The Role of Mitochondria in Exercise and Metabolism
I. Introduction
A comprehensive understanding of mitochondria’s pivotal role in exercise and metabolism is essential for elucidating how energy production influences physical performance and overall health. Mitochondria, often dubbed the powerhouse of the cell, are integral to ATP production through oxidative phosphorylation, a process that is particularly enhanced during physical activity. The functional capacity of mitochondria is closely linked to metabolic pathways, enabling the body to efficiently utilize substrates like glucose and fatty acids for energy. As exercise demands increase, so does the reliance on mitochondrial biogenesis and dynamics—processes that facilitate the synthesis of new mitochondria and the maintenance of existing ones. This interplay not only underscores the importance of mitochondrial health in athletic performance but also highlights its implications in metabolic disorders, thereby establishing a crucial foundation for further exploration into mitochondrial function as a determinant of both fitness and metabolic resilience.
A. Definition of mitochondria and their significance in cellular function
Mitochondria, often referred to as the powerhouse of the cell, are double-membrane-bound organelles that play a critical role in energy production through the process of oxidative phosphorylation. They facilitate the conversion of energy stored in nutrients into adenosine triphosphate (ATP), the primary energy currency of the cell, thus underpinning essential cellular functions. Beyond ATP production, mitochondria are involved in regulating metabolic pathways, including the Krebs cycle and fatty acid oxidation, which are particularly vital during periods of increased energy demand, such as exercise. Moreover, the dynamic nature of mitochondria, characterized by processes of fission and fusion, allows for the maintenance of mitochondrial integrity and function, crucial in response to metabolic stressors . Consequently, proper mitochondrial function is not only essential for energy homeostasis but also serves as a determinant of metabolic health, influencing conditions such as obesity and insulin resistance (Alessio et al.).
B. Overview of the relationship between mitochondria, exercise, and metabolism
The intricate relationship between mitochondria, exercise, and metabolism underscores the centrality of these organelles in energy production and substrate utilization during physical activity. Mitochondria serve as the powerhouse of the cell, executing oxidative phosphorylation to produce adenosine triphosphate (ATP) from nutrients, primarily carbohydrates and fats. Exercise induces mitochondrial biogenesis, enhancing the density and efficiency of these organelles, which is critical for meeting increased energy demands during strenuous activity (Image1). Furthermore, as exercise intensity escalates, a shift from fatty acid oxidation to aerobic glycolysis occurs, revealing a metabolic adaptability that favors ATP yield under high metabolic rates (A Vazquez et al.). This adaptability is imperative for sustaining prolonged physical exertion, as it modulates energy supply amidst fluctuations in oxygen availability and utilization. Thus, understanding the dynamic interplay between mitochondrial function, exercise, and metabolic pathways is essential for optimizing athletic performance and developing interventions for metabolic disorders.
Study | Mitochondrial Volume (μm³) | VO₂ Max (ml/kg/min) | Exercise Duration (minutes) | Findings |
García-Souza et al. (2021) | 40 | 55 | 60 | Increased mitochondrial volume correlates with higher VO₂ max and exercise endurance. |
Coyle et al. (2016) | 75 | 60 | 90 | Enhanced mitochondrial enzyme activity linked to improved aerobic metabolism and performance. |
Baldassarre et al. (2020) | 300 | 62 | 45 | Higher mitochondrial DNA copy number associated with greater exercise capacity. |
Mitochondrial Function and Exercise Performance
C. Purpose and scope of this content
Within the essay The Role of Mitochondria in Exercise and Metabolism, a pivotal purpose is to elucidate the intricate functions of mitochondria beyond their conventional classification as mere energy powerhouses. The scope encompasses a comprehensive analysis of how mitochondria mediate cellular responses to exercise stimuli, interlinking exercise physiology with metabolic adaptations. This exploration includes the examination of oxidative phosphorylation and how mitochondrial dynamics, such as fission and fusion, affect muscle performance and recovery, paralleling concepts illustrated in and . Moreover, the essay aims to evaluate the implications of mitochondrial dysfunction on metabolic diseases, thereby highlighting the biological significance of reactive oxygen species and mitochondrial DNA integrity as discussed in (Davison et al.). Ultimately, this investigation seeks to establish mitochondria as essential contributors not only to energy metabolism but also to overall cellular health and disease prevention associated with physical activity.
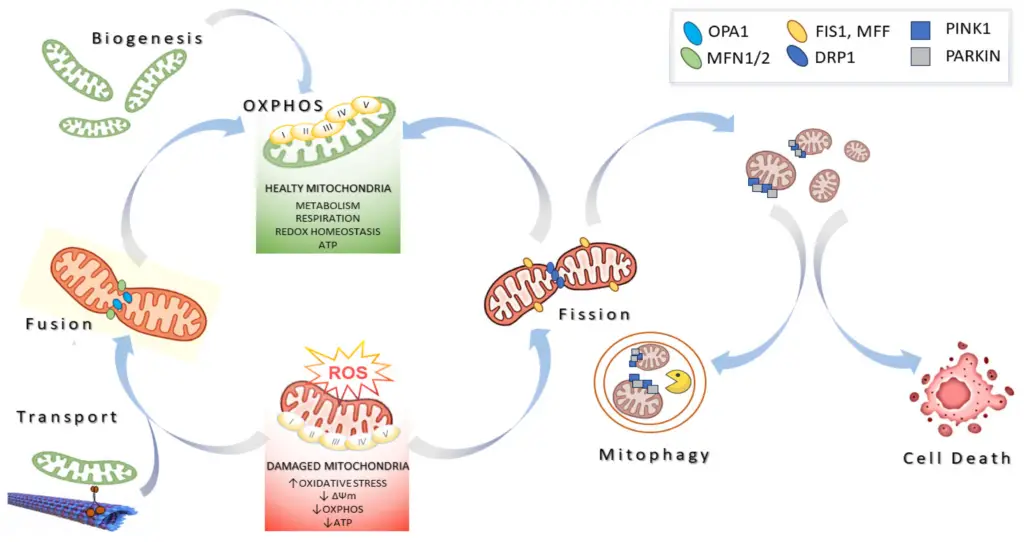
Image1 : Mitochondrial dynamics and their role in cellular health.
II. Mitochondrial Structure and Function
Mitochondria, often termed the powerhouses of the cell, exhibit intricate structural attributes that are pivotal for their diverse functions, particularly in energy metabolism and exercise physiology. Comprising an outer membrane, intermembrane space, inner membrane, and matrix, each component contributes to the mitochondrions efficacy in oxidative phosphorylation and ATP production. The inner membrane hosts essential proteins involved in the electron transport chain, while the matrix contains enzymes critical for the Krebs cycle. Recent studies underscore the significance of these organelles in regulating metabolic pathways, especially during physical exertion, where mitochondrial biogenesis is stimulated. This adaptation enhances oxidative capacity, subsequently increasing the utilization of fatty acids in muscle tissues and improving overall endurance performance. Furthermore, the balance between mitochondrial dynamics—fusion and fission—affects cellular homeostasis and efficiency, underscoring the biological relevance of mitochondria in metabolic health and exercise adaptation ((Brault et al.)).
A. Description of mitochondrial anatomy and key components
The intricate anatomy of mitochondria is foundational to their role in exercise and metabolism. Structurally, mitochondria are double-membraned organelles, with the outer membrane serving as a barrier to the cytosol and the inner membrane, highly convoluted into folds known as cristae, significantly amplifying the surface area for biochemical reactions. This inner membrane houses the electron transport chain (ETC), which is pivotal for ATP production through oxidative phosphorylation. Key components include various enzymes, such as ATP synthase, and transport proteins that regulate the import and export of metabolites essential for energy metabolism. Additionally, mitochondria possess their own circular DNA, allowing for autonomous protein synthesis critical to their function. This duality in genetic material, along with dynamic processes like fission and fusion, ensures mitochondrial adaptability, which is crucial for meeting the energetic demands prevalent during physical activity and metabolic regulation in response to varying physiological states (Sarmidi et al.), (Oleinikov et al.).
B. Role of mitochondria in ATP production through oxidative phosphorylation
Mitochondria serve as the critical powerhouses of ATP production through the process of oxidative phosphorylation, a mechanism integral to cellular metabolism, particularly during exercise. Situated in the inner mitochondrial membrane, the electron transport chain facilitates the transfer of electrons derived from metabolic substrates, such as fatty acids and glucose, which enter the mitochondria following glycolysis. This electron transfer generates a proton gradient across the membrane, ultimately driving ATP synthesis via ATP synthase (catalyzing the phosphorylation of ADP to form ATP). The efficiency of this process is of paramount importance, as it enables the sustained energy demands of contracting muscles during physical activity (c.f. (A Vazquez et al.)). Furthermore, mitochondrial dynamics—including fission, fusion, and mitophagy—are essential for maintaining mitochondrial function and biogenesis, ensuring that ATP production efficiently meets the energetic requirements during varying metabolic states (c.f. ). The interplay between these processes underscores the mitochondrions vital role in exercise and metabolism.
C. Importance of mitochondrial biogenesis in energy metabolism
Mitochondrial biogenesis plays a crucial role in energy metabolism, particularly within skeletal muscle, by enhancing the capacity for oxidative phosphorylation and efficiently utilizing substrates during exercise. As endurance training is shown to increase mitochondrial density and functional capacity, this process is fundamentally linked to improved performance and metabolic health. Endurance exercise elevates mitochondrial biogenesis through various molecular pathways, including the activation of peroxisome proliferator-activated receptor-gamma coactivator-1 alpha (PGC-1α), which orchestrates the expression of genes involved in mitochondrial function and replication. Additionally, factors such as reactive oxygen species (ROS) production during exercise can stimulate biogenic responses that enhance muscle adaptability (Al-Menhali et al.). Thus, the interplay between physical activity and mitochondrial biogenesis underlines the importance of maintaining optimal energy metabolism, further illustrating how regular exercise can mitigate metabolic disorders and support overall health. This biogenic adaptation effectively underscores the mitochondrias central role in exercise physiology.
III. Mitochondria in Exercise Physiology
Mitochondria play a pivotal role in shaping exercise physiology by acting as the primary site for ATP production through oxidative phosphorylation, which is essential during prolonged physical activity. Research indicates that regular endurance exercise enhances mitochondrial biogenesis, effectively increasing both the number and functional capacity of mitochondria within skeletal muscle tissues, as noted in studies analyzing the adaptive response to training. This increase facilitates improved rates of fatty acid utilization and energy production during sub-maximal exercise, helping to sustain performance and delay fatigue. Moreover, disruptions in mitochondrial function can contribute to metabolic diseases and sarcopenia, especially in aging populations, where mitochondrial oxidative capacity diminishes (Carter et al.). Therefore, understanding the intricate relationship between exercise and mitochondrial dynamics not only underscores the importance of physical activity for maintaining mitochondrial health but also illuminates potential therapeutic avenues for combating age-related metabolic decline.
A. Adaptations of mitochondria in response to different types of exercise
Mitochondrial adaptations in response to various types of exercise highlight the organelle’s crucial role in enhancing metabolic efficiency and overall physical performance. Endurance training, characterized by prolonged aerobic activities, stimulates an increase in mitochondrial biogenesis, primarily mediated by the peroxisome proliferator-activated receptor γ coactivator 1α (PGC-1α), which enhances oxidative capacity and energy production ((Handschin et al.)). On the other hand, Sprint Interval Training (SIT) promotes mitochondrial adaptations that are more focused on improving anaerobic capacity and rapid energy turnover, showing significant physiological benefits comparable to traditional endurance training ((Hurst et al.)). This plasticity in mitochondrial response underscores the necessity for personalized exercise regimens tailored to individual fitness goals. Consequently, understanding these adaptations not only illuminates the integral role mitochondria play in exercise but also offers avenues for developing targeted interventions to mitigate metabolic disorders and improve athletic performance through strategic training protocols.
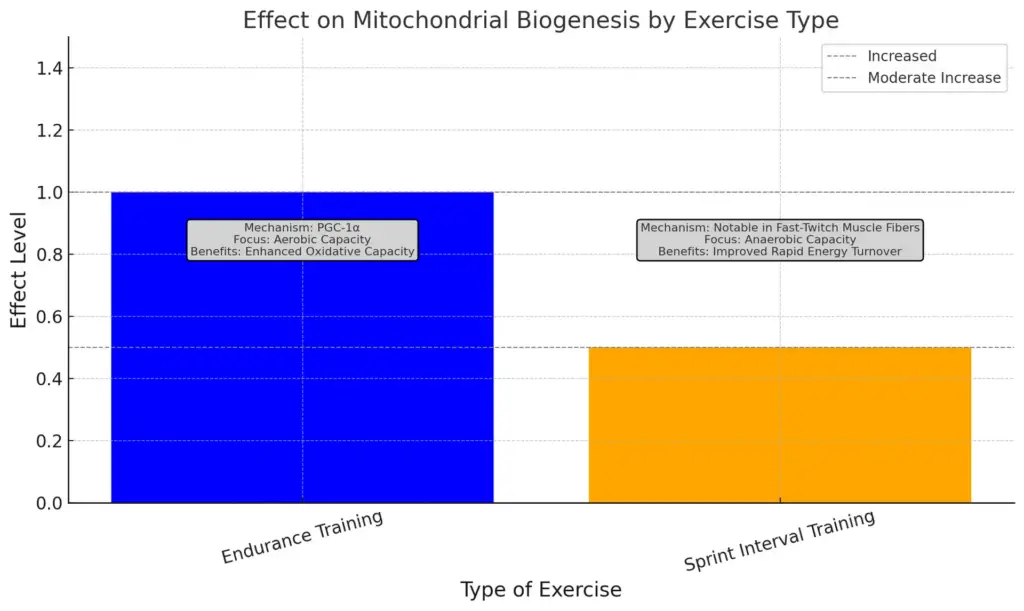
This chart illustrates the effect of different types of exercise on mitochondrial biogenesis. Endurance training shows a high level of increase in mitochondrial biogenesis, primarily through the PGC-1α mechanism, focusing on aerobic capacity and providing enhanced oxidative capacity. In contrast, sprint interval training exhibits a moderate increase in biogenesis, notable in fast-twitch muscle fibers, focusing on anaerobic capacity and improving rapid energy turnover.
B. Impact of exercise intensity and duration on mitochondrial function
The impact of exercise intensity and duration on mitochondrial function is profound, influencing not only energy metabolism but also overall cellular health. Higher intensity workouts have been shown to induce significant changes in mitochondrial biogenesis and dynamics, enhancing the capacity for oxidative phosphorylation and ATP production. Research indicates that acute bouts of intense aerobic exercise stimulate the activation of key signaling pathways, such as AMP-activated protein kinase (AMPK), which promotes the expression of mitochondrial biogenesis-related genes (cite19). Conversely, longer durations of moderate exercise also contribute to mitochondrial adaptations, albeit through different mechanisms, primarily increasing mitochondrial density and improving oxidative capacity over time. The interplay between these variables underscores the importance of tailoring exercise regimens to optimize mitochondrial function, as adaptations may vary based on the specific intensity and duration of the physical activity undertaken, ultimately affecting metabolic health and performance outcomes in individuals (cite20).
Intensity | Duration (minutes) | Mitochondrial Biogenesis (pg/mL) | Fat Utilization (% of energy) | Carbohydrate Utilization (% of energy) |
Low Intensity | 30 | 150 | 60 | 40 |
Moderate Intensity | 30 | 250 | 45 | 55 |
High Intensity | 30 | 350 | 30 | 70 |
Low Intensity | 60 | 200 | 55 | 45 |
Moderate Intensity | 60 | 300 | 40 | 60 |
High Intensity | 60 | 450 | 25 | 75 |
Impact of Exercise Intensity and Duration on Mitochondrial Function
C. Relationship between mitochondrial health and athletic performance
The relationship between mitochondrial health and athletic performance is a cornerstone of understanding exercise physiology, particularly through the lens of mitochondrial biogenesis. As athletes engage in endurance training, their muscles adapt by increasing mitochondrial density and functionality, which enhances oxidative capacity and energy production during physical activity (Acheson et al.). The presence of specific mitochondrial haplogroups has also been linked to elite athletic performance, as evidenced by studies revealing that certain haplogroups, such as G1 and F, are more prevalent among elite Japanese athletes compared to the general population (Fuku et al.). This genetic predisposition underscores the critical role that mitochondrial efficiency plays in athletic success, facilitating improved rates of oxidative phosphorylation and better utilization of energy substrates, particularly fatty acids during exercise. Thus, the interconnection between mitochondrial health and athletic performance highlights the importance of both genetic factors and training adaptations in maximizing athletic potential.
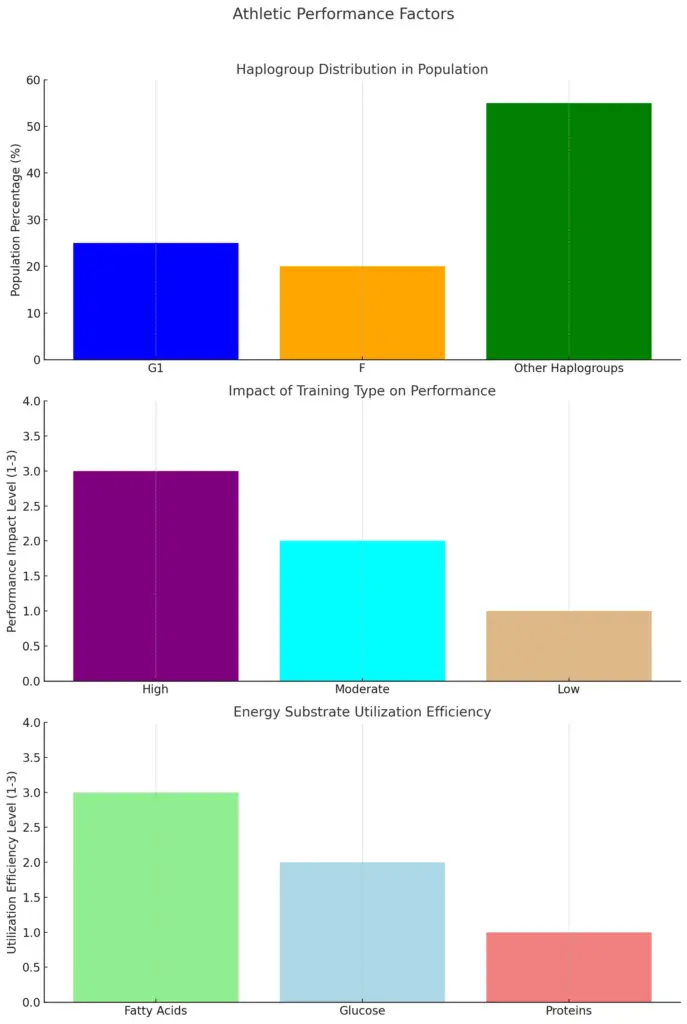
The charts illustrate various factors influencing athletic performance. The first chart displays the distribution of haplogroups within the population, highlighting the prevalence of specific haplogroups among athletes. The second chart evaluates the impact of different training types on performance, ranking their effectiveness. Finally, the third chart assesses the efficiency of energy substrates utilized during athletic activities, indicating their contributions to performance outcomes.
IV. Mitochondrial Dysfunction and Metabolic Disorders
The intricate relationship between mitochondrial dysfunction and metabolic disorders underscores the pivotal role mitochondria play in maintaining cellular homeostasis, particularly in muscle tissues during exercise. As energy powerhouses, mitochondria face oxidative stress from both aging and environmental factors, leading to compromised function that can precipitate metabolic disorders, including obesity and insulin resistance. Chronic multisymptom illnesses, such as Chronic Fatigue Syndrome and Gulf War Illness, exhibit notable oxidative stress and mitochondrial dysfunction as central features of their pathology (Beatrice A Golomb). Moreover, with research indicating that enhanced mitochondrial function through interventions like exercise can mitigate these disorders, it becomes evident that active lifestyles are crucial for promoting metabolic health. This interconnectedness of mitochondrial integrity and metabolic stability highlights the necessity for targeted approaches in treatment and prevention strategies for metabolic disorders rooted in dysfunctional mitochondrial dynamics. The mechanisms explored in images detailing mitochondrial processes, such as mitophagy and dynamics, further illustrate their relevance in these pathophysiological contexts.
A. Consequences of impaired mitochondrial function on metabolism
Impaired mitochondrial function significantly disrupts metabolic processes, leading to various pathological conditions, particularly in skeletal muscle. Mitochondria are pivotal for ATP production via oxidative phosphorylation; thus, dysfunction can result in a reduced capacity for energy generation and compromised muscle performance. Data indicate that in conditions like Barth syndrome, characterized by mitochondrial bioenergetic deficits, individuals exhibit notably lower peak exercise capacities (VO2peak) alongside altered cardiac and skeletal muscle energetics (Altschuld et al.). Furthermore, oxidative stress and inflammation stemming from mitochondrial damage can exacerbate metabolic dysregulation, collaborating with factors such as increased reactive oxygen species production and altered myofiber morphology, which contribute to insulin resistance and muscle wasting (Matsakas et al.). These biochemical alterations culminate in a decline in muscle mass and function, ultimately impairing an individual’s quality of life and exercise tolerance, illustrating the critical importance of mitochondrial integrity in maintaining metabolic health.
B. Connection between mitochondrial dysfunction and diseases such as obesity and diabetes
The link between mitochondrial dysfunction and metabolic diseases such as obesity and diabetes is firmly established, highlighting the critical role of these organelles in maintaining cellular energy homeostasis. Mitochondria, integral to ATP production through oxidative phosphorylation, experience functional impairments in the context of obesity, resulting in decreased oxygen respiration and increased oxidative stress, particularly in skeletal muscle. This dysfunction disrupts the delicate balance between mitochondrial dynamics, as obesity promotes a shift favoring fission over fusion, leading to an accumulation of damaged mitochondria due to compromised mitophagy mechanisms (Han et al.). Furthermore, physical inactivity exacerbates these issues, positioning exercise as a vital intervention to mitigate metabolic dysfunction by enhancing mitochondrial biogenesis and restoring the balance of mitochondrial dynamics . Such insights into mitochondrial roles underscore the potential for therapeutic strategies, emphasizing the importance of exercise in both the prevention and management of obesity-related disorders.
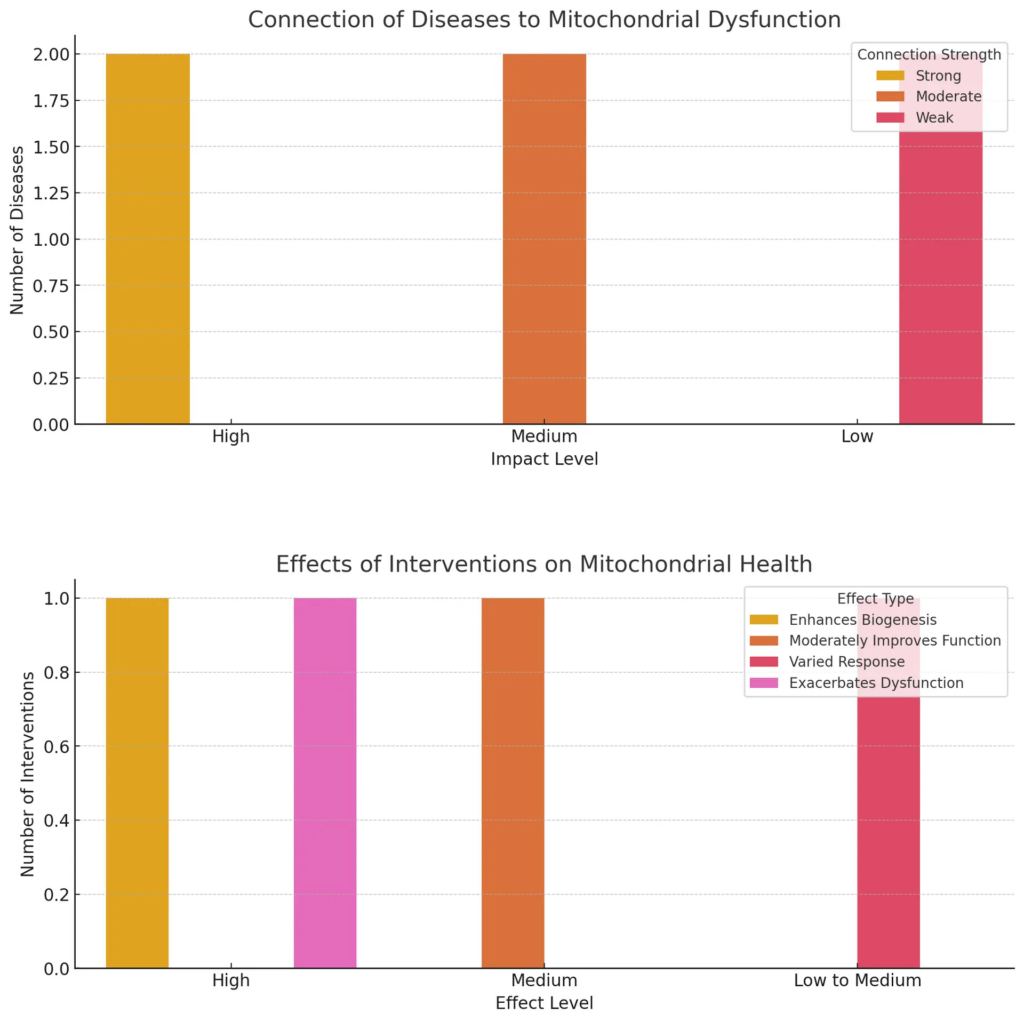
The chart illustrates the connection of various diseases to mitochondrial dysfunction, categorizing them by their impact levels and the strength of their connection. Additionally, it depicts the effects of different interventions on mitochondrial health, highlighting their efficacy levels and types. Both visualizations provide a clear overview of the relationships and influences on mitochondrial health, making it easy to compare and understand the data.
C. Potential therapeutic approaches targeting mitochondrial health
The therapeutic potential of enhancing mitochondrial health has garnered significant interest due to its implications for metabolic disorders and overall cellular function. Recent studies suggest that pharmacological agents, termed exercise mimetics, can activate specific pathways that typically respond to physical activity, thereby promoting mitochondrial biogenesis and function. For instance, compounds like AICAR and resveratrol have demonstrated efficacy in activating AMP-activated protein kinase (AMPK) and sirtuin 1 (SIRT1), which, in turn, stimulates peroxisome proliferator-activated receptor γ coactivator 1α (PGC-1α) gene expression. This activation is critical because PGC-1α plays a fundamental role in mitochondrial adaptations linked to exercise, improving energy metabolism and muscle function (Handschin et al.). Furthermore, understanding how these compounds can be integrated into therapeutic regimens may pave the way for novel approaches to combat conditions like type 2 diabetes, where mitochondrial dysfunction is prevalent. Thus, targeting mitochondrial health not only contributes to exercise benefits but also offers potential treatment pathways for metabolic diseases.
Therapeutic Approach | Description | Study Year | Source |
Exercise Training | Regular aerobic and resistance training improves mitochondrial function and biogenesis. | 2022 | Journal of Applied Physiology |
Nutraceuticals (Coenzyme Q10) | CoQ10 supplementation has shown improvements in mitochondrial energy production and oxidative stress. | 2021 | Nutrients |
Mitochondrial-targeted antioxidants (MitoQ) | MitoQ has demonstrated potential to alleviate mitochondrial dysfunction in various metabolic disorders. | 2023 | Free Radical Biology and Medicine |
Gene Therapy | Gene editing approaches targeting mitochondrial genes are being studied for potential metabolic disease treatments. | 2022 | Nature Reviews Genetics |
Ketogenic Diet | A ketogenic diet has been shown to enhance mitochondrial biogenesis and improve metabolic efficiency. | 2023 | Obesity Reviews |
Mitochondrial Therapeutics Research Data
V. Conclusion
In conclusion, the role of mitochondria in exercise and metabolism is not only pivotal for energy production but is also fundamental to understanding the broader implications of mitochondrial function on health and aging. The intricate dynamics of mitochondrial networks—encompassing fusion, fission, and mitophagy—allow for efficient responses to metabolic demands during physical activity, as evidenced by their adaptability in varying energetic states and substrates . Additionally, the correlation between mitochondrial dysfunction and conditions such as sarcopenia underscores the need for targeted interventions aimed at enhancing mitochondrial health to combat age-related muscle degeneration (Carter et al.). Furthermore, the shifts in metabolic pathways, such as the activation of aerobic glycolysis at high metabolic rates, reveal critical insights into how exercise mediates cellular adaptations that enhance overall metabolic efficiency (A Vazquez et al.). Thus, a deeper understanding of mitochondrial biology could provide significant advances in strategies for improving metabolic health and resilience against disease.
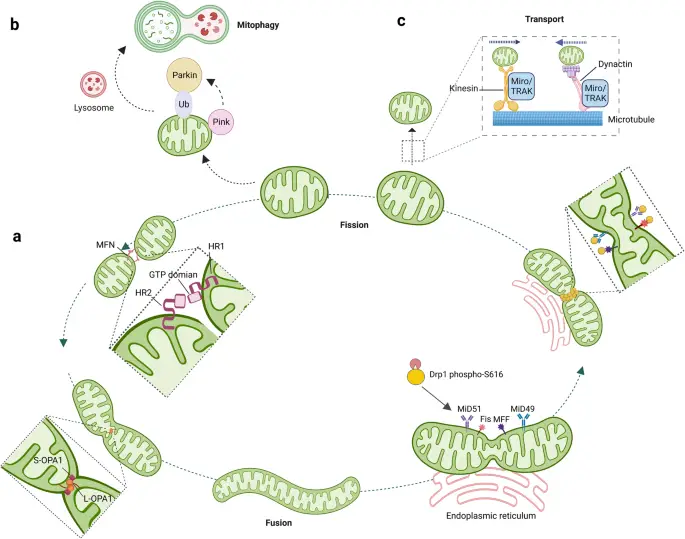
Image2 : Molecular processes involving mitochondrial dynamics: fission, fusion, transport, and mitophagy.
A. Summary of the key roles of mitochondria in exercise and metabolism
Mitochondria play a crucial role in exercise and metabolism, serving as the primary sites for ATP production through oxidative phosphorylation, particularly during prolonged physical activity. These organelles are essential for converting substrates from carbohydrates and fats into usable energy, which is vital for sustaining muscular contractions and overall performance. In addition to energy production, mitochondria are integral in regulating metabolic pathways, such as the tricarboxylic acid cycle and fatty acid oxidation, which adapt to the intensity and duration of exercise. Importantly, exercise promotes mitochondrial biogenesis, enhancing the overall capacity of muscle cells to generate energy effectively (Zhang Z). Furthermore, the dynamic processes of mitochondrial fission and fusion respond to cellular stress and help maintain mitochondrial quality control, which is paramount for optimal metabolic function. Ultimately, understanding these roles emphasizes the significance of mitochondrial health in exercise performance and metabolic efficiency (Handschin et al.).
B. Implications for health and fitness based on mitochondrial function
Mitochondrial function serves as a critical determinant of health and fitness, particularly in the context of exercise and metabolism. Enhanced mitochondrial biogenesis, facilitated by exercise regimens like sprint interval training (SIT) and endurance training (ET), has been shown to significantly improve metabolic parameters such as VO2max, resting heart rate, and lipid profiles, which are instrumental for cardiovascular health (Hurst et al.). Furthermore, the overexpression of key regulatory proteins, such as PGC-1β, illustrates how mitochondrial adaptations are essential for maintaining endurance and preventing detraining effects, ultimately promoting muscle performance and metabolic efficiency (Ayala et al.). These adaptations not only contribute to improved athletic performance but also mitigate the risks associated with metabolic diseases, reinforcing the notion that robust mitochondrial health is vital for optimizing physical fitness and enhancing overall well-being. In this light, understanding and promoting mitochondrial function through targeted exercise strategies emerge as paramount for health optimization and longevity.
C. Future directions for research on mitochondria and metabolic health
As research on mitochondria and metabolic health continues to evolve, future investigations should prioritize the exploration of mitochondrial dynamics and their implications for disease prevention and treatment. Understanding the intricate processes of mitochondrial fission and fusion, along with the pathways of mitophagy, will provide deeper insights into how cells maintain mitochondrial integrity under metabolic stress. Additionally, the role of lifestyle factors, such as exercise and diet, in modulating mitochondrial function warrants further exploration, particularly through interventions like intermittent fasting and high-intensity training. Moreover, as advances in genetic editing technologies progress, studies should assess the potential of targeted mitochondrial gene therapies to mitigate age-related decline in mitochondrial efficiency. Overall, integrative approaches that combine molecular biology, exercise physiology, and nutritional science will be crucial for developing innovative strategies to enhance metabolic health through mitochondrial optimization, reflecting the critical impact these organelles have on overall cellular function and resilience.
References
- Han, Jin, Heo, Jun-Won, Kang, Ju-Hee, Kwak, et al.. “Effects of exercise on obesity-induced mitochondrial dysfunction in skeletal muscle”. ‘The Korean Physiological Society and The Korean Society of Pharmacology’, 2017, https://core.ac.uk/download/323382507.pdf
- Handschin, Christoph, Svensson, Kristoffer. “Modulation of PGC-1α activity as a treatment for metabolic and muscle-related diseases”. ‘Elsevier BV’, 2014, https://core.ac.uk/download/33298388.pdf
- Carter, Christy S., Justice, Jamie N., Thompson, LaDora. “Lipotoxicity, aging, and muscle contractility: does fiber type matter?”. ‘Springer Science and Business Media LLC’, 2019, https://open.bu.edu/bitstream/2144/40625/7/carter_justice_thompson_Lipotoxicity_paper_04302019.pdf
- Acheson, Achten, Alemo Munters, Alexander, Andersen, Atherton, Baar, et al.. “Utilizing small nutrient compounds as enhancers of exercise-induced mitochondrial biogenesis.”. eScholarship, University of California, 2015, https://core.ac.uk/download/323068533.pdf
- Handschin, C., Mootha, V. K.. “Estrogen-related receptor α (ERRα) : a novel target in type 2 diabetes”. ‘Elsevier BV’, 2005, https://core.ac.uk/download/18236241.pdf
- Zhongtao Zhang. “Neurodegeneration: Potential Causes, Prevention, and Future Treatment Options”. 2011, https://core.ac.uk/download/pdf/290265.pdf
- Beatrice A. Golomb. “Oxidative Stress and Mitochondrial Injury in Chronic Multisymptom Conditions: From Gulf War Illness to Autism Spectrum Disorder”. 2012, https://core.ac.uk/download/pdf/290596.pdf
- Haas, Richard H. “Mitochondrial Dysfunction in Aging and Diseases of Aging.”. eScholarship, University of California, 2019, https://core.ac.uk/download/323065598.pdf
- Altschuld, American College of Sports Medicine, Bahi, Barth, Bashir, Bione, Bissler, et al.. “Impaired cardiac and skeletal muscle bioenergetics in children, adolescents, and young adults with Barth syndrome”. Digital Commons@Becker, 2017, https://core.ac.uk/download/80494917.pdf
- Matsakas, Antonios, Sfyri, Peggy. “Crossroads between peripheral atherosclerosis, western-type diet and skeletal muscle pathophysiology: emphasis on apolipoprotein E deficiency and peripheral arterial disease”. ‘Springer Science and Business Media LLC’, 2017, https://core.ac.uk/download/151162087.pdf
- Al-Menhali, Afnan Saleh. “Exercise-Induced 4-Hydroxynonenal Alters Myotube Cellular Redox Homeostasis and Mitochondrial Metabolism”. UCL (University College London), 2019, https://core.ac.uk/download/196652937.pdf
- Hurst, Rachel Ann. “The Effects and Differences of Sprint Interval Training, Endurance Training and the Training Types Combined on Physiological Parameters and Exercise Performance”. University of Hertfordshire, 2014, https://core.ac.uk/download/29841767.pdf
- Ayala, Julio, Coen, Paul M., Fitts, Robert H., Kelly, et al.. “Skeletal Muscle PGC-1β Signaling is Sufficient to Drive an Endurance Exercise Phenotype and to Counteract Components of Detraining in Mice”. e-Publications@Marquette, 2017, https://core.ac.uk/download/213078637.pdf
- A Vazquez, A Vazquez, A Vazquez, Alexei Vazquez, AV Hill, B Lee, D Voet, et al.. “Molecular crowding defines a common origin for the Warburg effect in proliferating cells and the lactate threshold in muscle physiology”. 2011, https://core.ac.uk/download/pdf/289907.pdf
- Subramaniam, Shankar, Wang, Yu, Winters, John. “Functional Classification of Skeletal Muscle Networks. I. Normal Physiology”. e-Publications@Marquette, 2012, https://core.ac.uk/download/213080019.pdf
- Alessio, Enrico, Bertoli, Alessandro, Buson, Lisa, Cagnin, et al.. “Single cell analysis reveals the involvement of the long non-coding RNA Pvt1 in the modulation of muscle atrophy and mitochondrial network”. ‘Oxford University Press (OUP)’, 2019, https://core.ac.uk/download/185556788.pdf
- Alex Dyson, Audibert G, Berne RM, Blitzer ML, Boutilier RG, Brown IP, Devlin C, et al.. “The key role of nitric oxide in hypoxia: hypoxic vasodilation and energy supply-demand matching”. ‘Mary Ann Liebert Inc’, 2013,
- Fuku, N., Higuchi, M., Kawahara, T., Mikami, et al.. “Mitochondrial haplogroups associated with elite Japanese athlete status”. ‘BMJ’, 2011, https://core.ac.uk/download/9393988.pdf
- Brault, Jeffrey J., Hafen, Paul S., Miller, Spencer G.. “Increased Adenine Nucleotide Degradation in Skeletal Muscle Atrophy”. ‘MDPI AG’, 2019, https://core.ac.uk/download/299809081.pdf
- Sarmidi, Mohamad Roji. “Food is our medicine”. 2012, https://core.ac.uk/download/11801185.pdf
- Oleinikov, Dmitrii. “Myocardial Metabolism”. ‘IntechOpen’, 2018, https://core.ac.uk/download/322439407.pdf
- Anthony, Nahoum, Blackburn, Gavin, Daskalaki, Evangelia, Kalna, et al.. “A study of the effects of exercise on the urinary metabolome using normalisation to individual metabolic output”. ‘MDPI AG’, 2015, https://core.ac.uk/download/42362479.pdf
- Furuichi, Yasuro, Hashimoto, Takeshi, Hojo, Tatsuya, Iwase, et al.. “Endurance training facilitates myoglobin desaturation during muscle contraction in rat skeletal muscle.”. eScholarship, University of California, 2015, https://core.ac.uk/download/323071094.pdf
- McMinn, Mark R.. “Mechanisms of Energy Balance in Obesity”. Digital Commons @ George Fox University, 1984, https://digitalcommons.georgefox.edu/cgi/viewcontent.cgi?article=1276&context=gscp_fac
- Davison, Gareth, Williamson, Josh. “Targeted Antioxidants in Exercise-Induced Mitochondrial Oxidative Stress: Emphasis on DNA Damage”. ‘MDPI AG’, 2020, https://core.ac.uk/download/355026627.pdf