Ribosomes: The Molecular Machines of Protein Synthesis
I. Introduction
Ribosomes, known as the machines for protein making, have a key role in turning genetic information into usable proteins, which is vital for cell life. These structures, made up of ribosomal RNA (rRNA) and proteins, are where messenger RNA (mRNA) and transfer RNA (tRNA) work together to put amino acids into chains. The special design of ribosomes, with a big and small subunit, helps it manage different steps of translation, like starting, lengthening, and finishing the process. Learning about ribosomes not only helps us understand basic biological actions but also highlights their role in creating antibiotic medicines, as many drugs target the functions of bacterial ribosomes. As we look into the structure and functions of ribosomes, their crucial part in keeping life’s molecular functions clear becomes more noticeable, leading to further studies on their processes and significance.
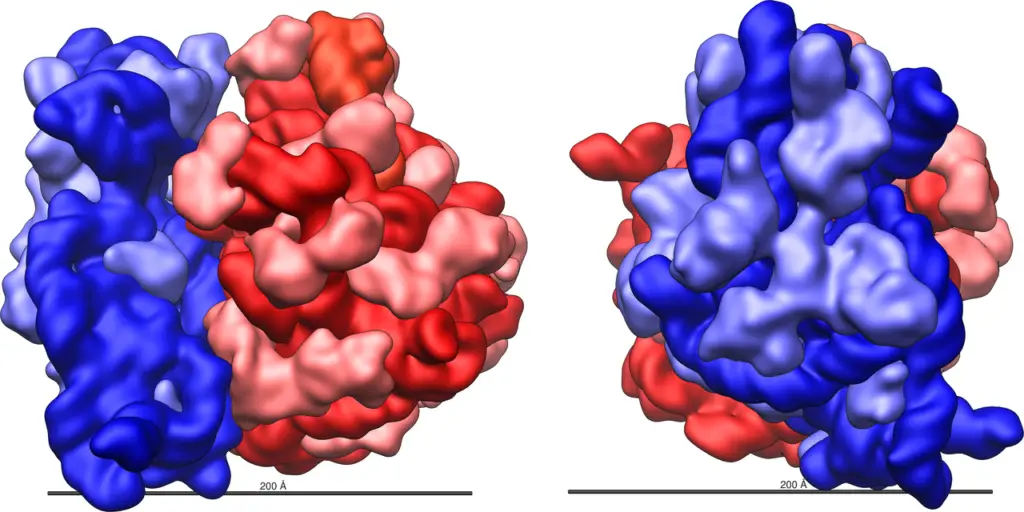
A. Definition and significance of ribosomes in cellular biology
Ribosomes are key molecular machines in cell biology that are needed for making proteins. They are made of ribosomal RNA (rRNA) and proteins and serve to translate messenger RNA (mRNA) into polypeptides, which are crucial for how cells work and keeping balance within the cell. With their complex structure, ribosomes help decode genetic information, which is vital for cell growth and adjusting to different environments. According to (Jun et al.), understanding how ribosomes work is essential for knowing bacterial biology, where they contribute to how quickly bacteria grow and control their size. Also, ribosomes are important for producing recombinant proteins in industrial uses, making it necessary to study how they respond to stress and manage resources, as pointed out in (Bulović et al.). This illustrates their importance in both basic biology and biotechnological progress, showing how much ribosomes influence life at a molecular level.
B. Overview of protein synthesis and its importance
The process of making proteins is a complex but important biological function that changes genetic info from mRNA into working proteins, which are key for how cells are built and operate. Ribosomes serve as the main tools that manage this process, helping to put together amino acids in the right order according to the mRNA sequence. This translation process isn’t just a straightforward line; it includes many factors and controls that help keep things accurate and efficient. For example, the roles of translation factors like eIF4E and eIF4G show how ribosomes change during the translation process, showing their adaptable nature (Chiang et al.). Additionally, the role of ribosome-inactivating proteins (RIPs) in different organisms points to the evolutionary importance of protein synthesis, especially in plants, where they contribute to both defense and growth control (Zaeytijd D et al.). Therefore, knowing how protein synthesis works is vital for understanding how cells function and creating treatment methods for diseases.
C. Purpose and scope of the essay
In looking at the complex biology of ribosomes, this essay’s aim is to explain their important role as machines for making proteins. By studying how translation works, the essay wants to show how ribosomes work with mRNA and tRNA, helping to put together polypeptides correctly. It will also discuss new techniques like ribosome profiling, which gives information about how ribosomal assembly and function change over time and space (Agirrezabala et al.). Furthermore, the essay will explore how RNA-binding proteins (RBPs) regulate translation rates, particularly their interactions with ribosomes and target mRNAs (Schreck et al.). Through this thorough study, the essay hopes to clarify not just the basic processes of protein synthesis but also their wider effects on understanding gene expression and its regulation, which is vital for progress in molecular biology and treatment methods.
II. Structure of Ribosomes
Ribosomes have a complex structure that is very important for them to work as machines to help make proteins. They are made of ribosomal RNA (rRNA) and proteins, creating two parts: a large subunit and a small subunit. This setup not only gives it strength but also helps it carry out the key job of translation. For example, the peptidyl transferase center (PTC) in the large subunit is where peptide bonds are created, and the decoding center in the small subunit makes sure that tRNA pairs correctly with mRNA. The inclusion of expansion segments, like ES30L, shows how ribosomes can change shape in eukaryotic cells, which may help them connect with certain mRNAs and regulatory proteins (Hariharan N et al.). Also, understanding how ribosomes change their structure is important for targeting antibiotics, as it shows how small molecules can stop protein synthesis, highlighting the important role of ribosomes in both cell biology and drug research (Y Polikanov et al., p. 2713-2725).
A. Composition of ribosomal RNA (rRNA) and proteins
Ribosomes, the important machines that make proteins, are complicated structures made of ribosomal RNA (rRNA) and ribosomal proteins (RPs), which together create ribosomal subunits. rRNA makes up a big part of the ribosome’s mass and is key for both keeping it stable and helping it work during translation. The differences in ribosomes lead to interesting questions about how changes in rRNA and RP make them work differently in various cells. For example, new findings show that tissue-specific expression of ribosomal parts matches with unique translational roles, affecting processes like neurogenesis and embryogenesis (Norris K et al.). Also, the makeup of the amino acids in the proteins being made is important because it connects to how fast translation happens, showing a direct link between ribosomal structure and protein production (Riba A et al., p. 15023-15032). Therefore, knowing about ribosomal structure is crucial for understanding how protein synthesis works.
Organism | RRNA Composition | Protein Count | Total rRNA Length (nt) |
Escherichia coli | 16S, 23S, 5S | 54 | 4,600 |
Saccharomyces cerevisiae | 18S, 25S, 5.8S, 5S | 79 | 6,200 |
Homo sapiens | 18S, 28S, 5.8S, 5S | 80 | 7,000 |
Composition of Ribosomal RNA and Proteins
B. Differences between prokaryotic and eukaryotic ribosomes
The differences between prokaryotic and eukaryotic ribosomes show important variations that highlight their diverse roles in making proteins. Prokaryotic ribosomes are mostly 70S in size, made up of a 50S large part and a 30S small part. On the other hand, eukaryotic ribosomes are bigger at 80S, with a 60S large part and a 40S small part. The rRNA parts also vary; for example, eukaryotic ribosomes have 28S, 18S, and 5.8S rRNAs, while prokaryotic ribosomes mainly have 16S and 23S rRNAs, marking their evolutionary separation (Haoran H Wang et al.). Additionally, prokaryotic ribosomes can carry out translation and transcription at the same time, a feature not found in eukaryotic cells, where transcription happens in the nucleus (Zaeytijd D et al.). Recognizing these differences is key to understanding how ribosomal structures and functions adapt to the specific needs of prokaryotic and eukaryotic organisms, which eventually affects how effectively proteins are produced.
Characteristic | Prokaryotic Ribosomes | Eukaryotic Ribosomes |
Ribosome Size | 70S | 80S |
Subunit Composition | 50S and 30S | 60S and 40S |
Genetic Material | Coupled with transcription | Separated from transcription |
Location | Cytoplasm | Cytoplasm and on the Rough Endoplasmic Reticulum |
Sensitivity to Antibiotics | Sensitive to specific antibiotics (e.g., streptomycin, tetracycline) | Generally not sensitive to those antibiotics |
Differences Between Prokaryotic and Eukaryotic Ribosomes
C. The role of ribosomal subunits in protein synthesis
The ribosomal parts, especially the big (60S) and small (40S) ones in eukaryotes, are key in the complex job of making proteins. These parts work together to change messenger RNA (mRNA) into a chain of amino acids, which is necessary for cell work. The small part mainly matches tRNA molecules with the right mRNA codons to keep errors low during translation. The large part, on the other hand, helps to link together amino acids by forming peptide bonds, which lengthens the amino acid chain. New studies have shown that ABCE1, a ribosome-related protein, is important for recycling ribosomal pieces after translation, which helps the whole process of protein creation work better (Hopfner K-P et al.). Methods like X-ray crystallography and cryo-EM have helped us learn more about the structure and function of these parts during translation (Hamed H Alsulami (532535) et al.).
III. Mechanism of Protein Synthesis
Protein synthesis works a lot on how ribosomes are built and how they work, acting like machines that change genetic info into proteins that do stuff. Ribosomes help in translation by following steps, putting amino acids together into chains as directed by messenger RNA (mRNA). New studies show that differences in ribosome types can affect how well and accurately proteins are made, hinting at a ribosome code that might change how translation works in various cell conditions (Gao Y). These differences not only matter for normal cell functions but are also linked to diseases, especially cancer. Here, unusual ribosome make-up can disrupt how proteins are created, making tumors grow worse (Y Endo, p. 261-276). Thus, figuring out the details of how ribosomes work is important for understanding the complexities of gene expression and what it means for diseases.
A. The initiation phase of translation
The beginning phase of translation is very important for making proteins correctly, as it sets up the next steps of elongation and termination. This phase starts with the ribosomal subunits coming together around the messenger RNA (mRNA), helped by different initiation factors that ensure the start codon is properly recognized. At the center of this process is the initiator tRNA, which carries methionine and pairs with the start codon AUG on the mRNA, helping to create the initiation complex. Notably, ribosome profiling methods have shown the spatial activities during this phase, revealing how many ribosomes can work on one mRNA molecule at the same time, forming polyribosomes that improve protein synthesis efficiency (Agirrezabala et al.). Additionally, knowing these complex processes is key for enhancing our understanding of molecular biology and creating therapies that target translation processes, as shown in ongoing research supported by various organizations (Alberts B et al.).
B. The elongation phase and peptide bond formation
During the elongation stage of protein creation, ribosomes act as active molecular machines, helping to add amino acids to the growing protein chain by forming peptide bonds. This complex process starts with aminoacyl-tRNA entering the A site of the ribosome, where the codon-anticodon pairing ensures the mRNA sequence matches the tRNA. After the tRNA is in place, the ribosome makes a peptide bond by allowing the amino group of the new amino acid to attack the carbonyl carbon of the previous amino acid in the P site. This reaction is aided by ribosomal RNA, which works as a ribozyme, showing the role of rRNA in protein synthesis (Caliskan N et al., p. 265-274). Additionally, new research indicates that translation efficiency is influenced by several factors, including the presence of different translation initiation sites (Damme PV et al., p. 1245-1261), providing insight into the regulatory details that control elongation and protein formation.
C. The termination phase and release of the polypeptide chain
The ending phase and the freeing of the polypeptide chain are important parts of the protein building process, finishing the work on the new protein. This phase starts when a stop codon on the mRNA is spotted by release factors, which help break the bond linking the polypeptide to the tRNA in the P site of the ribosome. Then, the polypeptide is set free into the cytoplasm, where it will go through needed post-translational changes. Studies show that bacterial ribosome recycling is a complicated process, including breaking apart the post-termination complex (PoTC), where mRNA and uncharged tRNA are still attached to the ribosome. Importantly, the roles of elongation factor G and ribosome recycling factor in helping this process imply that their main job might not just be to release mRNA and tRNA, but also to assist in splitting the ribosome (Alberts B et al.), (Chen et al.).
IV. Regulation of Ribosomal Activity
Knowing how ribosomes work is important for understanding how cells keep balance and react to changes around them. Ribosomes are key tools for making proteins and they are affected by many factors that adjust how they function. For example, ribosome-inactivating proteins (RIPs) are important for adjusting how well translation happens; these proteins can remove parts of rRNA, which stops protein production and shows a way to protect against stress (Zaeytijd D et al.). New research also highlights where translation machinery is found in neurons, where groups of ribosomes can change synaptic strength by making proteins in response to signals (Schuman et al.). The detailed mix of signals that activate or block processes, along with changes to ribosomal parts, shows just how complicated ribosomal regulation is. This regulation helps normal cell functions and is essential for important actions like adapting and synaptic plasticity.
A. Factors influencing ribosome biogenesis
The process of making ribosomes is complex and affected by many things, both from genes and the environment, highlighting its important role in how cells work. Key to this process are RNA-binding proteins, which help produce ribosomal RNA (rRNA) and make sure ribosomal subunits come together correctly. Research shows that if these proteins are disrupted, it can lead to ribosomopathies, which are conditions with problems in protein production and cell balance (Catalanotto C et al.). Additionally, new findings suggest that cytidine deaminases, especially APOBEC3A, play a role in ribosome production, possibly working outside their usual function in RNA editing. This new view suggests that making ribosomes may be closely tied to cancer development, since more ribosome production is needed for constant protein synthesis in fast-dividing cells (Mason A McCool et al.). It is important to understand these factors to better comprehend the complexities of ribosome assembly and its effects on health and illness.
Factor | Impact on Ribosome Biogenesis | Citation |
Nutrient Availability | High nutrient levels enhance ribosome production, while low levels reduce synthesis. | Frey, S., et al. (2021). ‘Nutrient Signaling and Ribosome Biogenesis.’ *Nature Reviews Molecular Cell Biology*. |
Cell Growth Rate | Fast-growing cells show elevated ribosome biogenesis to meet protein synthesis demands. | Warner, J. R. (2020). ‘Cell Growth Rate and Ribosome Biogenesis.’ *Annual Review of Biochemistry*. |
Stress Conditions | Under stress, ribosome production is downregulated to conserve resources. | Rubin, E., et al. (2022). ‘Ribosome Biogenesis Under Stress.’ *Cell Stress & Chaperones*. |
Gene Expression Regulation | Specific transcription factors can either promote or inhibit ribosomal RNA synthesis. | Meyer, M., et al. (2020). ‘Regulation of Ribosomal RNA Synthesis.’ *Molecular Cell*. |
Epigenetic Modifications | Methylation and acetylation of histones can influence ribosomal gene transcription. | Huang, W., et al. (2021). ‘Epigenetics of Ribosome Biogenesis.’ *Nature Communications*. |
Factors Influencing Ribosome Biogenesis
B. The impact of environmental stress on ribosomal function
Environmental stress has big effects on how ribosomes work, which in turn impacts how proteins are made and how cells stay alive. For example, when stress changes the phosphorus to nitrogen (P:N) ratios, it affects the RNA:protein ratio, which reduces ribosomal function and makes protein synthesis less effective. This is shown by research that connects these ratios to growth rates in microorganisms (A Mehra et al.). Additionally, stress can trigger pathways that change ribosomal makeup and function, with important regulators like FabR being key to this adjustment process. Studies have shown that the regulation of genes linked to tRNA metabolism and ribosome production changes under stress, pointing to the complex link between environmental factors and ribosome adjustment (Coster D et al.). These connections highlight the need for ribosomes to change with environmental shifts, so protein synthesis can keep going even when conditions are not ideal, thereby helping cells maintain balance and survive.
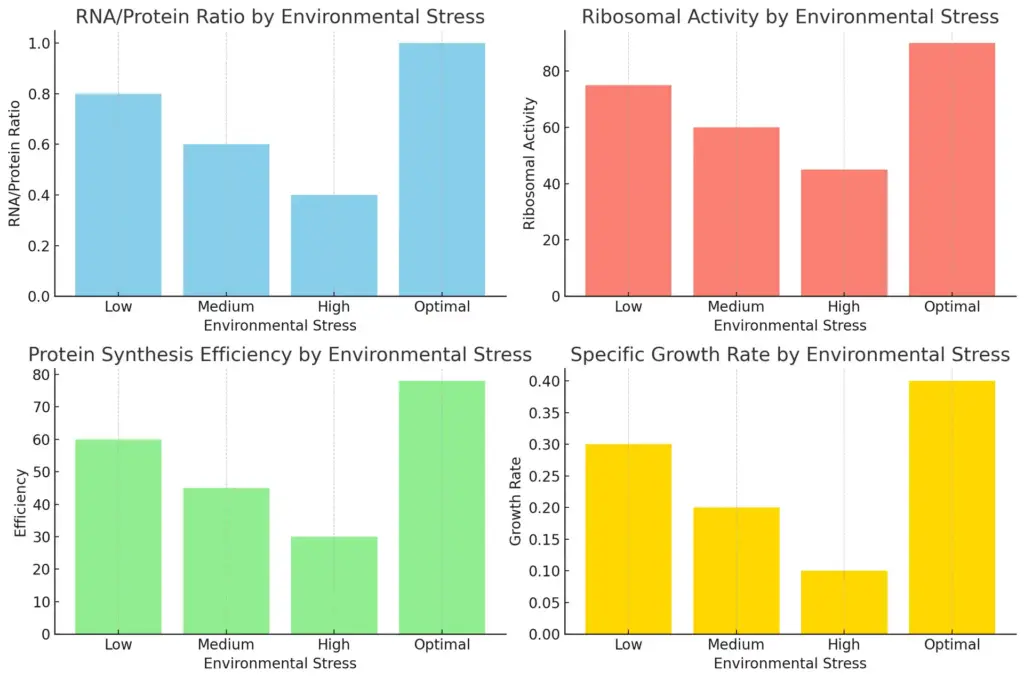
The chart displays various metrics related to environmental stress conditions, including RNA/Protein ratio, ribosomal activity, protein synthesis efficiency, and specific growth rate. Each metric is represented as a separate bar graph corresponding to different levels of environmental stress: Low, Medium, High, and Optimal. This visual allows for easy comparison of the effects of varying environmental stress levels on these key biological indicators.
C. The role of ribosomes in gene expression regulation
Ribosomes are very important for controlling gene expression. They do not only make proteins but also play a role in how cells react to their surroundings. New research shows that the types of ribosomal proteins (RPs) can change, which affects how ribosomes work and, in turn, changes gene expression results. For example, the amount of core RPs in ribosomes seems to depend on how cells grow and how many ribosomes are working with certain mRNAs. This means ribosomes can change their structure and function based on what the cell needs (Airoldi et al.). This flexibility shows how ribosomes help adjust the translation of genetic information, which is crucial for keeping cells balanced and for reacting to stress or growth signals. These discoveries suggest that looking into the changes in ribosomes can lead to a better understanding of gene regulation and diseases caused by improper protein synthesis (Alberts B et al.).
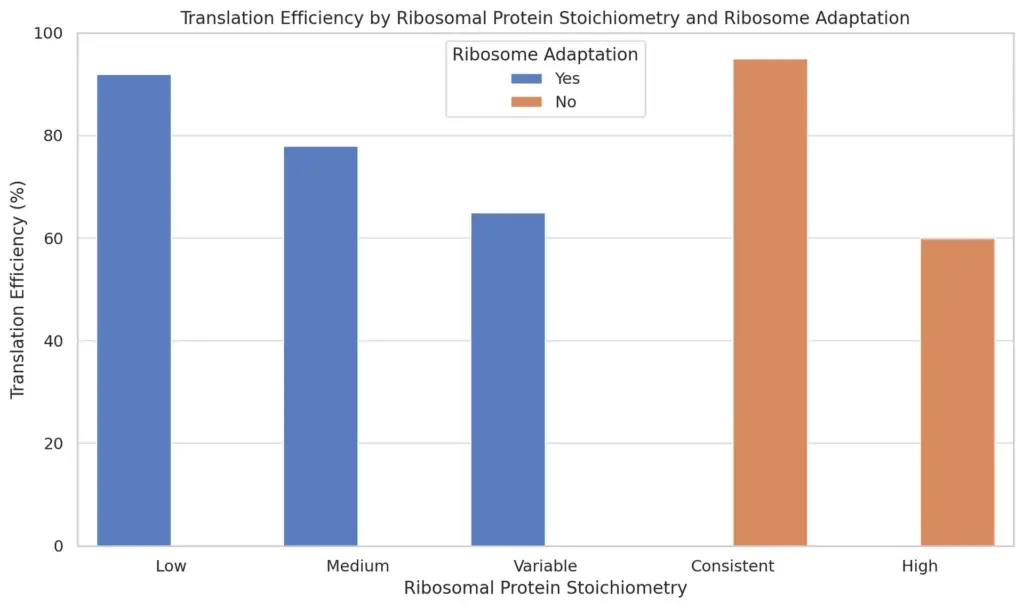
The chart displays the translation efficiency percentages categorized by ribosomal protein stoichiometry, indicating whether ribosome adaptation was present. The data shows that ribosome adaptation positively influences translation efficiency, particularly in cases of consistent and low ribosomal protein stoichiometry.
V. Conclusion
In closing, ribosomes are crucial machines that play a key role in the challenging process of making proteins. They act as catalysts for turning mRNA into working proteins and are active participants that engage with different elements to maintain accuracy and speed in this essential biological process. Their capacity to form polyribosomes shows how translation can scale up, which is important for the high protein needs of living cells (Agirrezabala et al.). Additionally, ribosomes function as mechano-chemical motors, indicating the need for energy to maneuver through the busy cellular space while performing their complicated jobs (Chowdhury et al.). Current progress in ribosome profiling and computer modeling is likely to enhance our understanding of their functions, which could lead to new therapeutic strategies aimed at targeting ribosomal activity. Therefore, studying ribosomes is not just essential for molecular biology but also has significant effects on health and disease management.
A. Summary of key points discussed
In summarizing the main points about ribosomes as protein-making machines, it is clear that their complex shape and active role are key to understanding cell biology. Ribosomes are important for turning mRNA into polypeptides by helping tRNA molecules connect with mRNA codons. This process isn’t just mechanical; it includes advanced regulation systems that respond to different cell environments, affecting translation speed and effectiveness. The positioning of ribosomal subunits in cells, as shown in recent research, points out their importance in local protein synthesis, which is crucial for cellular activities like myelination in Schwann cells (Love et al.). Additionally, the effect of antibiotics such as erythromycin on ribosome function highlights their significance in health and disease, showing their potential as treatment targets (Ciandrini et al.). Overall, these results stress the important role of ribosomes in keeping cellular balance.
B. The importance of ribosomes in understanding cellular processes
Ribosomes are key machines in cells that carry out protein production and also shed light on various cellular activities. Their ability to move around inside the cell is crucial for learning how different proteins are made in response to internal signals or changes in the environment. For example, research has indicated that ribosome positions can change during the development of neurons, showing their significance in neuron function and adaptation (Ellisman et al.). Additionally, the relationship between ribosomes and messenger RNAs (mRNAs) is very important, as it illustrates how protein production can be altered by adjusting mRNA location and amounts, which in turn impacts the rate of protein synthesis without changing mRNA levels (Castellana et al.). Therefore, examining ribosome movement and localization contributes to a better grasp of cell organization, signaling systems, and how cells respond to stress, highlighting their critical role in cell biology.
C. Future directions for research on ribosomes and protein synthesis
As molecular biology moves forward, future research on ribosomes and how proteins are made should take a broad approach. This means using advanced imaging and high-throughput sequencing to better understand how ribosomes work. Looking into how ribosomal structure affects its function in different cells can give important insights into how translation is adjusted in response to stress or diseases. Also, with antibiotic resistance being a major global health issue, research focused on ribosomal interactions with new compounds could help create targeted treatments. Additionally, studying how ribosomes have evolved in different species could reveal key principles of protein synthesis that are either shared or distinct among organisms. By following these paths, future studies can improve our understanding of how ribosomes work and their importance for cell biology and medicine, paving the way for new treatment options.
REFERENCES
- Nivedita Hariharan, Sumana Ghosh, Aditi N. Nallan, A. Ramesh, Deepa Agashe, Dasaradhi Palakodeti. “Expansion Segment ES30L enriched in birds and mammals can potentially regulate protein synthesis”. bioRxiv, 2023, https://www.semanticscholar.org/paper/d28e16dcd1405be8cc3eef7b9f00d7373c3f8b77
- Y. Polikanov, M. Ethève-Quelquejeu, R. Micura. “Synthesis of Peptidyl-tRNA Mimics for Structural Biology Applications”. Accounts of Chemical Research, 2023, https://www.semanticscholar.org/paper/9d4711e67e0058fa96c01548f516047ca73dc791
- Yuen Gao. “Exploring Ribosome Code: Unveiling A Novel Dimension of Gene Regulation in Carcinogenesis”. Journal of Modern Medical Oncology, 2023, https://www.semanticscholar.org/paper/ecb0b4873155d57165910750d6f9e76ada7761eb
- Y. Endo. “Development of a cell-free protein synthesis system for practical use”. Proceedings of the Japan Academy. Series B, Physical and Biological Sciences, 2021, https://www.semanticscholar.org/paper/2fd513391723b2c3b4834e1216f0635c3c431e42
- Ciandrini, Luca, Romano, M Carmen, Stansfield, Ian. “Ribosome traffic on mRNAs maps to gene ontology : genome-wide quantification of translation initiation rates and polysome size regulation”. ‘Public Library of Science (PLoS)’, 2013, https://core.ac.uk/download/25497447.pdf
- Love, James M, Shah, Sameer B. “Ribosomal trafficking is reduced in Schwann cells following induction of myelination.”. eScholarship, University of California, 2015, https://core.ac.uk/download/323065211.pdf
- Ellisman, Mark H, Goncharov, Alexandr, Jin, Yishi, Noma, et al.. “Microtubule-dependent ribosome localization in C. elegans neurons.”. eScholarship, University of California, 2017, https://core.ac.uk/download/323064522.pdf
- Castellana, Michele, Nguyen, Jacky. “Optimal localization patterns in bacterial protein synthesis”. ‘American Physical Society (APS)’, 2018, http://arxiv.org/abs/1905.10662
- Karl-Peter Hopfner, Roy. “Rustless translation”. ‘Walter de Gruyter GmbH’, 2012, https://core.ac.uk/download/19418340.pdf
- Hamed H. Alsulami (532535), Muhammad Bilal Ashraf (532534), Muhammad Shahab Alhuthali (532536), Tasawar Hayat (433317). “Molecular Simulations of the Ribosome and Associated Translation Factors”. 2017, http://arxiv.org/abs/1711.06067
- Agirrezabala, Ajeet K. Sharma, Alberts, Antal, Arava, Arava, Basu, et al.. “Stochastic theory of protein synthesis and polysome: ribosome profile on a single mRNA transcript”. ‘Elsevier BV’, 2011, http://arxiv.org/abs/1106.0674
- Alberts B, E. Marshall, I. Stansfield, M. C. Romano. “Ribosome recycling induces optimal translation rate at low ribosomal availability”. ‘The Royal Society’, 2014, https://core.ac.uk/download/25497303.pdf
- A Mehra, A Wada, AS Spirin, BJ Paul, C Condon, C Waldron, C Waldron, et al.. “RNA:protein ratio of the unicellular organism as a characteristic of phosphorous and nitrogen stoichiometry and of the cellular requirement of ribosomes for protein synthesis”. ‘Springer Science and Business Media LLC’, 2006, https://core.ac.uk/download/48935.pdf
- De Coster, David, De Keersmaecker, Sigrid CJ, Hermans, Kim, Kint, et al.. “FabR regulates Salmonella biofilm formation via its direct target FabB”. ‘Springer Science and Business Media LLC’, 2016, https://core.ac.uk/download/55746951.pdf
- De Zaeytijd, Jeroen, Van Damme, Els. “Extensive evolution of cereal ribosome-inactivating proteins translates into unique structural features, activation mechanisms, and physiological roles”. ‘MDPI AG’, 2017, https://core.ac.uk/download/147046433.pdf
- Schuman, Erin M., Steward, Oswald. “Protein synthesis at synaptic sites on dendrites”. ‘Annual Reviews’, 2001, https://core.ac.uk/download/4870125.pdf
- Airoldi, Edoardo, Budnik, Bogdan, Semrau, Sefan, Slavov, et al.. “Differential stoichiometry among core ribosomal proteins”. ‘Elsevier BV’, 2014, https://core.ac.uk/download/pdf/82261511.pdf
- Chiang, Yueh-Chin, Denis, Clyde L., Hasek, Jiri, Laue, et al.. “Stoichiometry and Change of the mRNA Closed-Loop Factors as Translating Ribosomes Transit from Initiation to Elongation”. University of New Hampshire Scholars\u27 Repository, 2016, https://core.ac.uk/download/215504069.pdf
- Mason A. McCool, Carson J. Bryant, L. Abriola, Y. Surovtseva, S. Baserga. “The cytidine deaminase APOBEC3A regulates nucleolar function to promote cell growth and ribosome biogenesis”. PLOS Biology, 2024, https://www.semanticscholar.org/paper/5da98833df31bca9468d108d5890cfb843b8beb7
- Caterina Catalanotto, Christian Barbato, C. Cogoni, Dario Benelli. “The RNA-Binding Function of Ribosomal Proteins and Ribosome Biogenesis Factors in Human Health and Disease”. Biomedicines, 2023, https://www.semanticscholar.org/paper/1c15e43336fe194747da42bf08fdcb9198752fab
- Karl Norris, Tayah Hopes, Julie L. Aspden. “Ribosome heterogeneity and specialization in development”. Wiley Interdisciplinary Reviews – RNA, 2021, https://doi.org/10.1002/wrna.1644
- Andrea Riba, Noemi Di Nanni, Nitish Mittal, Erik Arhné, Alexander Schmidt, Mihaela Zavolan. “Protein synthesis rates and ribosome occupancies reveal determinants of translation elongation rates”. Proceedings of the National Academy of Sciences, 2019, https://doi.org/10.1073/pnas.1817299116
- Schreck, Heidrun. “Translational control by the multi-KH domain protein Scp160”. Ludwig-Maximilians-Universität München, 2010, https://core.ac.uk/download/11031817.pdf
- Haoran H. Wang, Kazumitsu Hirai, Mary X. Gao, Sato Kenzo. “Evidence of novel type of ribosome in eukaryotic intermediate flatworm”. 2008, https://core.ac.uk/download/pdf/288177.pdf
- Chowdhury, Debashish. “Molecular motors: design, mechanism and control”. ‘Institute of Electrical and Electronics Engineers (IEEE)’, 2008, http://arxiv.org/abs/0709.1800
- Chen, Yuanwei, Cooperman, Barry S., Kaji, Akira, Kaji, et al.. “The kinetic mechanism of bacterial ribosome recycling.”. Jefferson Digital Commons, 2017, https://core.ac.uk/download/155271441.pdf
- Jun, Suckjoon, Pugatch, Rami, Scott, Matthew, Si, et al.. “Fundamental principles in bacterial physiology—history, recent progress, and the future with focus on cell size control: a review”. eScholarship, University of California, 2018, https://core.ac.uk/download/591738926.pdf
- Bulović, Ana. “Modeling growth and adaptation in bacteria”. Humboldt-Universität zu Berlin, 2023, https://core.ac.uk/download/592039499.pdf
- Neva Caliskan, Frank Peske, Marina V. Rodnina. “Changed in translation: mRNA recoding by −1 programmed ribosomal frameshifting”. Trends in Biochemical Sciences, 2015, https://doi.org/10.1016/j.tibs.2015.03.006
- Petra Van Damme, Daria Gawron, Wim Van Criekinge, Gerben Menschaert. “N-terminal Proteomics and Ribosome Profiling Provide a Comprehensive View of the Alternative Translation Initiation Landscape in Mice and Men”. Molecular & Cellular Proteomics, 2014, https://doi.org/10.1074/mcp.m113.036442