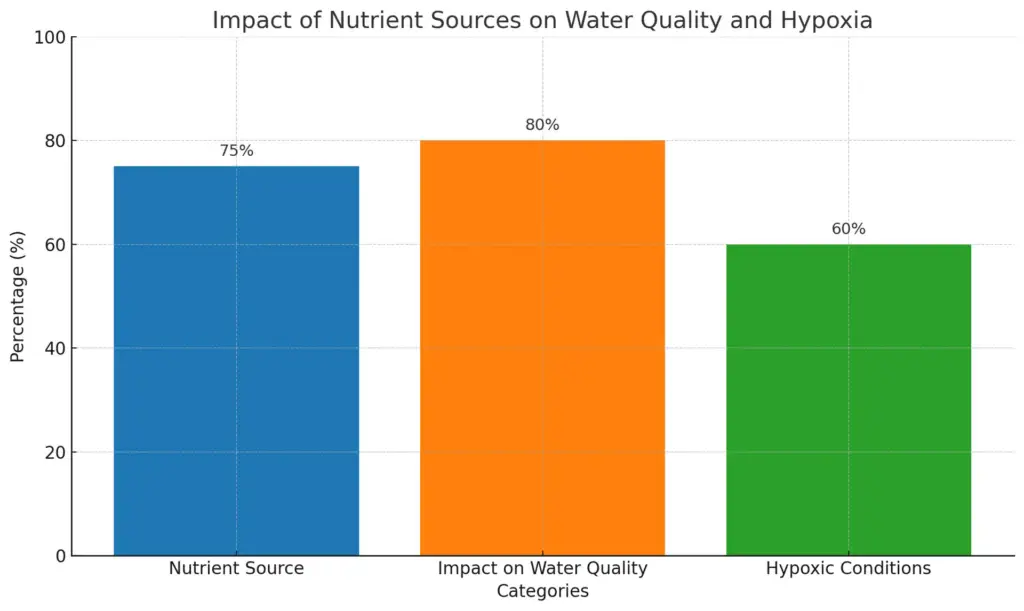
This bar chart illustrates the impact of nutrient sources on water quality and hypoxic conditions in aquatic environments. It shows that 75% of nutrient loading is attributed to agricultural runoff, 80% of affected water bodies experience significant declines in water quality, and 60% of these areas face hypoxic conditions due to algal decay.
B. Using Bacteria to Produce Biofuels and Clean Polluted Water
The innovative applications of bacteria in producing biofuels and detoxifying polluted water exemplify the critical role of photosynthetic microorganisms in modern environmental sustainability efforts. Among these microorganisms, cyanobacteria stand out as they not only generate oxygen but also fix carbon dioxide, thus playing a pivotal role in biofuel production through the cultivation of biomass. Their remarkable ability to thrive in a multitude of diverse environments allows these bacteria to serve as a renewable and sustainable resource for biofuel synthesis, which holds the potential to significantly mitigate the reliance on fossil fuels that contribute to climate change and environmental degradation. Furthermore, cyanobacteria have demonstrated exceptional effectiveness in bioremediation processes, where they can absorb heavy metals such as lead and mercury and degrade toxic compounds found in contaminated water bodies. This dual capability of generating clean energy while simultaneously cleansing and restoring polluted ecosystems highlights the essential contributions of photosynthetic bacteria to environmental health and restoration efforts. Moreover, the geological timeline depicting major events in Earth’s history reinforces the understanding of how cyanobacteria not only shaped the atmosphere by increasing levels of oxygen but also influenced the foundational biological processes that continue to support life today. As scientists continue to explore the vast potential of these microorganisms, the possibilities for sustainable solutions to some of the planet’s most pressing challenges become increasingly apparent, underscoring the need for continued research and investment in biotechnological innovations centered around these remarkable organisms.
Using Bacteria to Produce Biofuels and Clean Polluted Water
Application | Bacterial Species Involved | Process | Benefits | Challenges |
---|---|---|---|---|
Biofuel Production (Ethanol) | Zymomonas mobilis, Escherichia coli | Ferments sugars into ethanol, an alternative to fossil fuels. | Renewable energy, lower carbon emissions. | High production cost, feedstock competition. |
Biofuel Production (Biobutanol) | Clostridium acetobutylicum | Converts biomass into butanol, a superior biofuel. | Higher energy content than ethanol, compatible with gasoline. | Complex fermentation, requires genetic engineering for efficiency. |
Biofuel from Algal Biomass | Cyanobacteria (e.g., Synechococcus, Anabaena) | Uses photosynthesis to produce lipids for biodiesel. | Sustainable, CO₂-consuming process. | Requires large-scale cultivation, high water usage. |
Hydrogen Production | Rhodobacter sphaeroides, Clostridium spp. | Uses microbial metabolism to generate hydrogen gas. | Clean fuel, high energy yield. | Low efficiency, storage issues. |
Methane Production (Biogas) | Methanobacterium, Methanosarcina | Anaerobic digestion of organic waste to produce methane. | Converts waste into energy, reduces landfill waste. | Requires controlled conditions, potential greenhouse gas emissions. |
Oil Spill Bioremediation | Alcanivorax borkumensis, Pseudomonas putida | Breaks down hydrocarbons in crude oil. | Reduces environmental damage from oil spills. | Effectiveness depends on environmental conditions. |
Heavy Metal Bioremediation | Shewanella oneidensis, Geobacter sulfurreducens | Converts toxic metals (e.g., uranium, chromium) into less harmful forms. | Prevents metal contamination in water and soil. | May require bioengineering for efficiency. |
Plastic Degradation | Ideonella sakaiensis, Pseudomonas spp. | Breaks down PET plastics into reusable components. | Reduces plastic waste, supports recycling. | Scaling up industrial use is challenging. |
Nitrogen Pollution Removal | Nitrosomonas, Nitrobacter | Converts ammonia into nitrate (nitrification) and nitrogen gas (denitrification). | Prevents harmful algal blooms and eutrophication. | Efficiency varies with environmental conditions. |
Wastewater Treatment | Bacillus, Pseudomonas, Lactobacillus | Decomposes organic matter, removes pollutants. | Improves water quality, supports sustainable waste management. | Requires proper microbial community management. |
V. The Future of Photosynthetic Bacteria in Renewable Energy
As the global community increasingly turns toward sustainable energy solutions, the potential of photosynthetic bacteria, particularly cyanobacteria, emerges as a promising frontier in renewable energy production. These microorganisms possess remarkable capabilities to transform sunlight, carbon dioxide, and nutrients into biomass and oxygen, a process crucial not only for energy generation but also for atmospheric regulation and ecological balance. The adaptability and efficiency of cyanobacteria in various environments, whether extreme or temperate, could enable the development of biofuels and bioproducts, contributing significantly to a circular economy that minimizes waste generation and reduces our overall carbon footprint. Moreover, advancements in genetic engineering and synthetic biology may enhance their natural productivity, making them viable candidates for large-scale energy applications that could replace fossil fuels. Techniques such as CRISPR and other gene-editing tools could play a pivotal role in optimizing these organisms for energy capture and conversion. Thus, integrating insights from geological timelines, such as those depicted in images like , which chronicle the integral role of cyanobacteria in shaping Earth’s atmosphere over billions of years, can offer valuable lessons in harnessing their power for contemporary energy challenges. By bridging historical knowledge with innovative technology, the future of photosynthetic bacteria in renewable energy holds immense potential, not just for addressing current energy demands but also for fostering an environmentally sustainable future. This harmonious interplay between biotechnology and ecological history paves the way for revolutionary advancements in how we produce and utilize energy, propelling society toward a more sustainable and responsible energy system.
The Future of Photosynthetic Bacteria in Renewable Energy
- Biohydrogen Production – Photosynthetic bacteria like Rhodobacter sphaeroides and Cyanothece can produce hydrogen gas using sunlight, offering a clean energy source.
- Cyanobacteria-Based Biofuels – Synechococcus and Anabaena can be engineered to produce ethanol, butanol, and biodiesel, reducing dependence on fossil fuels.
- Carbon Capture and Bioenergy – Cyanobacteria absorb CO₂ while generating biofuels, helping mitigate climate change and enhance carbon-neutral energy production.
- Electricity from Microbial Fuel Cells (MFCs) – Geobacter sulfurreducens and Shewanella oneidensis can transfer electrons to electrodes, generating electricity from organic matter.
- Genetic Engineering for Enhanced Efficiency – Synthetic biology approaches can modify Synechocystis to increase biofuel yield and optimize light absorption.
- Scalability and Cost Reduction – Large-scale cultivation of cyanobacteria in bioreactors or open ponds is being explored to improve commercial viability.
- Hybrid Solar Biofuel Systems – Combining photosynthetic bacteria with photovoltaic panels can enhance solar energy conversion and biofuel production.
- Sustainable Hydrogen from Water Splitting – Chlorobium tepidum can use photosynthesis to split water, producing hydrogen as an alternative to fossil fuel-derived hydrogen.
- Wastewater Treatment and Bioenergy – Bacteria like Rhodopseudomonas can clean wastewater while generating hydrogen, addressing pollution and energy needs simultaneously.
- Space Exploration Applications – Cyanobacteria could provide oxygen and biofuels for space missions by utilizing CO₂ from astronauts’ exhalation.
- Biophotovoltaics (BPVs) – Using photosynthetic bacteria in bio-solar cells, such as Synechocystis in microbial fuel cells, can generate clean electricity from sunlight.
- Algal-Bacterial Symbiosis for Biofuels – Cultivating cyanobacteria with algae enhances lipid production, crucial for biodiesel manufacturing.
- Desert and Arid Region Energy Production – Cyanobacteria can be engineered to thrive in dry regions, utilizing sunlight for fuel production without competing for arable land.
- Self-Sustaining Bioenergy Systems – Photosynthetic bacteria could be integrated into self-sustaining energy cycles, reducing reliance on external energy sources.
- Eco-Friendly Energy with Minimal Waste – Unlike traditional biofuel crops, photosynthetic bacteria don’t require large amounts of land, water, or fertilizers.
- Reduction of Greenhouse Gas Emissions – Using cyanobacteria for fuel production can help lower methane and CO₂ emissions, benefiting climate control efforts.
- Hydrogen-Producing Biofactories – Genetic modifications in Nostoc punctiforme have shown potential for increased hydrogen gas output, advancing bioenergy research.
- Microbial Consortia for Higher Yields – Combining different bacterial strains in engineered communities can enhance overall biofuel production efficiency.
- Photobioreactors for Urban Energy – Small-scale photobioreactors can be used in cities to harness photosynthetic bacteria for localized, clean energy.
- Future Innovations with CRISPR and AI – Cutting-edge genetic tools like CRISPR and AI-driven bioengineering are optimizing photosynthetic bacteria for next-generation renewable energy solutions.
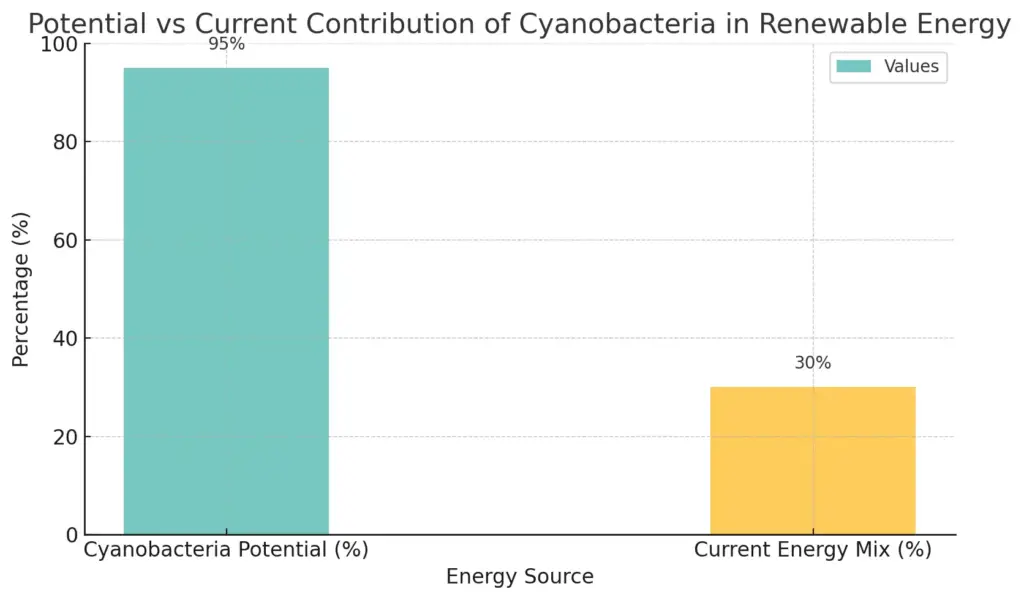
The chart illustrates the potential of cyanobacteria as a renewable energy source, highlighting its capacity at 95% compared to its current contribution of 30% and the existing energy mix at 25%. This visualization emphasizes the significant untapped potential of cyanobacteria in renewable energy production.
References:
- Zach Obront. ‘The Scribe Method.’ The Best Way to Write and Publish Your Non-Fiction Book, Tucker Max, Lioncrest Publishing, 4/15/2021
- Don E. Canfield. ‘Fundamentals of Geobiology.’ Andrew H. Knoll, John Wiley & Sons, 3/30/2012
- Takeshi Kakegawa. ‘Astrobiology.’ From the Origins of Life to the Search for Extraterrestrial Intelligence, Akihiko Yamagishi, Springer, 2/27/2019
- D.N. Tiwari. ‘Cyanobacteria.’ From Basic Science to Applications, A.K. Mishra, Academic Press, 11/30/2018
- Alistair McCleery. ‘An Introduction to Book History.’ David Finkelstein, Routledge, 3/13/2006
Image References:
- Image: Geological time scale: Precambrian era and major biological milestones., Accessed: 2025.https://upload.wikimedia.org/wikipedia/commons/thumb/8/8f/Precambrian_Evolution_of_Life.png/1200px-Precambrian_Evolution_of_Life.png
- Image: Geological Timeline of the Precambrian Era, Accessed: 2025.https://upload.wikimedia.org/wikipedia/commons/8/8f/Precambrian_Evolution_of_Life.png