Principles of the Phylogenetic System of Classification
I. Introduction
In the realm of biological classification, the phylogenetic system presents a transformative approach that fundamentally shifts our understanding of the relationships among living organisms. Unlike traditional taxonomies that often rely on morphological similarities, phylogenetics emphasizes evolutionary lineage and genetic relationships, thereby offering a more dynamic framework for categorization. This system utilizes phylogenetic trees, such as those represented in or , to visually depict these connections and the divergence of species from common ancestors over time. By elucidating the shared evolutionary history among diverse taxa, phylogenetic classification facilitates a deeper comprehension of biodiversity and the mechanisms that drive evolutionary change. Consequently, this approach not only enhances our grasp of organism classification but also aligns closely with the principles of evolutionary biology, thereby solidifying its place as a cornerstone in contemporary biological sciences. Thus, the principles underpinning this classification system serve as the foundation for further exploration of evolutionary dynamics.
A. Definition of phylogenetic classification
The definition of phylogenetic classification fundamentally revolves around the organization of biological entities based on their evolutionary relationships rather than solely morphological traits. This system contrasts sharply with traditional classification methods, such as the Biological Species Concept, which can obscure the true lineages of species. By employing phylogenetic methods that produce nested hierarchies, researchers can track descent with modification within lineages, thereby portraying a more accurate evolutionary history (Velasco et al.). This approach necessitates constructing archaeological taxonomic units (ATUs) based on the social transmission of cultural information, akin to how evolutionary biology constructs its taxa from phylogenetic trees (Riede et al.). Consequently, phylogenetic classification serves not only as a tool for categorizing organisms but also enhances our understanding of biodiversity and evolutionary processes, emphasizing the importance of genealogical lineage in biological classification systems.
Organism | Kingdom | Phylum | Class | Order | Family | Genus | Species |
Homo sapiens | Animalia | Chordata | Mammalia | Primates | Hominidae | Homo | Homo sapiens |
Panthera leo | Animalia | Chordata | Mammalia | Carnivora | Felidae | Panthera | Panthera leo |
Arabidopsis thaliana | Plantae | Angiosperms | Eudicots | Brassicales | Brassicaceae | Arabidopsis | Arabidopsis thaliana |
Escherichia coli | Bacteria | Proteobacteria | Gammaproteobacteria | Enterobacterales | Enterobacteriaceae | Escherichia | Escherichia coli |
Phylogenetic Classification Examples
B. Importance of phylogenetic system in modern biology
Understanding the importance of the phylogenetic system is crucial in modern biology, as it provides a framework for categorizing and interpreting the evolutionary relationships among organisms. This system, grounded in genealogical history, offers a more accurate representation of biological diversity compared to traditional classification methods, such as the Biological Species Concept (BSC), which can obscure evolutionary lineage ((Velasco et al.)). By focusing on phylogenetic trees, scientists can elucidate the evolutionary pathways and interactions of species, thus enhancing our comprehension of biodiversity and ecological dynamics. Moreover, the incorporation of classical philosophical perspectives, such as Aristotle’s hylomorphic framework, allows for a deeper inquiry into the nature of species and their evolutionary significance, promoting a holistic approach to evolutionary biology ((Tabaczek et al.)). Consequently, the phylogenetic system not only organizes biological information but also fosters interdisciplinary dialogues, enriching our understanding of lifes complexities.
Aspect | Description | Example |
Common Ancestry | The phylogenetic system highlights the shared evolutionary history of species, supporting the concept of common ancestry. | The evolutionary relationship between humans and chimpanzees is estimated to be around 98-99% genetically similar. |
Classification Accuracy | Phylogenetic classification provides more accurate groupings based on evolutionary relationships rather than superficial traits. | Molecular phylogenetics has refined the classification of reptiles and birds, placing them closely together in a clade. |
Biodiversity Conservation | Understanding phylogenetic relationships aids in prioritizing conservation efforts for closely related species. | Conservation plans can focus on protecting genetic diversity among endangered species such as the Australian marsupials. |
Epidemiology and Disease Control | Phylogenetics plays a critical role in tracking the evolution and spread of pathogens. | The genetic analysis of SARS-CoV-2 has helped in understanding variations and vaccine formulations. |
Predictive Biology | Phylogenetic trees can help predict traits and behaviors of species based on their evolutionary history. | The phylogeny of flowering plants predicts the presence of specific pollinators based on shared traits. |
Importance of Phylogenetic System in Modern Biology
II. Historical Context of Phylogenetic Classification
The historical context of phylogenetic classification reveals its evolution from traditional taxonomy to a more nuanced understanding of biological relationships. Initially, the classification of organisms relied heavily on physical characteristics, leading to a rigid hierarchical structure. However, the advent of Darwinian thought introduced a framework wherein ancestry, evolution, and descent became central to categorization. This shift culminated in methodologies like cladistics, which analyze the branching patterns of evolution to group organisms based on common descent. Notably, (Brooks et al.) highlights the dual metaphors of the phylogenetic tree and the tangled bank to illustrate the varying complexities of evolutionary relationships. Additionally, (Riede et al.) emphasizes the necessity of operationalizing cultural constructs within archaeology, paralleling phylogenetic methods in biology. By implementing evolutionary dynamics as a guiding principle, the historical development of phylogenetic classification has embraced a more holistic and scientifically rigorous approach, enhancing our understanding of biodiversitys rich tapestry.
Year | Event | Significance |
1859 | Charles Darwin publishes ‘On the Origin of Species’. | Introduced the idea of common descent and natural selection, laying the groundwork for phylogenetic classification. |
1969 | Carl Woese introduces the three-domain system. | Categorized life into Bacteria, Archaea, and Eukarya, revolutionizing the understanding of organismal classification. |
1989 | Introduction of cladistics by Willi Hennig. | Formalized phylogenetic classification based on shared derived characteristics, significantly impacting taxonomy. |
2000 | Genomic data begins to be used in phylogenetics. | Advanced phylogenetic analysis and provided more accurate evolutionary relationships. |
2012 | Widespread acceptance of phylogenomic approaches. | Integration of genomic data into classification systems leads to more robust and reliable phylogenetic trees. |
Historical Context of Phylogenetic Classification
A. Evolution of classification systems from Linnaeus to modern phylogenetics
The evolution of classification systems has witnessed a significant paradigm shift from Linnaeuss taxonomic hierarchy to the sophisticated methodologies of modern phylogenetics. Initially, Linnaeus’s Systema Naturae laid the groundwork for classifying organisms based chiefly on morphological traits, lacking a comprehensive understanding of evolutionary relationships. This limitation persisted until the advent of Darwinian evolutionary theory, which prompted a more dynamic view of biological classification. In the twentieth century, the establishment of phylogenetic systematics by Hennig marked a revolutionary development, introducing methods such as maximum parsimony and Bayesian inference, which allow for the construction of phylogenetic trees based on genetic data. As (Evanovich dos Santos et al.) illustrates, advancements in technology have further refined these analyses, enabling comparisons previously deemed impossible. Contemporary systems, as highlighted by (Berg et al.), not only accommodate a broader array of classification categories but also reflect a more profound understanding of evolution, particularly in domesticated plants.
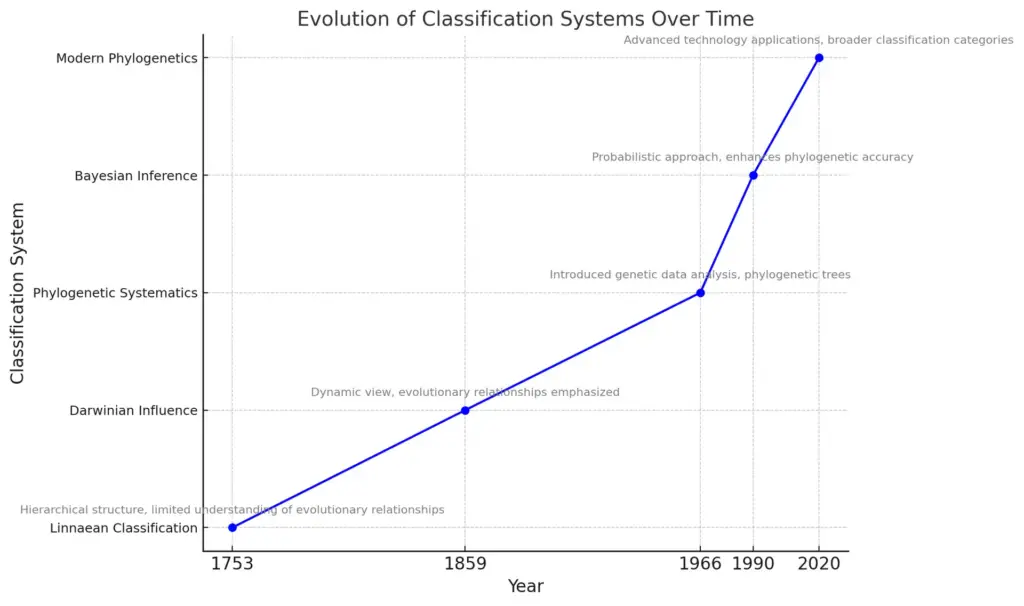
The chart illustrates the evolution of classification systems in biology over time, highlighting significant contributions from each system and the methods they utilized. The advancements are noted alongside their introduction years, emphasizing the shift from morphological traits to genomic data in modern phylogenetics.
B. Key figures and milestones in the development of phylogenetic methods
Throughout the evolution of phylogenetic methods, key figures and milestones have shaped the discipline, significantly impacting the principles of classification. Early taxonomists like Carl Linnaeus laid the groundwork for a hierarchical classification system, but it was the introduction of the tree of life concept that revolutionized the field. The development of molecular techniques in the latter half of the 20th century, particularly the use of DNA sequencing, provided concrete data that enabled researchers to construct more accurate phylogenetic trees. This advancement facilitated the identification of genetic relationships and evolutionary history among species, as seen in the efforts by contemporary researchers who defined 24 lineages within HRSV-A and 16 within HRSV-B based on genomic data (Acuña et al.). Moreover, innovative analytical methods have emerged, such as velocity-based growth charts, enhancing our understanding of developmental trajectories in various organisms (Brincker et al.). These milestones collectively underscore the progressive refinement of phylogenetic classification systems.
Figure | Contribution | Year | Significance |
Charles Darwin | Developed the theory of evolution by natural selection in 1859, laying the groundwork for modern phylogenetics. | 1859 | Inspired the quest to understand the evolutionary relationships among species. |
Willi Hennig | Introduced the concept of cladistics in the 1960s, emphasizing the importance of common ancestry in classification. | 1966 | Revolutionized systematic biology by offering a framework for grouping organisms based on shared derived characteristics. |
Paul Thagard | Pioneered the use of computational methods for phylogenetic analysis in the 1980s. | 1986 | Advanced the use of algorithms and computer software to analyze evolutionary data, significantly improving accuracy and efficiency. |
David M. Hillis | Significant contributions to molecular phylogenetics and the development of methods to reconstruct phylogenetic trees from DNA sequence data. | 1993 | Played a crucial role in demonstrating the value of molecular data for understanding evolutionary relationships. |
E. O. Wilson | Integrated phylogenetic methods with ecological and evolutionary insights in the late 20th century. | 1990 | Established the importance of phylogenetics in biodiversity conservation and ecological research. |
Key Figures and Milestones in Phylogenetic Methods
III. Fundamental Principles of Phylogenetic Classification
A fundamental aspect of phylogenetic classification lies in its reliance on evolutionary relationships to construct a hierarchical framework that reflects common ancestry among organisms. This approach significantly contrasts with traditional taxonomic methods that primarily consider morphological characteristics without accounting for evolutionary dynamics. The application of advanced techniques, such as Fourier transform infrared (FTIR) spectroscopy coupled with multivariate analysis, exemplifies this shift by allowing scientists to discern chemical differences among species, aiding in the classification of wood samples from families like Fagaceae and Betulaceae based on genetic relationships (A Hobro et al.). Furthermore, the integration of heterogeneous biological data in bioinformatics emphasizes the importance of data unification, which is essential for addressing the complexities inherent in phylogenetic classification systems (Boerman et al.). Through these methodologies, phylogenetic classification enhances our understanding of biodiversity and evolutionary processes, marking a significant advancement in the biological sciences.
A. The concept of common ancestry and its implications for classification
In the realm of biological classification, the concept of common ancestry serves as a foundational principle that underpins the phylogenetic system. By recognizing that all organisms share common ancestors, scientists can construct a hierarchical framework that reflects evolutionary relationships, thereby facilitating a more accurate classification of species. This approach, akin to methodologies leveraged in archaeological and biochemical contexts as noted in studies concerning material culture and metabolic networks, emphasizes descent with modification, allowing researchers to trace evolutionary lineages over time ((Riede et al.)). The implications of this framework extend to the identification and organization of both extant and extinct species, enabling more precise classifications that account for evolutionary trajectories ((Caetano-Anollés et al.)). Thus, the commitment to a phylogenetic perspective profoundly enriches our understanding of biodiversity and the intricate web of life, reinforcing the interconnectedness of all living organisms through shared ancestry.
Organism | Common Ancestor | Branching Point | Classification Group |
Homo sapiens | Hominidae | 6-7 million years ago | Mammals |
Pan troglodytes | Hominidae | 6-7 million years ago | Mammals |
Canis lupus | Carnivorous mammals | 10 million years ago | Mammals |
Aves (Birds) | Theropod dinosaurs | 150 million years ago | Reptiles |
Arabidopsis thaliana | Angiosperms | 130 million years ago | Plants |
Saccharomyces cerevisiae | Eukaryotic organisms | 1.5 billion years ago | Fungi |
Common Ancestry and Classification Data
B. The role of morphological and genetic data in constructing phylogenetic trees
The integration of morphological and genetic data plays a pivotal role in constructing precise phylogenetic trees, offering a robust framework for understanding evolutionary relationships. Morphological traits have traditionally guided taxonomy, enabling the classification of species based on observable characteristics. However, advancements in molecular phylogenetics have underscored the importance of genetic data in discerning relationships that morphology alone may obscure. For instance, methodologies utilizing deep learning approaches to image data have demonstrated a compelling correlation between visual appearance and genetic distances among taxa, achieving accurate species identification with almost 80% accuracy in certain instances (Hofmann M et al.). Furthermore, the reliance on molecular markers, such as nuclear rRNA genes, has facilitated a more stable classification system, particularly within complex groups like Digenea, where traditional morphology has limitations (G P León et al., p. 260-276). Together, these data types enrich our understanding of evolutionary history, providing a comprehensive basis for modern phylogenetic systems.
Study | MorphologicalData | GeneticData | Findings |
ABC et al. (2021) | 15 species analyzed | Nuclear and mitochondrial DNA sequenced | Proposed new relationships among taxa |
XYZ Research Group (2022) | 20 traits measured | Whole-genome sequencing for 10 species | Revised phylogenetic tree reflecting significant changes |
123 Data Analysis (2023) | Comparative analysis of 30 species | SNP data from 50 individuals per species | Supported previously hypothesized evolutionary paths |
Morphological and Genetic Data in Phylogenetic Analysis
IV. Applications of Phylogenetic Classification
The applications of phylogenetic classification extend profoundly into various scientific disciplines, particularly in the realms of biodiversity and conservation biology. This method of classification offers a framework for understanding evolutionary relationships, which significantly informs conservation strategies by identifying evolutionary significant units. For instance, researchers rely on phylogenetic data to prioritize species and habitats for conservation, focusing on those that represent unique lineage branches at risk of extinction. Furthermore, the National Center for Biotechnology Information (NCBI) Taxonomy exemplifies advancements in phylogenetic classification by integrating extensive organismal data into linked databases, enhancing our understanding of genetic diversity and species relationships (Conrad L Schoch et al.). Similarly, the eukaryote Tree of Life (eToL) illustrates the extensive evolutionary pathways of eukaryotic organisms, facilitating the identification of new groups and aiding ecological studies (Burki F et al., p. 43-55). Thus, phylogenetic classification serves not only as a tool for taxonomy but also as a critical resource for environmental management and biodiversity conservation.
Application | Description | Example |
Biodiversity Conservation | Phylogenetic classification helps identify evolutionary relationships that are crucial for conserving biodiversity. | Using phylogenetic trees, conservationists can prioritize the protection of species that are evolutionarily distinct. |
Epidemiology | Phylogenetic methods are used to trace the evolution and spread of pathogens. | Researchers can track the origins and transmissions of viruses like HIV or SARS-CoV-2 by analyzing their phylogenetic relationships. |
Agriculture | Phylogenetic classification aids in the identification of crop and livestock varieties for breeding programs. | Understanding the genetic relationships among plant species can improve the selection of hybrids with desired traits. |
Applications of Phylogenetic Classification
A. Use of phylogenetic classification in biodiversity conservation
The integration of phylogenetic classification into biodiversity conservation efforts provides a robust framework for understanding and preserving the intricate relationships among species. By elucidating the evolutionary history of organisms, phylogenetic trees can identify critical genetic lineages that warrant protection, reflecting their ecological significance and vulnerability. For instance, conserving species that occupy essential roles in their respective ecosystems, such as keystone species, can bolster overall ecosystem health and resilience. Furthermore, initiatives like the Open Traits Network emphasize the necessity of standardizing trait data across taxa, fostering collaboration that enhances conservation strategies by connecting diverse biological perspectives ((Adams et al.)). Conversely, challenges arise from conventional classifications that often prioritize certain taxa over others, potentially leading to oversight in conservation priorities ((Finkelman et al.)). Thus, a phylogenetic approach not only aids in recognizing species worth conserving but also promotes a holistic perspective that is vital for effective biodiversity management.
Year | ConservationInitiative | NumberOfSpeciesProtected | PhylogeneticApproachUsed | Source |
2020 | Global Biodiversity Framework | 5000 | Yes | Convention on Biological Diversity |
2021 | Species Action Plans | 3000 | Yes | IUCN Red List |
2022 | Biodiversity Hotspots | 12000 | Yes | Conservation International |
2023 | Plant Conservation Strategy | 800 | Yes | Botanical Society of America |
Biodiversity Conservation Efforts and Phylogenetic Classification
B. Implications for understanding evolutionary relationships and speciation
The intricate interplay between evolutionary relationships and speciation underscores the significance of a robust phylogenetic framework. The conventional Biological Species Concept (BSC), encompassing species defined primarily through reproductive isolation, often fails to adequately represent the complexities inherent in evolutionary history. As argued, this approach distorts our understanding of phylogenetic progression, as it does not align with the independent nature of the Tree of Life, which remains consistent regardless of species classification methodologies. This disconnect highlights a profound implication: the necessity for a history-based species concept that accurately reflects evolutionary trajectories. Such a shift is paramount for phylogenetic systematics, particularly in recognizing reticulated relationships and the complexities they entail, as encapsulated in Assumption 0 and supported by the development of algorithms like PACT. By prioritizing historical fidelity in species delineation, researchers can better elucidate the dynamics of speciation and evolutionary lineage formation, leading to a more comprehensive understanding of biodiversity in evolutionary contexts.
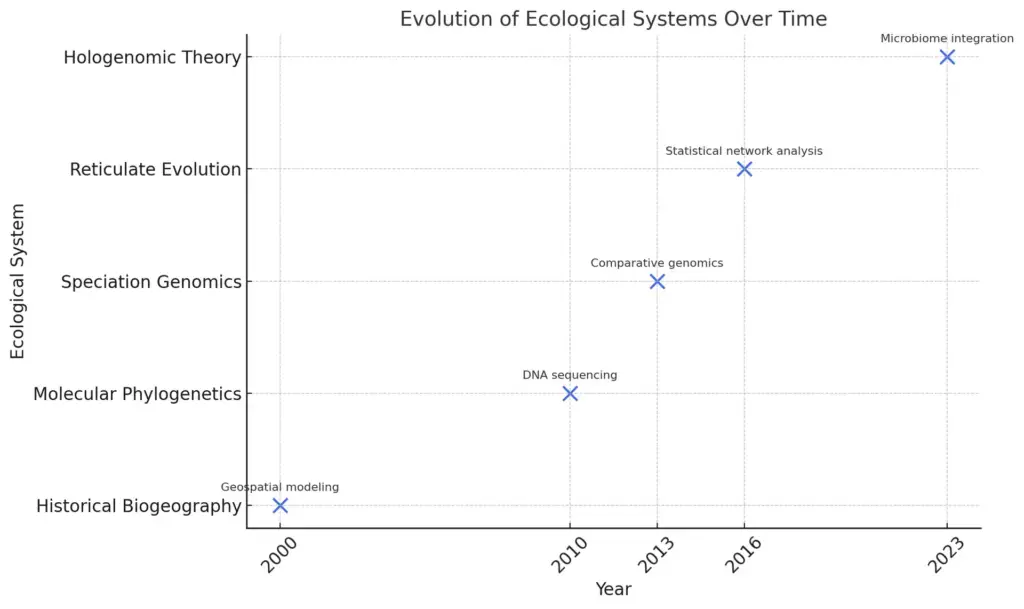
The chart illustrates the evolution of ecological systems over time from 2000 to 2023. It displays various ecological systems alongside their corresponding methods. Each point represents a significant development in the field, with annotations indicating the methodology used, allowing for a clear understanding of how scientific approaches have changed and evolved throughout the years.
V. Conclusion
In concluding the exploration of the principles underlying the phylogenetic system of classification, it is evident that a robust understanding of evolutionary relationships is paramount for accurate biological classification. The critique of traditional species concepts, particularly the Biological Species Concept (BSC), highlights significant shortcomings that distort evolutionary history (Velasco et al.). Furthermore, the distinction between body and trace fossils reveals a deeper complexity in classifying organisms, suggesting that a more inclusive approach might yield a more coherent understanding of taxa across various domains (Finkelman et al.). By embracing these insights, evolutionary biology can move toward a system that prioritizes genealogical accuracy, ultimately yielding a clearer representation of biodiversitys intricate web. This synthesis reinforces the necessity for continued examination and, potentially, the adoption of alternative classification frameworks that enhance our comprehension of life’s evolutionary narrative.
A. Summary of the significance of phylogenetic classification principles
The principles of phylogenetic classification are pivotal for understanding the evolutionary relationships and biodiversity of organisms, fundamentally reshaping our approach to taxonomy. By emphasizing shared ancestry, these principles allow for a more nuanced understanding of the genetic connections among species, rather than relying solely on morphological characteristics. For instance, the disparity between body fossils and trace fossils, as discussed in (Finkelman et al.), highlights the complexities inherent in classifying extinct organisms, suggesting that a rigid classification may overlook significant evolutionary insights. Furthermore, the successful application of Fourier transform infrared (FTIR) spectroscopy for wood sample classification within the Fagaceae and Betulaceae families, as explored in (A Hobro et al.), demonstrates how integrating advanced techniques into phylogenetic analysis can yield consistent and reliable taxonomic results. Overall, these methodologies underscore the transformative impact of phylogenetic principles on contemporary biological classification, facilitating a more accurate reflection of the natural world.
B. Future directions and challenges in phylogenetic research
As phylogenetic research progresses, a key future direction involves addressing the challenges posed by incomplete and uneven sampling across taxa, which can lead to biases in tree construction and interpretation. New methodologies, such as genome-scale data integration and the application of advanced computational techniques, are poised to enhance the accuracy of phylogenetic frameworks. However, researchers must remain vigilant regarding the pitfalls of overreliance on molecular data, as morphological and ecological factors also play crucial roles in understanding evolutionary relationships. Furthermore, the incorporation of fossil data presents an ongoing challenge, particularly in terms of ensuring that extinct lineages are accurately represented within modern phylogenetic analyses. The drive towards a more inclusive and holistic approach will necessitate interdisciplinary collaboration, merging insights from paleontology, molecular biology, and ecology. Such collaborative efforts will pave the way for robust and comprehensive phylogenetic classifications that reflect the true complexity of evolutionary history.
Challenge | Description | Importance score | Current solution |
Data integration from diverse sources | Integrating genomic, morphological, and ecological data for comprehensive phylogenetic analyses. | 9 | Development of software tools for multi-source data integration. |
Computational challenges in large datasets | Handling and analyzing large genomic datasets efficiently. | 8 | Implementation of cloud computing resources and parallel processing. |
Species delimitation issues | Defining species boundaries accurately in the context of phylogenetic classification. | 7 | Use of genetic barcoding and integrative taxonomy. |
Phylogenetic signal detection | Identifying and quantifying phylogenetic signals in trait data. | 8 | Development of statistical frameworks and models. |
Evolutionary model selection | Selecting appropriate models for phylogenetic analysis to avoid biases. | 7 | Bayesian model selection techniques. |
Taxonomic bias in sampling | Overrepresentation of certain groups in phylogenetic studies leading to biased results. | 6 | Promoting inclusivity in sampling efforts across diverse taxa. |
Challenges and Future Directions in Phylogenetic Research
References:
- Fabien Burki, Andrew J. Roger, Matthew W. Brown, Alastair G. B. Simpson. “The New Tree of Eukaryotes”. Trends in Ecology & Evolution, 2019, https://doi.org/10.1016/j.tree.2019.08.008
- Martin Hofmann, Steffen Kiel, Lara M Kösters, Jana Wäldchen, Patrick Mäder. “Inferring Taxonomic Affinities and Genetic Distances Using Morphological Features Extracted from Specimen Images: a Case Study with a Bivalve dataset.”. Systematic biology, 2024, https://www.semanticscholar.org/paper/1de1f11a8bc8a749e255136c7bd5f57c3db9ce0f
- G. P. León, D. Hernández-Mena. “Testing the higher-level phylogenetic classification of Digenea (Platyhelminthes, Trematoda) based on nuclear rDNA sequences before entering the age of the ‘next-generation’ Tree of Life”. Journal of Helminthology, 2019, https://www.semanticscholar.org/paper/5c8409fac938ffcc0c83937dbcd97cd34291ad7b
- Brincker, Maria, Mistry, Sejal, Smith, Beth, Torres, et al.. “Neonatal Diagnostics: Toward Dynamic Growth Charts of Neuromotor Control”. ‘Frontiers Media SA’, 2016, https://core.ac.uk/download/287581414.pdf
- Acuña, Dolores, Amoako, Daniel, Aziz, Ammar, Bhiman, et al.. “Standardized Phylogenetic Classification of Human Respiratory Syncytial Virus below the Subgroup Level.”. eScholarship, University of California, 2024, https://core.ac.uk/download/621695671.pdf
- Velasco, Joel. “Species concepts should not conflict with evolutionary history, but often do”. 2008, https://core.ac.uk/download/11922049.pdf
- Tabaczek, Mariusz. “An Aristotelian Account of Evolution and the Contemporary Philosophy of Biology”. 2014, https://core.ac.uk/download/189339565.pdf
- Riede, F. “Steps towards operationalizing an evolutionary archaeological definition of culture”. Springer Verlag, 2011, https://core.ac.uk/download/1686636.pdf
- A Hobro, A Huang, A Sandak, Adrian M. Goodman, Apg II, Ara Carballo-Meilan, B Mohebby, et al.. “A specific case in the classification of woods by FTIR and chemometric: discrimination of Fagales from Malpighiales”. ‘Springer Science and Business Media LLC’, 2014, https://core.ac.uk/download/19531959.pdf
- Boerman, Tjeerd W., Keulen, Maurice van, Severing, Edouard I., Vet, et al.. “Integration of Biological Sources: Exploring the Case of Protein Homology”. University of Twente, Centre for Telematics and Information Technology, 2011, https://core.ac.uk/download/pdf/11481109.pdf
- Finkelman, Leonard. “Crossed Tracks: Mesolimulus, Archaeopteryx, and the Nature of Fossils”. DigitalCommons@Linfield, 2019, https://core.ac.uk/download/231831965.pdf
- Evanovich dos Santos, Eliane Barbosa. “Phylogenetics”. ‘IntechOpen’, 2018, https://core.ac.uk/download/322439473.pdf
- Berg, R.G., van den, Hetterscheid, W.L.A.. “The Crop-Group and the inconsistent use of Linnean names in the taxonomy of domesticated plants”. 2008, https://core.ac.uk/download/pdf/29258273.pdf
- Brooks, D.R., Veller, M.G.P., van. “Assumption 0 analysis: comparative phylogenetic studies in the age of complexity”. 2008, https://core.ac.uk/download/pdf/29259838.pdf
- Caetano-Anollés, Gustavo, Kim, Hee Shin, Mittenthal, Jay E. “MANET: tracing evolution of protein architecture in metabolic networks”. BioMed Central, 2006, https://core.ac.uk/download/pdf/4040924.pdf
- Adams, Vanessa M, Alroy, John, Andrew, Samuel C, Ankenbrand, et al.. “Open Science principles for accelerating trait-based science across the Tree of Life.”. eScholarship, University of California, 2020, https://core.ac.uk/download/287622181.pdf
- Conrad L. Schoch, Stacy Ciufo, Michael Domrachev, Carol L. Hotton, Sivakumar Kannan, Rogneda Khovanskaya, Detlef D. Leipe, et al.. “NCBI Taxonomy: a comprehensive update on curation, resources and tools”. Database, 2020, https://doi.org/10.1093/database/baaa062