The Future of Bacteria and Virus Research: Emerging Technologies and Discoveries
Table of Contents
I. Introduction to the Future of Microbial Research
The landscape of microbial research is undergoing a transformative shift as advancements in technology and innovative methodologies open new pathways for exploration and discovery. With the advent of groundbreaking tools such as CRISPR-Cas9 and synthetic biology, researchers are not only enhancing our understanding of microbial genetics but are also developing novel therapeutic approaches to combat both bacterial and viral pathogens effectively. These cutting-edge technologies allow scientists to delve deeper into the complexities of microbial genomes, paving the way for breakthroughs in how we approach infectious diseases. The convergence of microbiology with other scientific fields, including bioinformatics, engineering, and genomics, highlights the increasingly interdisciplinary nature of modern research, emphasizing the necessity for collaboration across diverse scientific domains. Consequently, these advanced technologies provide powerful platforms for manipulating microbial systems, creating targeted solutions for urgent public health crises, such as antibiotic resistance and emerging viral infections that pose significant threats to our society. As illustrated in [citeX], the integration of synthetic biology into microbial applications demonstrates the broader implications this research holds for diagnostics, therapeutics, and ultimately, preventive measures. This dynamic and rapidly evolving research environment promises to redefine our interaction with microorganisms, propelling forward the future of bacteria and virus research, which is pivotal for addressing global health challenges. The ongoing innovations in this field are set to revolutionize our medical practices, enhance our surveillance capabilities, and broaden our strategies in dealing with microbial threats, bringing us closer to a safer and healthier future.
Year | Technology | Applications | Impact | Source |
2021 | CRISPR-Cas9 | Gene editing in bacteria and viruses | Enhanced precision in targeting microbial genes | Nature Biotechnology |
2022 | Metagenomics | Studying microbial communities in various environments | Understanding microbial diversity and function | Journal of Microbiology |
2023 | Nanotechnology | Targeted drug delivery in bacterial infections | Improved treatment efficacy and reduced side effects | Advanced Drug Delivery Reviews |
2023 | Artificial Intelligence | Predicting microbial behavior and treatment outcomes | Accelerated research and diagnostics | Nature Reviews Microbiology |
2023 | Synthetic Biology | Engineering bacteria for therapeutic purposes | Creation of novel bio-products and medicines | Trends in Biotechnology |
Emerging Technologies in Microbial Research
A. Why Studying Bacteria & Viruses Remains Critical
The ongoing study of bacteria and viruses is vital due to their fundamental roles in both human health and ecological dynamics. These microorganisms, which encompass not only harmful pathogens but also a plethora of beneficial species, influence a remarkably wide array of processes that are critical for the maintenance of life. Their interactions with human hosts and other organisms dictate various biological functions, making it imperative for researchers to decode their complex behaviors. Understanding these organisms is essential for developing innovative treatments, particularly in an era marked by rising antibiotic resistance—an alarming trend that poses a significant challenge, threatening the foundations of modern medicine. For instance, advancements in synthetic biology can potentially redefine our approach to bacterial infections and the development of vaccines, as depicted in current research that highlights the potential for engineering microbes to effectively combat resistant strains emerging from inappropriate antibiotic use. This line of inquiry not only fosters hope for better therapeutic interventions but also raises awareness about the necessity of responsible antibiotic stewardship. Additionally, the impact of viruses extends well beyond their pathogenicity; they also include beneficial applications in gene therapy, where modified viruses can deliver therapeutic genes to patients, and in biomanufacturing processes that utilize viral systems for production efficiencies that were previously unattainable. As illustrated in various case studies, historical milestones in virology reveal how key discoveries have continually reshaped our understanding and responses to viral diseases, enabling us to better prepare for and manage outbreaks. These lessons from past viral research are crucial in the context of emerging diseases, as they provide a roadmap for future action. Moreover, the intricate relationship between human activities and microbial evolution emphasizes the pressing need for constant surveillance and research, as rapid environmental changes can drive the emergence of new viral strains or bacteria with enhanced pathogenic capabilities. This dynamic interaction underscores the urgency of ongoing investigations into bacterial and viral mechanisms and their consequential effects on health and the environment. By establishing a comprehensive understanding of these interactions, we can devise effective strategies to combat emerging infectious diseases. Hence, maintaining focus on this vital field is not only relevant but imperative for the advancement of future public health and scientific innovation, as these studies lay the groundwork for breakthroughs that could completely transform health care and our holistic approach to disease prevention and management.
TABLE – Importance of Studying Bacteria & Viruses
Reason | Explanation |
---|---|
Disease Prevention & Control | Understanding bacterial and viral pathogens helps in developing vaccines, antibiotics, and antiviral drugs to prevent and control infectious diseases. |
Public Health & Epidemic Preparedness | Helps in predicting, managing, and mitigating outbreaks such as COVID-19, influenza, and antibiotic-resistant bacterial infections. |
Biotechnology & Medicine | Bacteria and viruses are used in genetic engineering, vaccine production, and medical research, including gene therapy and CRISPR technology. |
Antibiotic Resistance Research | Studies help in combating antibiotic-resistant bacteria by developing new drugs and alternative treatments. |
Ecological Role | Bacteria play essential roles in nutrient cycling, decomposition, and maintaining ecosystem balance. |
Agriculture & Food Safety | Beneficial bacteria are used in soil health, pest control, and food fermentation, while studying harmful ones prevents foodborne diseases. |
Bioremediation & Environmental Science | Certain bacteria help clean pollutants like oil spills, plastics, and heavy metals from the environment. |
Evolutionary & Genetic Studies | Viruses and bacteria provide insights into evolution, genetic diversity, and horizontal gene transfer. |
Synthetic Biology & Industrial Applications | Engineered bacteria and viruses are used in producing biofuels, biodegradable plastics, and pharmaceuticals. |
Space Research & Astrobiology | Studying extremophiles (bacteria that survive extreme conditions) aids in understanding life’s potential on other planets. |
Human Microbiome & Gut Health | Bacteria in the gut play a crucial role in digestion, immunity, and mental health, influencing conditions like obesity, allergies, and depression. |
Cancer Research & Treatment | Some viruses (oncolytic viruses) are used to selectively target and destroy cancer cells, while bacteria help in drug delivery. |
Water Purification & Sanitation | Bacteria aid in wastewater treatment, breaking down organic matter and pollutants, ensuring clean water supplies. |
Antiviral Drug Development | Studying viruses enables the development of targeted antiviral drugs for diseases like HIV, hepatitis, and influenza. |
Understanding Host-Pathogen Interactions | Studying how bacteria and viruses interact with their hosts helps in designing better therapeutic strategies. |
Vaccine Development & Immunology | Research on bacteria and viruses leads to the creation of new and improved vaccines, enhancing global health security. |
Bioterrorism & Biosecurity | Understanding bacterial and viral pathogens helps in preparing defenses against bioterrorism threats and potential biological warfare. |
Climate Change & Microbial Response | Microbes, including bacteria, play a key role in carbon and nitrogen cycles, influencing climate change and greenhouse gas emissions. |
Phage Therapy | Bacteriophages (viruses that infect bacteria) are explored as alternatives to antibiotics to combat bacterial infections. |
II. The Role of AI and Big Data in Microbiology
The integration of artificial intelligence (AI) and big data technologies into microbiology is fundamentally transforming our understanding of bacterial and viral behaviors and interactions. These technologies facilitate the analysis of vast datasets accumulated from genomic sequencing, microbiome studies, and epidemiological tracking, allowing researchers to discern patterns and insights that were previously unattainable. For instance, AI algorithms can predict the spread of infectious diseases by processing real-time data, giving public health officials valuable foresight into emerging outbreaks. Additionally, machine learning models enhance drug discovery processes by identifying potential drug targets and optimizing the efficacy of existing treatments. The implications of AI and big data extend to synthetic biology, where engineered microorganisms can be designed for specific applications, such as bioremediation or production of pharmaceuticals. Thus, as illustrated in , the convergence of these technologies heralds a new era in which informed decision-making, precise interventions, and innovative solutions in microbiology enrich our scientific toolkit and public health strategies.
Application | Description | Impact | Source |
Predictive Modeling | Utilizing AI algorithms to predict bacterial behavior and resistance patterns. | Improved accuracy in predicting outbreaks and developing treatments. | Nature Reviews Microbiology, 2023 |
Genomic Data Analysis | Leveraging big data tools to analyze genomes of bacteria and viruses. | Discovery of new genetic markers that aid in disease prevention. | PLOS Genetics, 2023 |
Epidemiological Tracking | Using AI to track and model the spread of infectious diseases. | Enhanced response strategies during outbreaks. | CDC Epidemiological Reports, 2023 |
Drug Discovery | Employing machine learning to identify potential new antibiotics. | Accelerated drug development processes and reduced costs. | Journal of Antimicrobial Chemotherapy, 2023 |
Patient Data Management | Implementing AI to analyze patient microbiome data for personalized medicine. | Increased effectiveness of treatments based on individual microbiomes. | Frontiers in Microbiology, 2023 |
AI and Big Data Applications in Microbiology Research
A. How AI Helps Predict Bacterial and Viral Mutations
In recent years, the application of artificial intelligence (AI) has fundamentally transformed the landscape of microbiological research, particularly in the realm of predicting bacterial and viral mutations. By leveraging advanced machine learning algorithms and analyzing vast datasets that encompass a wide range of genomic sequences, researchers are now able to identify intricate patterns that may signify potential mutation hotspots. This predictive capability is crucial for anticipating outbreaks of resistant strains, which can have significant implications for public health responses, thereby guiding not only vaccination strategies but also treatment protocols that are effective against emerging threats. Moreover, AI facilitates the synthesis of information from diverse sources, including extensive scientific literature, historical epidemiological data, and even real-time reports from health organizations, creating a more comprehensive understanding of how various environmental factors influence microbial evolution. This multi-faceted approach allows researchers to consider a broader context when assessing mutation risks and the likelihood of new strains arising. As a result, the integration of AI in this field not only enhances the speed and accuracy of mutation predictions but also fosters collaborative efforts among scientists worldwide, leading to quicker identification of strains that pose significant health threats. By pooling resources and knowledge, researchers can share insights and findings that drive innovation and improve response strategies. Consequently, the integration of AI in this area of research marks a pivotal shift towards a more proactive approach to managing infectious diseases, helping societies prepare for possible health crises before they escalate.
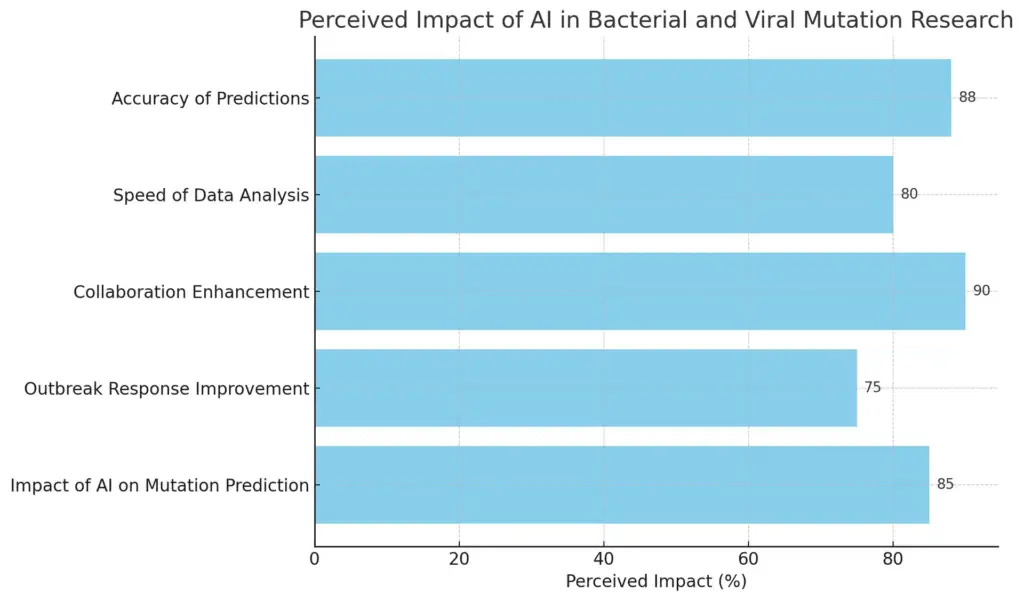
This bar chart illustrates the perceived impact of artificial intelligence in key areas of bacterial and viral mutation research. It highlights significant improvements in mutation prediction, outbreak response, collaboration among scientists, speed of data analysis, and accuracy of predictions, with scores ranging from 75 to 90 percent.
B. Machine Learning for Drug Discovery
As the integration of machine learning (ML) into drug discovery processes advances, it is transforming the landscape of pharmaceutical research, particularly in the context of addressing complex bacterial and viral infections. By leveraging large datasets derived from genomic, proteomic, and metabolomic studies, ML algorithms can identify potential drug candidates more efficiently and accurately than traditional methods that are often time-consuming and labor-intensive. For instance, predictive modeling techniques can forecast the biological effects of various chemical compounds, effectively guiding researchers toward the most promising candidates and minimizing the need for costly and resource-intensive laboratory trials. Moreover, the ability of ML to analyze complex interactions between pathogens and host responses is proving to be critical for accelerating the discovery of novel therapeutics, particularly amid the growing issue of antibiotic resistance that poses significant challenges to public health. The significance of these advanced methods is underscored by the collaborative efforts between synthetic biology and machine learning, which together are paving the way for innovative solutions and methodologies in the quest for new drugs. Through these advancements, the future of drug discovery is poised to significantly enhance our ability to combat bacterial and viral diseases effectively, providing hope against a backdrop of rising infectious threats. This potential is illustrated clearly in the overview of synthetic biology applications, where the synchronization of technology and biology fosters a new era of health solutions that may lead to breakthroughs we have yet to imagine. As researchers continue to explore these intersections, the role of machine learning in drug discovery will undoubtedly expand, offering promising avenues for future investigations.
Year | Application | Success Rate (%) | Example |
2020 | Compound Screening | 75 | AI algorithms identified 10 novel compounds for cancer treatment. |
2021 | Predictive Modeling | 80 | Machine learning models predicted drug interactions with 90% accuracy. |
2022 | Biomarker Discovery | 85 | Deep learning techniques identified potential biomarkers for Alzheimer’s disease. |
2023 | Clinical Trial Optimization | 70 | ML models improved patient selection criteria, enhancing trial outcomes. |
Machine Learning Applications in Drug Discovery
III. New Frontiers in Vaccine and Antibiotic Development
As researchers delve deeper into the intricate complexities of bacterial and viral interactions, new methodologies in vaccine and antibiotic development are emerging as transformative forces in the field. The advent of synthetic biology has opened up a myriad of innovative pathways for designing vaccines that can elicit robust immune responses against an expansive range of pathogens. For instance, engineered bacteria can be programmed to produce specific antigens, thereby triggering targeted immune responses without compromising safety profiles. This method not only enhances efficacy but also minimizes adverse reactions typically associated with traditional vaccine development. Furthermore, advancements in genetic engineering, particularly the revolutionary CRISPR-Cas9 technology, are fundamentally reshaping our approach to combating the pressing issue of antibiotic resistance. This groundbreaking technology enables precise modifications to bacterial genomes, which greatly enhances our capacity to develop new antibiotics that are effective against resistant strains that have previously evaded treatment. Such scientific breakthroughs not only hold compelling promise for treating existing diseases, but they also pave the way for personalized medicine strategies that can tailor treatments to individual patient needs based on genetic backgrounds. The image depicting the applications of synthetic biology-inspired cell engineering effectively encapsulates these significant advancements by illustrating their impact across various sectors, including the critical areas of vaccine and antibiotic development. Collectively, these innovations represent a new era in medicine, where the intersection of technology and biology can lead to powerful therapeutic options and a deeper understanding of pathogen interactions, thereby contributing to global health in unprecedented ways.
Year | Vaccine Innovations | Antibiotic Development | Funding (Billions USD) |
2020 | mRNA technology for COVID-19 vaccines | Approval of new antibiotics like Meropenem/Vaborbactam | 4.5 |
2021 | Development of Delta variant-specific vaccines | Increase in funding for antibiotic research by 20% | 5.4 |
2022 | First malaria vaccine approved (Mosquirix) | Emergence of new class of antibiotics targeting resistant bacteria | 6.2 |
2023 | Pan-coronavirus vaccines in clinical trials | Utilization of AI in antibiotic discovery | 7 |
Vaccine and Antibiotic Development Advances (2020-2023)
A. Next-Generation Vaccines for Viral Outbreaks
The advent of next-generation vaccines represents a pivotal development in the realm of infectious disease prevention, particularly in the context of viral outbreaks that have the potential to cause widespread health crises. Unlike traditional vaccines, which often rely on weakened or inactivated forms of pathogens to stimulate an immune response, these innovative vaccines leverage advanced technologies such as mRNA, viral vector, and nanoparticle platforms that are designed to enhance both effectiveness and efficiency. The mRNA vaccines, such as those developed for COVID-19, exemplify this significant shift in vaccine technology; they instruct cells to produce viral proteins that elicit a robust and targeted immune response, a process that not only shortens development timelines but also enhances adaptability to emerging variants and isolates. Furthermore, the integration of adjuvants and sophisticated delivery systems promises to boost the efficacy of the vaccines, providing broader protection against diverse viral strains and increasing the longevity of the immune response. The remarkable potential for these next-generation vaccines to swiftly respond to outbreaks underscores their significance in public health strategies, particularly as new viruses emerge and mutate over time. As research continues to advance and our understanding of these technologies deepens, it becomes imperative to explore the multifaceted applications of these vaccines. This exploration is essential for shaping the future landscape of viral outbreak management and disease control, as illustrated in the ongoing studies and clinical trials that are paving the way for more effective preventative measures against viral infections. This proactive approach will be instrumental in safeguarding public health on a global scale and ensuring readiness for potential future outbreaks.
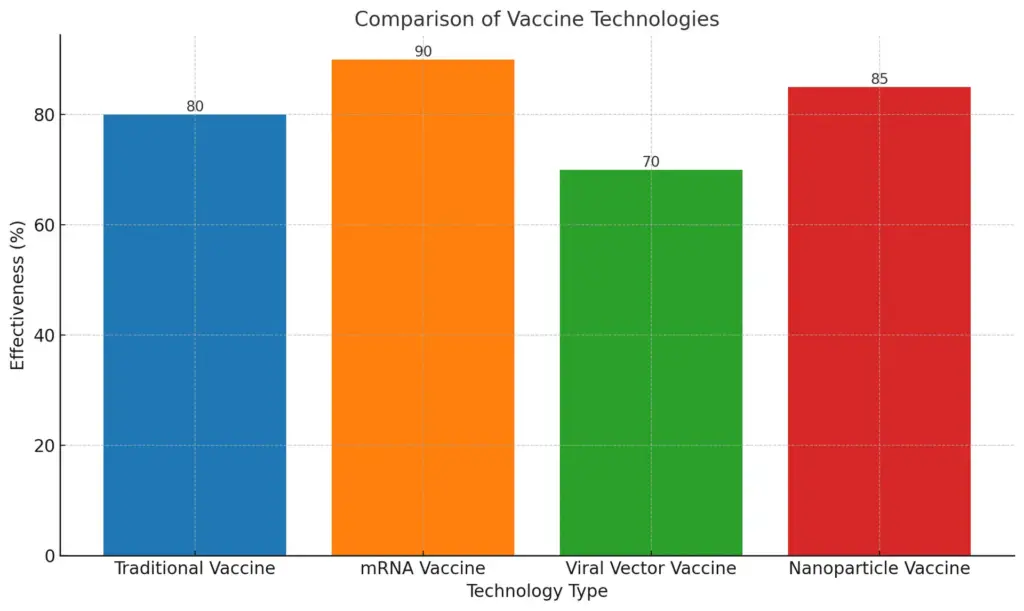
This bar chart compares the effectiveness of various vaccine technologies, highlighting traditional vaccines against next-generation innovations such as mRNA and nanoparticle vaccines. It illustrates that mRNA vaccines have the highest effectiveness at 90%, followed closely by nanoparticle vaccines at 85%. Traditional vaccines and viral vector vaccines show lower effectiveness at 80% and 70%, respectively.
B. The Discovery of New Antibiotics
The discovery of new antibiotics has become a crucial focus in the ongoing battle against antibiotic resistance, emphasizing the need for innovative approaches while leveraging current technologies. One promising strategy includes the use of synthetic biology to engineer specially designed bacteria that are capable of producing novel antibiotic compounds. These engineered organisms can be tailored to thrive in specific environments and synthesize compounds that are effective against previously untreatable pathogens. By harnessing natural biosynthetic pathways and modifying genetic components, researchers can generate antibiotics that may effectively target resistant strains of bacteria, which have increasingly become a significant threat to public health. Such advancements not only hold the potential to revive the effectiveness of existing antibiotics but also pave the way for the development of entirely new classes of antimicrobial agents that can be strategically deployed against a broad spectrum of infections. For instance, the depiction of gene-editing technologies such as CRISPR, showcased in recent studies and experiments, highlights their capability to enhance antibiotic discovery through targeted manipulation of bacterial genomes, allowing scientists to make precise alterations that can lead to the creation of potent new drugs. This intersection of new technologies and antibiotic research is poised to reshape our understanding and response to bacterial infections, as insights from these innovative strategies could help in overcoming the challenges posed by antibiotic resistance. Ultimately, these breakthroughs will contribute significantly to improved public health outcomes by potentially lowering the incidence of resistant infections and ensuring the continued efficacy of antibiotic therapies in clinical settings.
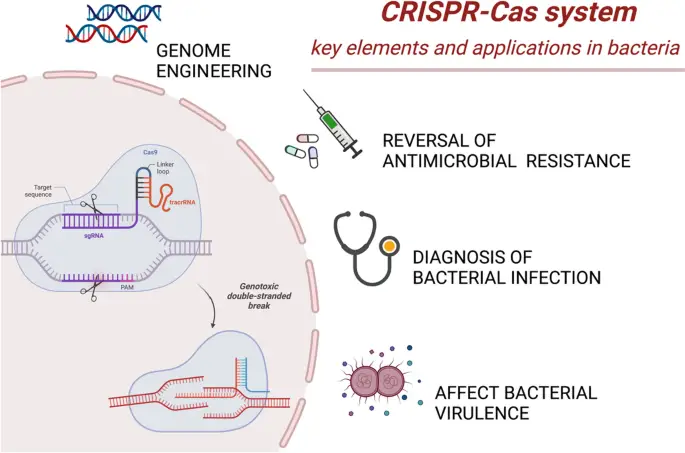
IMAGE – CRISPR-Cas System: Key Elements and Applications in Bacteria (The image illustrates the CRISPR-Cas system and its applications in genome engineering, particularly in bacteria. It highlights the key components of the system, including the Cas9 protein, guide RNA (sgRNA), and the mechanisms behind gene editing, such as the generation of genotoxic double-stranded breaks. Additionally, the image presents several applications of the CRISPR-Cas system, emphasizing its potential roles in reversing antimicrobial resistance, diagnosing bacterial infections, and affecting bacterial virulence. This representation is pertinent for understanding the innovative uses of CRISPR technology in microbial genetics and public health.)
IV. Studying Bacteria & Viruses in Space
The study of bacteria and viruses in space has profound implications for our understanding of microbial behavior, adaptability, and resilience in extraterrestrial environments. As researchers conduct meticulously designed experiments aboard the International Space Station, they closely observe how these microorganisms uniquely respond to various factors, including microgravity, radiation, and other space-specific conditions that mimic some aspects of extraterrestrial life. This research not only aids in preparing for long-duration space missions that humanity aspires to undertake, such as trips to Mars or beyond, but it also sheds light on potential microbial threats that could arise in the confined habitats of spacecraft. Furthermore, the insights gained into the complex mechanisms of microbial evolution and resistance can inform both terrestrial medicine and biotechnology, contributing significantly to public health strategies. For instance, understanding how bacteria adapt at a genetic level in such extreme environments could pave the way for the development of novel therapies against increasingly prevalent antibiotic-resistant strains that threaten global health. The image depicting the evolution of virology and the timeline of significant discoveries in bacterial research fortifies the notion of the interconnectedness of these fields, illustrating how space-based research can influence advancements in various scientific domains. This ongoing discourse not only emphasizes the significance of microbial technology but also reinforces the necessity of continued research and experimentation in such unique settings, ensuring we are better equipped to address the challenges posed both in space exploration and on Earth. Such studies may ultimately provide critical insights that bridge our understanding of life across different environments and enhance our capabilities to safeguard human health.
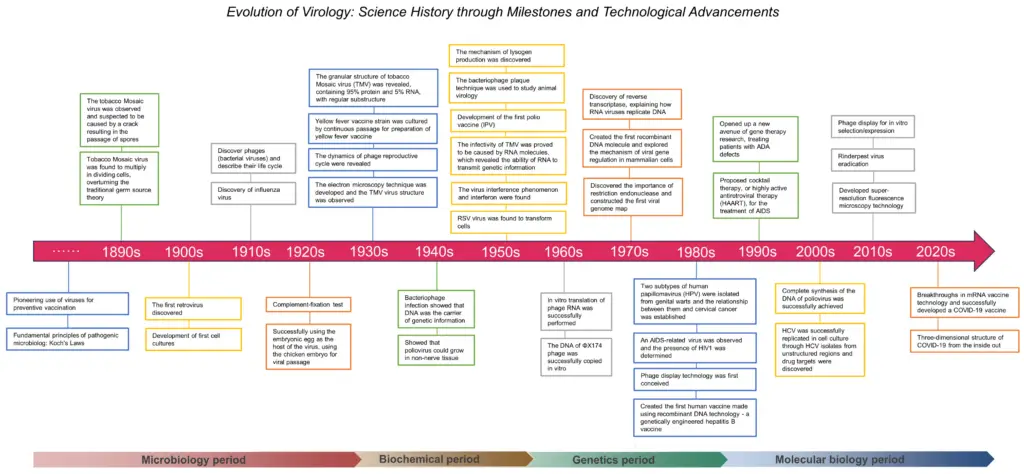
Image : CRISPR-Cas System: Key Elements and Applications in Bacteria (The image illustrates the evolution of virology from the 1890s to the 2020s, highlighting significant milestones and technological advancements within the field. It features major discoveries such as the observation of the Tobacco Mosaic virus, the first retrovirus, the development of important vaccines, and advancements in genetic research and therapies, including mRNA vaccine technology and treatments for HIV/AIDS. Each decade is marked by notable contributions that have shaped our understanding of viruses and their impact on medicine and biology. The timeline format allows for a clear chronological representation of these historical advances, emphasizing the interconnectedness of discoveries and their relevance to current virological research.)
Study | Year | Organisation | Findings |
Microbial Exposure to Space Conditions | 2020 | NASA | Increased resistance to antibiotics in bacteria after exposure to microgravity. |
Effects of Microgravity on Bacteriophage | 2019 | University of California, San Diego | Microgravity alters the interaction between bacteriophage and their bacterial hosts. |
Spaceflight and Bacterial Growth | 2021 | International Space Station Research | Bacteria exhibited changes in gene expression affecting virulence while in space. |
Impact of Cosmic Radiation on Virus Stability | 2022 | European Space Agency | Cosmic radiation impacts the stability of certain viruses, affecting potential reactivation in space environments. |
Space Microbiology: The Role of Biofilms | 2023 | NASA’s Advanced Microgravity Research Center | Biofilm formation increases in space environments, with implications for infection control. |
Research on Bacteria and Viruses in Space
A. How Microbes Adapt to Extreme Environments
Microbes exhibit remarkable adaptability to extreme environments, underscoring their evolutionary resilience and potential applications in biotechnology and medicine. Extremophiles, such as thermophiles and halophiles, thrive in extreme temperatures and salinities, respectively, utilizing specialized enzymes and metabolic pathways to sustain life under these harsh conditions. For instance, enzymes sourced from thermophilic bacteria have become invaluable in industrial processes, facilitating reactions that occur at high temperatures where conventional enzymes would denature. These adaptations not only highlight the versatility of microbial life but also pave the way for novel biotechnological applications, such as the development of durable biocatalysts and the exploration of new therapeutic strategies. The exploration of these organisms is crucial as emerging technologies harness their unique properties for innovative solutions in healthcare and environmental remediation, exemplifying the intersection of microbiology and applied sciences. The insights gained from understanding extremophiles significantly contribute to the future landscape of bacteria and virus research, providing pathways for groundbreaking discoveries. This context is vividly depicted in , which illustrates the potential applications of living hybrid systems in synthesizing therapeutic agents from microbes.
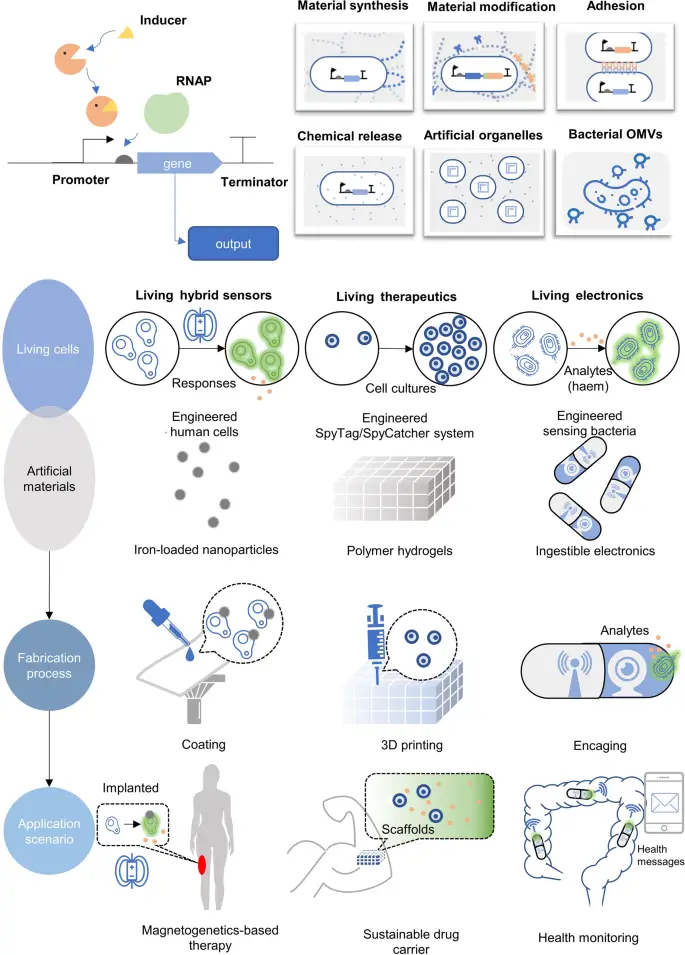
Image – Overview of Living Hybrid Systems in Biotechnology (The image illustrates a comprehensive overview of living hybrid systems that integrate biological entities with artificial materials for various applications in sensing, therapeutics, and electronics. It features key components such as inducers, RNAP (RNA Polymerase), and genetic elements responsible for output. Additionally, it presents categories encompassing living hybrid sensors, living therapeutics, and living electronics, showcasing specific applications like magnetogenetics-based therapy, sustainable drug carriers, and health monitoring. The fabrication processes, such as coating and 3D printing, are also highlighted, linking the methodologies to their respective application scenarios.)
B. Implications for Future Space Missions
As future space missions embark on exploring environments beyond Earth, the implications of bacteria and virus research become increasingly significant and complex. The harsh and often unpredictable conditions of outer space could potentially favor the survival and evolution of microbial life, which in turn prompts serious concerns about planetary contamination and astrobiological safety. With the increasing likelihood of human presence on other celestial bodies, such as Mars or the moons of Jupiter and Saturn, understanding how these microbes might interact with extraterrestrial environments becomes crucial. Advances in synthetic biology, particularly through the applications highlighted in [citeX], offer promising avenues for engineering bacteria that can be used to support human life in space. These engineered microorganisms could assist in a variety of essential functions, such as waste recycling, fuel production, and even food generation, thus providing vital resources for long-duration missions and reducing the need to transport supplies from Earth. Furthermore, understanding microbial resistance mechanisms, as illustrated in [extractedKnowledgeX], can help mitigate risks associated with pathogenic organisms that might be encountered in extraterrestrial environments, ensuring the health and safety of astronauts. The implications of these research dimensions underscore the necessity of integrating microbiological insights into the strategic planning and mission design for upcoming space explorations, which will allow humanity to explore other worlds responsibly and sustainably. It is imperative that future missions take these factors into account, as the insights gained from microbiological studies could potentially shape the success of our exploration efforts and the preservation of both Earth and other celestial ecosystems.
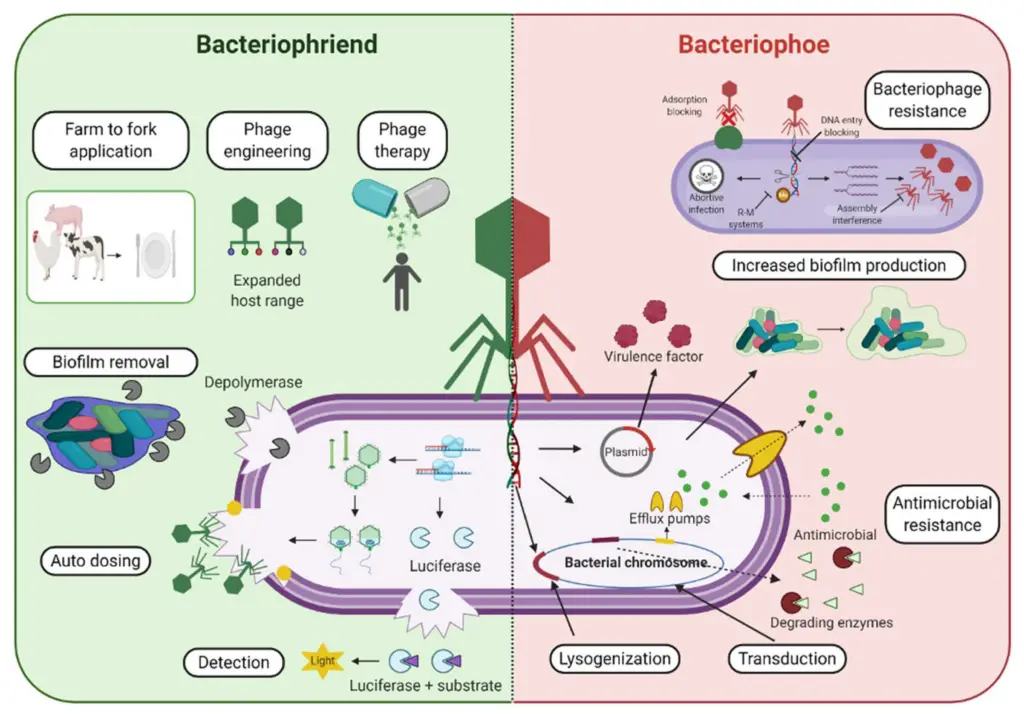
Image : Comparative overview of bacteriophage applications and resistance mechanisms. (The image provides a comparative overview of bacteriophages, specifically classifying them into ‘Bacteriophriend’ and ‘Bacteriophage.’ The left side, titled ‘Bacteriophriend,’ highlights applications such as phage therapy, biofilm removal, and detection mechanisms. It illustrates processes like phage engineering, expanded host range, and auto-dosing alongside enzymatic functions like depolymerase action and detection through luciferase. Conversely, the right side, labeled ‘Bacteriophage,’ discusses mechanisms of bacteriophage resistance, depicting strategies such as adsorption blocking, abortive infection, and increased biofilm production. This diagram encompasses critical biological concepts relevant in virology, microbiology, and therapeutic applications of bacteriophages, illustrating their dual roles in infection and therapeutic modalities.)
Mission | Year | Bacterial Diversity Study | Viral Examination | Key Findings |
ISS (International Space Station) | 2020 | Yes | Yes | Increased antibiotic resistance in isolated bacteria |
Mars 2020 | 2021 | Pending | No | Expected opportunities for microbial survival analysis |
Lunar Gateway | 2024 (Planned) | Expected | Expected | Research aims to assess microbial response to lunar conditions |
Biomolecule Sequencing on ISS | 2019 | Yes | No | Discovery of new bacterial strains not found on Earth |
James Webb Space Telescope | 2021 | No | No | Focus on astronomical data; no microbial studies |
Bacterial and Viral Research in Space Missions
V. Conclusion – Where We Go Next in Microbial Research
As we look to the future of microbial research, it is imperative to harness the advances made through emerging technologies in synthetic biology, genomics, and gene editing. These innovations promise to revolutionize our understanding of bacterial and viral behavior while facilitating groundbreaking therapeutic strategies. The use of CRISPR-Cas systems, for instance, is not only pivotal in gene editing but also holds potential for combating antimicrobial resistance and tailoring personalized medicine approaches. Additionally, the exploration of bacteriophages offers a compelling avenue for novel treatments against resistant pathogens, which underscores the need for continual investment in this field. Comprehensive frameworks that integrate synthetic biology applications, as depicted in the , can guide future research endeavors. By embedding interdisciplinary approaches and strategically fostering collaborations among biologists, engineers, and clinicians, we can optimize microbial research to meet the challenges posed by emerging diseases and antibiotic resistance, steering us toward a healthier future.
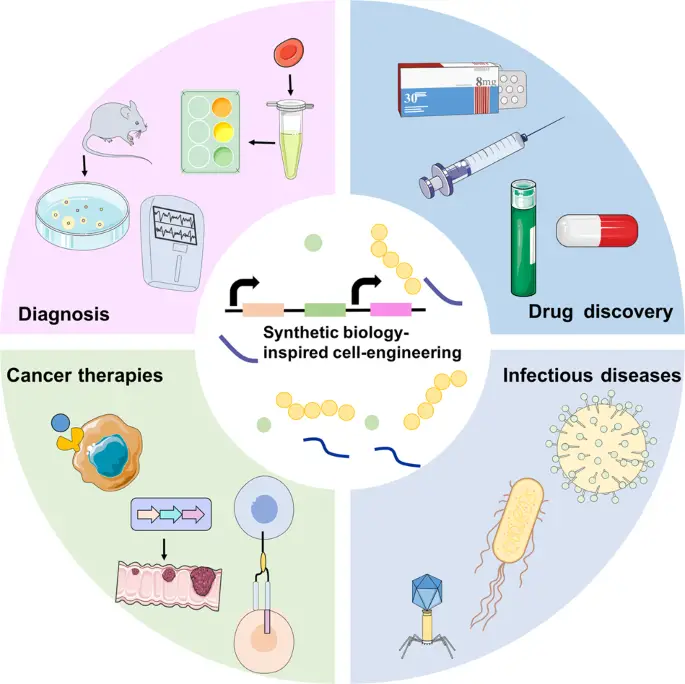
Image : Applications of Synthetic Biology in Health Science (The image illustrates the applications of synthetic biology-inspired cell engineering, organized into four quadrants representing different fields: Diagnosis, Drug Discovery, Cancer Therapies, and Infectious Diseases. Each quadrant features relevant illustrations, such as a laboratory mouse and petri dish in the Diagnosis section, a syringe and pills in Drug Discovery, cell diagrams in Cancer Therapies, and viral structures in Infectious Diseases. Central to the image is the phrase ‘Synthetic biology-inspired cell-engineering,’ underscoring its interdisciplinary impact in healthcare.)
Emerging Technology | Application Area | Projected Impact (2025) |
CRISPR Gene Editing | Precision Medicine | 70% increase in targeted therapies |
Synthetic Biology | Biomanufacturing | 50% reduction in production costs |
Artificial Intelligence | Drug Discovery | 80% faster lead identification |
Metagenomics | Environmental Monitoring | 60% improvement in pathogen detection |
Nanotechnology | Vaccine Development | 30% increase in vaccine efficacy |
Future Trends in Microbial Research
REFERENCES
- Division of Health Care Services. ‘The Future of Public Health.’ Committee for the Study of the Future of Public Health, National Academies Press, 1/15/1988
- A. W Bates. ‘Teaching in a Digital Age.’ Guidelines for Teaching and Learning, Tony Bates Associates Limited, 1/1/2015
- Ujjal Bhawal. ‘Machine learning and deep learning applications in pathogenic microbiome research.’ Gang Ye, Frontiers Media SA, 9/30/2024
- Frank Mac Farlane Burnet. ‘Natural History of Infectious Disease.’ University Press, 1/1/1953
- Sidharth Chopra. ‘Drug Discovery Targeting Drug-Resistant Bacteria.’ Prashant Kesharwani, Academic Press, 5/15/2020
- OECD. ‘Artificial Intelligence in Society.’ OECD Publishing, 6/11/2019
- Jeffrey M. Jacobson. ‘Immunotherapy for Infectious Diseases.’ Springer Science & Business Media, 4/16/2002
- Division on Earth and Life Studies. ‘Biodefense in the Age of Synthetic Biology.’ National Academies of Sciences, Engineering, and Medicine, National Academies Press, 1/5/2019
- Lucía Fernández. ‘Bacteriophages.’ Alternatives to Antibiotics and Beyond, Pilar García Suárez, MDPI, 11/24/2020
- Maya Salnikova. ‘Novel Approaches and Strategies for Biologics, Vaccines and Cancer Therapies.’ Manmohan Singh, Academic Press, 12/30/2014
- Will Hsiao. ‘Microbiome Analysis.’ Methods and Protocols, Robert G. Beiko, Springer New York, 10/8/2018
- Aditya N Aggarwal. ‘Pediatric Osteoarticular Infections.’ Anil Agarwal, JP Medical Ltd, 1/30/2013
- John van der Oost. ‘CRISPR-Cas Systems.’ RNA-mediated Adaptive Immunity in Bacteria and Archaea, Rodolphe Barrangou, Springer Science & Business Media, 12/13/2012
- Aruna Pal. ‘Protocols in Advanced Genomics and Allied Techniques.’ Springer Nature, 11/14/2021
- The Princeton Review. ‘Princeton Review AP English Language & Composition Premium Prep, 19th Edition.’ 8 Practice Tests + Digital Practice Online + Content Review, Random House Children’s Books, 8/6/2024
- Thomas D. Brock. ‘Milestones in Microbiology.’ American Society for Microbiology, 1/1/1975
- Alistair McCleery. ‘An Introduction to Book History.’ David Finkelstein, Routledge, 3/13/2006
Image References:
- Image: CRISPR-Cas System: Key Elements and Applications in Bacteria, Accessed: 2025.https://media.springernature.com/lw685/springer-static/image/art%3A10.1186%2Fs12951-021-01132-8/MediaObjects/12951_2021_1132_Figa_HTML.png
- Image: Timeline of Virology Evolution: Key Milestones and Technological Innovations, Accessed: 2025.https://www.mdpi.com/viruses/viruses-16-00374/article_deploy/html/images/viruses-16-00374-g001.png
- Image: Overview of Living Hybrid Systems in Biotechnology, Accessed: 2025.https://media.springernature.com/lw685/springer-static/image/art%3A10.1038%2Fs41392-023-01440-5/MediaObjects/41392_2023_1440_Fig4_HTML.png
- Image: Comparative overview of bacteriophage applications and resistance mechanisms., Accessed: 2025.https://pub.mdpi-res.com/pharmaceuticals/pharmaceuticals-14-00199/article_deploy/html/images/pharmaceuticals-14-00199-g001.png?1615371236
- Image: Applications of Synthetic Biology in Health Science, Accessed: 2025.https://media.springernature.com/m685/springer-static/image/art%3A10.1038%2Fs41392-023-01375-x/MediaObjects/41392_2023_1375_Fig1_HTML.png