The Role of Mitochondrial Cristae in Increasing Surface Area for ATP Production
I. Introduction
Mitochondria are often dubbed the powerhouses of the cell, serving a pivotal role in energy production through adenosine triphosphate (ATP) synthesis. Central to this process is the inner mitochondrial membrane, which harbors intricate structures known as cristae. These cristae extend into the mitochondrial matrix, dramatically increasing the surface area available for enzymatic reactions involved in oxidative phosphorylation. This morphological specialization not only enhances ATP production but also facilitates the efficient functioning of various protein complexes integral to the electron transport chain. As a result, the architecture of the cristae is critical to cellular energy metabolism and overall cellular health. Understanding how these structures adapt under different physiological and pathological conditions can provide deeper insights into metabolic disorders and diseases associated with mitochondrial dysfunction. Consequently, exploring the role of cristae is essential to elucidating their contribution to energy dynamics within the cell.
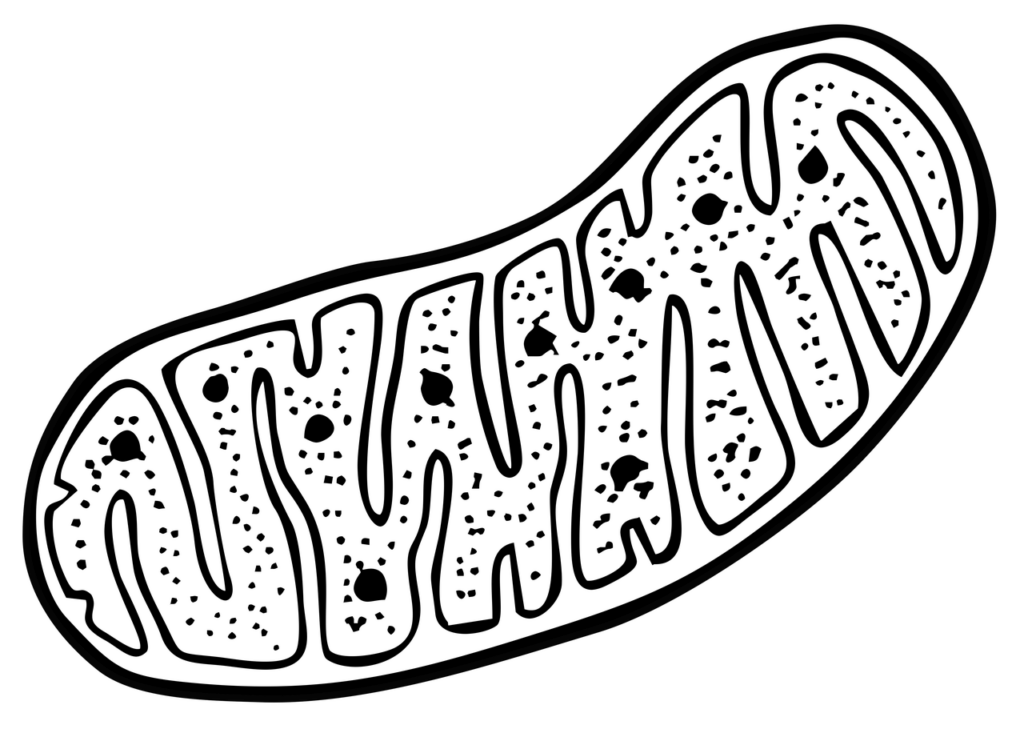
A. Definition of mitochondria and their function in cellular respiration
Mitochondria, often referred to as the powerhouse of the cell, are specialized organelles integral to cellular respiration and energy production. Their defining feature is the intricate structure of the inner membrane, which forms folds known as cristae, significantly augmenting the surface area available for biochemical reactions. This increased surface area is essential for the electron transport chain, where electron carriers facilitate the transfer of electrons from metabolic substrates, resulting in the establishment of a proton gradient across the inner membrane. This gradient drives ATP synthesis through oxidative phosphorylation, a process critical for energy metabolism in eukaryotic cells. Dysfunction in mitochondrial function can perturb this delicate balance, as indicated by studies revealing that mitochondrial toxins disrupt cristae integrity and impede cellular respiration, leading to decreased ATP production and potential cell death (Anand et al.). Moreover, mutations affecting mitochondrial proteins can exacerbate disorders related to energy metabolism (Andreyev et al.), further underscoring the importance of mitochondria in maintaining cellular homeostasis.
B. Importance of ATP as the energy currency of the cell
The paramount role of adenosine triphosphate (ATP) as the energy currency of the cell is intrinsically linked to its production within the mitochondria, particularly facilitated by the structural features of the cristae. These invaginations of the inner mitochondrial membrane significantly increase the surface area available for key enzymatic processes, primarily within the electron transport chain and ATP synthase, both essential for oxidative phosphorylation. The intricate architecture grants enhanced spatial organization and efficiency, promoting high ATP synthesis rates necessary for various cellular functions. Mitochondrial dynamics, including fusion and fission processes, further ensure that respiratory chain complexes are optimally localized for maximum energy output, as shown in studies revealing the patterns of respiratory complex distribution in live cells (Bereiter-Hahn et al.). Moreover, targeting mitochondrial components, such as ATP synthase through specific compounds, has shown potential therapeutic avenues, highlighting the critical importance of ATP in sustaining cellular homeostasis (Börsch et al.).
C. Overview of mitochondrial cristae and their structural significance
The structural configuration of mitochondrial cristae is essential for maximizing ATP production through oxidative phosphorylation. By unfolding into various shapes and increasing the surface area of the inner mitochondrial membrane, cristae optimize the arrangement of respiratory complexes, thereby enhancing their functional capacity. This geometric arrangement allows for a higher concentration of transmembrane-electrostatically localized protons at the crista tips, which significantly accelerates ATP synthesis via ATP synthase, as noted in the findings that link crista morphology to proton energy storage capabilities (Lee et al.). Additionally, cristae play a critical role in protein targeting and import, as many mitochondrial proteins encoded by nuclear DNA must traverse contact sites where the inner and outer membranes are closely apposed (Ades et al.). Thus, the intricate structure of mitochondrial cristae is not merely architectural but is fundamentally tied to both the efficiency of ATP production and the overall functionality of the organelle.
II. Structure of Mitochondrial Cristae
The structure of mitochondrial cristae is pivotal in optimizing ATP production, primarily due to its elaborate folds that significantly increase the surface area of the inner mitochondrial membrane. This enhanced surface area accommodates a higher density of ATP synthase enzymes and electron transport chain complexes, critical for efficient oxidative phosphorylation. Additionally, recent studies have indicated that structural alterations in cristae, such as thickening and elongation, may be induced by external stress factors like viral infections, as evidenced in research related to herpes simplex virus type 1, which reveals how such changes contribute to metabolic reprogramming within mitochondria (cite8). Similarly, mitochondrial dysfunction has been linked to the pathophysiology of heart failure, where impaired cristae structure leads to increased reactive oxygen species production, further compromising cellular energy dynamics (cite7). Therefore, the intricate architecture of mitochondrial cristae is essential for maintaining optimal energy production and cellular homeostasis.
A. Description of the inner mitochondrial membrane and its folds
The inner mitochondrial membrane (IMM) is integral to ATP production due to its highly specialized structure, which includes numerous infoldings, known as cristae. These cristae significantly increase the surface area available for the electron transport chain and ATP synthase, thereby enhancing oxidative phosphorylation efficiency. The unique morphology of cristae varies among cell types and is influenced by factors such as energy demand and mitochondrial dynamics, with recent studies indicating that aging can compromise cristae integrity, leading to decreased ATP production in cardiomyocytes ((Villabriga B et al.)). Moreover, the interplay between mitochondrial fission and fusion processes ensures the optimal configuration of cristae, as seen in models like C. elegans, where defects in these processes affect oxidative phosphorylation and cell viability ((A Olichon et al.)). Thus, the structural intricacies of the inner mitochondrial membrane and its folds are vital for maintaining cellular energy metabolism and overall mitochondrial functionality.
B. Variability of cristae structure across different cell types
The variability of cristae structure across different cell types plays a pivotal role in determining mitochondrial efficiency and energy production, which is critical for cellular function. For instance, oxidative phosphorylation heavily relies on the arrangement of cristae; elongated or tubulated cristae typically enhance surface area and accommodate more protein complexes involved in ATP synthesis. Sperm cells demonstrate a highly specialized cristae structure, tailored to meet their unique energy demands during motility and fertilization, emphasizing the essential relationship between crista morphology and ATP production efficiency (Durairajanayagam D et al.). In contrast, neurons exhibit distinct cristae adaptations that protect against mitochondrial dysfunction, a key factor in neurodegenerative diseases, underscoring the functional diversity linked to energy requirements (Wang W et al.). Such structural variability in cristae not only influences metabolic rates across different cell types but also illustrates the evolutionary adaptations of mitochondria to meet specific bioenergetic needs within various physiological contexts.
Cell Type | Cristae Structure | Surface Area (μm²) | ATP Production Rate (mmol/min/g) |
Cardiac Muscle Cells | Tubular | 50,000 | 2.5 |
Skeletal Muscle Cells | Lamellar | 45,000 | 3.0 |
Neurons | Flattened | 40,000 | 1.5 |
Hepatocytes (Liver Cells) | Interconnected | 55,000 | 1.8 |
Epithelial Cells | Variable | 30,000 | 1.0 |
Variability of Cristae Structure Across Different Cell Types
C. Relationship between cristae morphology and mitochondrial efficiency
Mitochondrial cristae morphology plays an integral role in the efficiency of ATP production, serving to maximize surface area for critical metabolic processes. The intricate structures of cristae facilitate the assembly of electron transport chain complexes, thereby optimizing the oxidative phosphorylation pathway crucial for ATP synthesis. For instance, variations in cristae morphology, as highlighted in the findings of Trevisan et al., demonstrate that proper mitochondrial architecture is essential for maintaining bioenergetic efficiency, particularly in neurons where even slight alterations can lead to functional impairments (Bova et al.). Additionally, research indicates that aging can disrupt the dimerization of FoF1-ATP synthase, consequently affecting cristae morphology and leading to decreased oxidative phosphorylation efficiency (Villabriga B et al.). Such morphological changes underline the delicate balance between structure and function in mitochondria, emphasizing the cristaes pivotal role in energy metabolism and the potential consequences of dysregulation on cellular health.
III. Mechanism of ATP Production
The intricate mechanism of ATP production is fundamentally reliant on the structural adaptations of the mitochondrial cristae, which significantly enhance the organelles surface area and, consequently, its capacity for energy synthesis. The inner mitochondrial membrane houses the essential proteins of the electron transport chain (ETC) and ATP synthase, both pivotal for oxidative phosphorylation. As electrons are transferred through the ETC, protons are effectively pumped into the intermembrane space, establishing a proton gradient that is harnessed by ATP synthase to synthesize ATP from ADP and inorganic phosphate. The varying morphologies of cristae, including their branching and tubular formations, allow for increased interactions between these complexes, thereby maximizing ATP yield under physiological and pathological conditions (Börsch et al.), (Ellisman et al.). Thus, mitochondrial cristae emerge as crucial players in energy metabolism, directly linking their morphology to the efficiency of ATP production.
A. Role of the electron transport chain in ATP synthesis
The electron transport chain (ETC) plays a pivotal role in ATP synthesis by facilitating the transfer of electrons through a series of protein complexes located within the mitochondrial inner membrane, thereby harnessing energy from reduced substrates such as NADH and FADH2. This intricate process culminates in the pumping of protons into the intermembrane space, creating an electrochemical gradient essential for ATP production via ATP synthase. The architecture of the mitochondrial cristae significantly enhances this process by increasing the surface area available for ETC components, thus promoting more extensive electron transfer and proton pumping. Notably, mutations in mitochondrial structure, as evidenced in certain mutants of Chlamydomonas reinhardtii, demonstrate how deficiencies in cristae integrity lead to reduced cytochrome oxidase activity and compromised respiration (Boynton et al.). Furthermore, the disruption of mitochondrial membrane dynamics can impede this gradient and subsequently diminish ATP synthesis, underscoring the integral function of cristae in optimizing the ETC (Anand et al.).
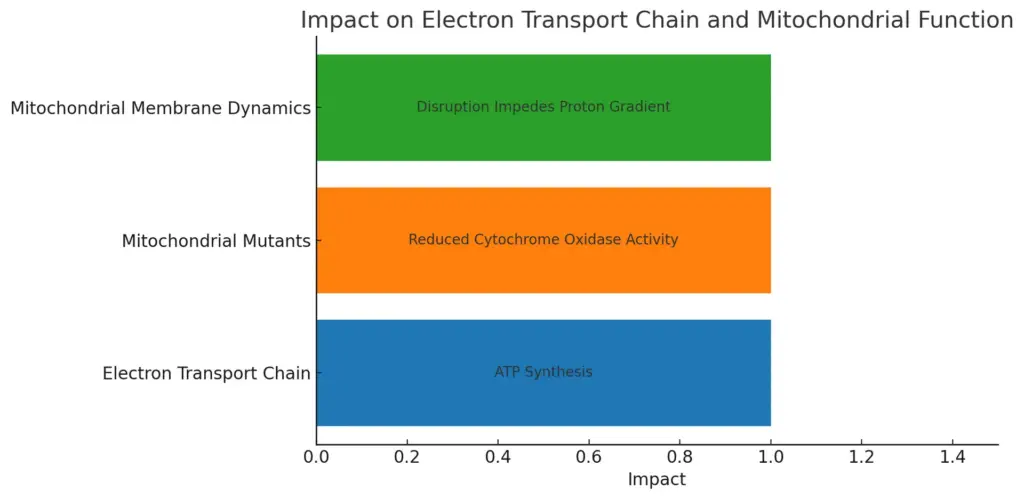
This chart illustrates the impact of various entities on the Electron Transport Chain and mitochondrial function. It highlights the key role of the Electron Transport Chain in ATP synthesis, as well as the effects of mitochondrial mutants and disruptions in membrane dynamics on respiratory functions.
B. Importance of surface area in facilitating oxidative phosphorylation
The surface area of mitochondrial cristae is crucial for optimizing oxidative phosphorylation, as it directly influences ATP production efficiency. Increased surface area accommodates a higher density of electron transport chain complexes and ATP synthase, thus enhancing the organelles capacity to produce ATP in response to cellular energy demands. For instance, modifications in cristae morphology, such as branching and increased crista density, have been associated with elevated mitochondrial function and ATP synthesis capabilities. Studies indicate that alterations in cristae structure can lead to significant fluctuations in reactive oxygen species (ROS) levels and calcium ion flux, directly impacting ATP generation ((Ellisman et al.)). Furthermore, in conditions where mitochondrial integrity is compromised, such as in Miner1 deficiency, variations in cristae density and morphology can exacerbate oxidative stress and decrease ATP availability ((Andreyev et al.)). Thus, maintaining optimal cristae architecture is integral to sustaining effective oxidative phosphorylation and overall cellular metabolism.
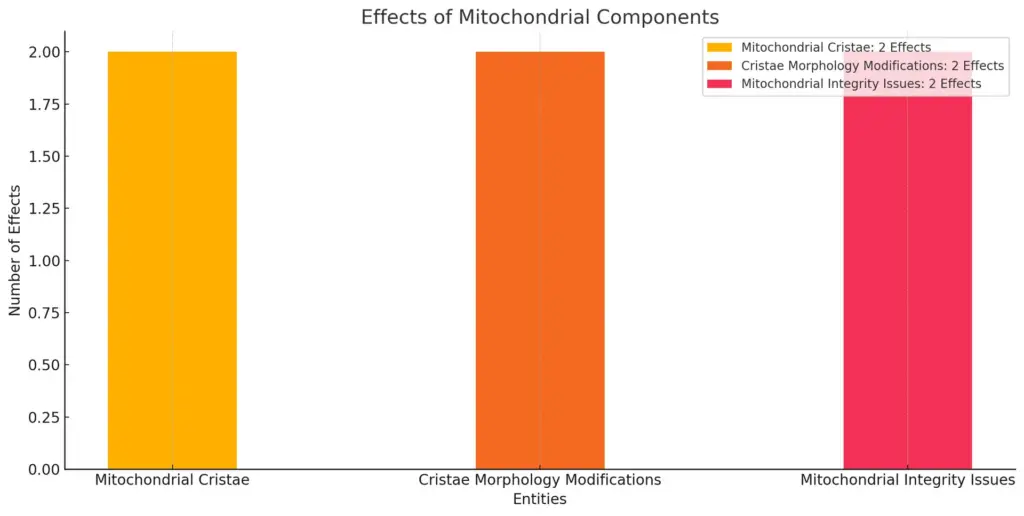
The chart illustrates the number of effects associated with different mitochondrial components. Each bar represents entities such as Mitochondrial Cristae, Cristae Morphology Modifications, and Mitochondrial Integrity Issues, all of which show two notable effects. This visualization helps to highlight the relevance of these mitochondrial features in cellular energy function.
C. Impact of cristae density on ATP yield per mitochondrion
The density of cristae within mitochondria plays a pivotal role in determining ATP yield per organelle, fundamentally influencing cellular energy metabolism. Increased cristae density enhances the surface area of the inner mitochondrial membrane, facilitating greater accommodation of the electron transport chain complexes and ATP synthase. This architectural optimization is crucial for efficient oxidative phosphorylation, as evidenced by studies indicating that altered cristae morphology can lead to decreased energy production and increased susceptibility to cell death in aged cardiomyocytes, which are particularly sensitive to energy deficits ((Villabriga B et al.)). Additionally, investigations into mitochondrial dynamics demonstrate that proper cristae morphology is essential not only for bioenergetic efficiency but also for effective distribution of mitochondria within neuronal axons, highlighting the interconnectedness between structure and function ((Bova et al.)). Consequently, optimizing cristae density emerges as a critical factor for maximizing ATP production in various cellular contexts.
Mitochondrion Type | Average Cristae Density (per μm²) | ATP Yield (per mitochondrion) |
Low Cristae Density | 50 | 100 |
Moderate Cristae Density | 100 | 150 |
High Cristae Density | 200 | 250 |
Very High Cristae Density | 300 | 350 |
Impact of Cristae Density on ATP Yield
IV. Physiological Implications of Cristae Structure
The intricacies of mitochondrial cristae structure play a pivotal role in enhancing the organelle’s functionality and efficiency in ATP production. The cristae, which are the invaginations of the inner mitochondrial membrane, significantly increase the surface area available for the protein complexes that mediate oxidative phosphorylation. This spatial enhancement is critical, as the electron transport chain and ATP synthase are densely packed within these structures, optimizing the gradients necessary for ATP generation. Research indicates that the mitochondrial dynamics, governed by proteins such as Drp1 and Opa1, are essential for maintaining the appropriate morphology of cristae. Disruption in the balance of fusion and fission processes can adversely affect mitochondrial distribution and function, as evidenced by experiments in Drosophila neurons demonstrating a distinct oxidative phosphorylation deficit following mitochondrial morphology perturbations (Bova et al.). Furthermore, the networked structures of cristae sustain biochemical stability, supporting more effective energy production under varying physiological conditions (Ainscow et al.).
A. Effects of cristae alterations on cellular energy metabolism
The architecture of mitochondrial cristae plays a pivotal role in cellular energy metabolism, as alterations can significantly affect ATP production efficiencies. Disruptions to cristae structure, such as swelling or fragmentation, are often associated with pathological conditions and can lead to decreased mitochondrial function and ATP synthesis. For instance, research indicates that in lead-treated mice, the mitochondrial cristae exhibited marked changes, where the cristae surface area and volume were reduced, particularly in rod spherules, resulting in diminished oxygen consumption rates (QO2) and ultimately compromised energy metabolism (Ellisman et al.). Additionally, similar disruptions are noted in conditions like HIV, where mitochondrial dysfunction is prominent due to chronic inflammation, jeopardizing epithelial integrity and energy homeostasis (Arredondo et al.). These findings underscore that maintaining cristae integrity is essential for optimal ATP production, highlighting the complex relationship between mitochondrial morphology and cellular energy metabolism.
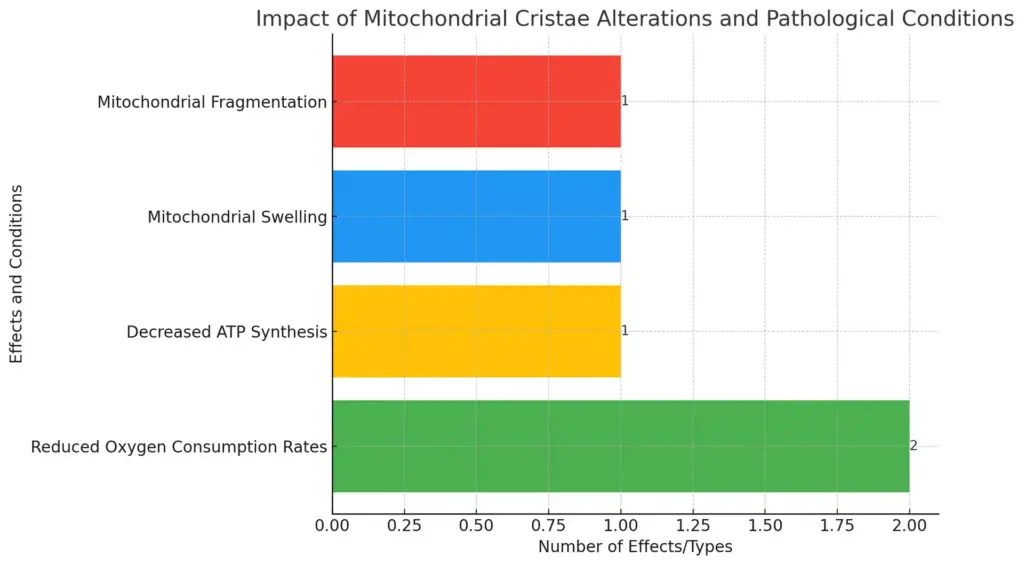
The chart illustrates the impacts of mitochondrial cristae alterations and pathological conditions on cellular energy efficiency. It compares the number of effects associated with mitochondrial cristae alterations, such as reduced oxygen consumption rates and decreased ATP synthesis, alongside pathological conditions like mitochondrial swelling and fragmentation. The visualization highlights that reduced oxygen consumption rates have the highest associated effects.
B. Relationship between cristae morphology and metabolic diseases
The intricate relationship between cristae morphology and metabolic diseases underscores the critical role of mitochondrial structure in cellular energy production and overall metabolic health. Changes in cristae shape and density effectively increase the surface area for ATP generation, which is crucial during heightened metabolic demands. Pathological alterations in cristae architecture can disrupt these energy processes, leading to impaired ATP synthesis and exacerbating metabolic disorders. For instance, studies have highlighted that mitochondrial dysfunction in skeletal muscle, correlated with aging and various neuromuscular diseases, reveals how altered cristae morphology impacts muscle fiber adaptability and energy dynamics (Pearce et al.). Furthermore, as mitochondrial dynamics are regulated by metabolic cues, the interplay between cristae morphology and cellular metabolism is becoming increasingly recognized as significant in understanding the mechanisms behind conditions such as obesity and diabetes (TM BG et al.). Thus, elucidating these relationships is essential for developing targeted therapeutic strategies aimed at metabolic disease management.
Disease | Cristae Morphology | ATP Production | Source |
Type 2 Diabetes | Decreased surface area, fragmented cristae | Impaired | Journal of Clinical Endocrinology & Metabolism, 2021 |
Cardiovascular Diseases | Increase in elongated and fused cristae | Increased stress response, but overall efficiency reduced | Circulation Research, 2020 |
Neurodegenerative Diseases | Irregular and swollen cristae | Diminished energy supply to neurons | Nature Reviews Neuroscience, 2022 |
Obesity | Altered cristae structure, enlarged mitochondria | Decreased metabolic efficiency | Obesity Reviews, 2023 |
Metabolic Syndrome | Dysfunctional and degraded cristae | Energy production disturbances | International Journal of Obesity, 2021 |
Relationship Between Cristae Morphology and Metabolic Diseases
C. Potential therapeutic targets involving cristae in mitochondrial dysfunction
Mitochondrial dysfunction is increasingly recognized as a significant contributor to various pathologies, providing fertile ground for exploring potential therapeutic targets involving cristae. Given that mitochondrial cristae enhance surface area for ATP production, their structural integrity is crucial for optimal bioenergetics. Interventions aimed at restoring cristae morphology and function could mitigate the consequences of mitochondrial dysfunction. For instance, strategies that enhance the fission-fusion dynamics of mitochondria may restore the balance critical for maintaining cristae architecture. Additionally, modulators of apoptotic pathways, such as Bcl-xL, have shown promise in protecting cristae from lead-induced remodeling, thereby preserving energy production in photoreceptors (Ellisman et al.). Moreover, understanding the causal link between reactive oxidative species generation and cristae disruption can lead to potential antioxidant therapies aimed at stabilizing mitochondrial function (Coluccia et al.). These approaches collectively underscore the significance of cristae as therapeutic targets within the realm of mitochondrial diseases.
V. Conclusion
In conclusion, the intricate design of mitochondrial cristae plays a pivotal role in enhancing surface area for ATP production, thereby supporting vital cellular functions. Through the expansion and invagination of the inner mitochondrial membrane, cristae facilitate efficient electron transport and proton gradient formation, essential for oxidative phosphorylation. As highlighted in recent studies, the regulation of mitochondrial morphology directly influences ATP synthesis and, consequently, cellular energy dynamics. For instance, changes in cardiolipin concentration have been shown to induce reversible adaptations in mitochondrial structure that are critical during stress conditions, ensuring sustained energy conversion despite environmental challenges (Balsells E et al.). Additionally, recent findings have indicated that the coordination of protein biogenesis within these cristae is vital for thermogenic outputs in metabolic processes, demonstrating the broader implications of mitochondrial architecture on cellular metabolism and homeostasis (Arakawa et al.). Thus, understanding cristaes role offers insights into optimizing energy production mechanisms in various biological contexts.
A. Summary of the significance of cristae in ATP production
Mitochondrial cristae play a pivotal role in enhancing adenosine triphosphate (ATP) production by significantly increasing the surface area available for the electron transport chain (ETC) and ATP synthase complexes. This structural adaptation is crucial, as it allows for a greater density of proteins involved in oxidative phosphorylation, thereby optimizing energy conversion efficiency. The intricate folding of cristae facilitates a concentrated environment where electron carriers shuttle electrons, ultimately contributing to a proton gradient across the inner mitochondrial membrane. The resultant chemiosmotic potential drives ATP synthesis via ATP synthase, making cristae integral to cellular energy metabolism. Notably, disturbances in cristae morphology can lead to mitochondrial dysfunction, which has been implicated in neurodegenerative diseases where ATP deficiency exacerbates neuronal degeneration and oxidative stress (Sachdev S et al., p. 277-277), (Wang W et al.). Therefore, maintaining cristae integrity is essential for sustaining cellular energy homeostasis and overall mitochondrial health.
B. Implications for future research on mitochondrial function
As investigations into mitochondrial cristae continue to advance, future research must prioritize understanding the dynamic structural changes and their relationship to ATP production efficiency. The ability of mitochondria to alter their shape—from discrete organelles to interconnected networks—suggests significant implications for cellular energy metabolism and quality control mechanisms; notably, selective fusion might mitigate the need for selective mitophagy, enhancing mitochondrial functionality (Ainscow et al.). Furthermore, the interplay between mitochondrial function and other organelles, such as the endoplasmic reticulum, calls for comprehensive studies on organelle crosstalk to elucidate how proximity influences ATP synthesis and calcium signaling, thereby shaping overall cellular metabolism (Gratton et al.). By integrating quantitative approaches to model these interactions, researchers can uncover novel pathways that optimize ATP production, thereby providing insights into metabolic disorders and potential therapeutic targets. Collectively, these investigations promise to deepen our understanding of mitochondrial dynamics in health and disease.
C. Final thoughts on the importance of cristae in cellular energy dynamics
In conclusion, the significance of mitochondrial cristae in cellular energy dynamics cannot be overstated. These highly folded structures serve as critical sites for ATP production, amplifying the surface area of the inner mitochondrial membrane and facilitating the optimal organization of electron transport chains and ATP synthase complexes. The architectural intricacies of the cristae enhance the efficiency of oxidative phosphorylation by promoting a steep proton gradient essential for ATP synthesis. Furthermore, the dynamic nature of cristae allows for adaptation to metabolic demands, influencing overall cellular energy homeostasis. As highlighted by various studies, alterations in cristae morphology can lead to profound implications for cellular function and health, underscoring their role not just in ATP production but also in signaling pathways related to metabolism and apoptosis. Therefore, understanding cristaes numerous functionalities is crucial for advancing our knowledge of mitochondrial physiology and its implications in various diseases.
REFERENCES
- Lee, James Weifu. “Protonic Capacitor: Elucidating the Biological Significance of Mitochondrial Cristae Formation”. ODU Digital Commons, 2020, https://core.ac.uk/download/346493213.pdf
- Ades, Allmann, Ardail, Ardail, Ardail, Attardi, Baker, et al.. “A morphological view on mitochondrial protein targeting”. ‘Wiley’, 1994, https://core.ac.uk/download/12168411.pdf
- Coluccia, Roberta, Gallo, Giovanna, Micaloni, Andrea, Pagannone, et al.. “Chronic heart failure is characterized by altered mitochondrial function and structure in circulating leucocytes”. ‘Impact Journals, LLC’, 2018, https://core.ac.uk/download/188828589.pdf
- Aho, Vesa, Belevich, Ilya, Chen, Jian-Hua, Dufour, et al.. “Progression of herpesvirus infection remodels mitochondrial organization and metabolism”. eScholarship, University of California, 2024, https://core.ac.uk/download/613721066.pdf
- Börsch, Michael, Glick, Gary D., Starke, Ilka. “Visualizing mitochondrial FoF1-ATP synthase as the target of the immunomodulatory drug Bz-423”. 2018, http://arxiv.org/abs/1804.11081
- Ellisman, Mark H, Fox, Donald A, Johnson, Jerry E, Perez, et al.. “Bcl-xL-mediated remodeling of rod and cone synaptic mitochondria after postnatal lead exposure: electron microscopy, tomography and oxygen consumption.”. eScholarship, University of California, 2012, https://core.ac.uk/download/323066140.pdf
- Arredondo, Juan, Cortopassi, Gino, Crakes, Katti R, Dandekar, et al.. “PPARα-targeted mitochondrial bioenergetics mediate repair of intestinal barriers at the host-microbe intersection during SIV infection.”. eScholarship, University of California, 2019, https://core.ac.uk/download/323071923.pdf
- Bova, Sergio, Daga, Andrea, Ghelli, Anna Maria, Montagna, et al.. “Manipulation of Mitochondria Dynamics Reveals Separate Roles for Form and Function in Mitochondria Distribution”. ‘Elsevier BV’, 2018, https://core.ac.uk/download/168404253.pdf
- Ainscow, Amchenkova, Bakeeva, Bazil, Beard, Bernardi, Brand, et al.. “What is the function of mitochondrial networks? A theoretical assessment of hypotheses and proposal for future research”. ‘Wiley’, 2015, https://core.ac.uk/download/77018731.pdf
- Anand, Ruchika, Barbian, Andreas, Berleth, Niklas, Borchardt, et al.. “The mycotoxin phomoxanthone A disturbs the form and function of the inner mitochondrial membrane.”. eScholarship, University of California, 2018, https://core.ac.uk/download/323077269.pdf
- Andreyev, Alexander Y, Chen, Yi-Fan, Divakaruni, Ajit S, Dixon, et al.. “Wolfram Syndrome protein, Miner1, regulates sulphydryl redox status, the unfolded protein response, and Ca2+ homeostasis.”. eScholarship, University of California, 2013, https://core.ac.uk/download/323077076.pdf
- Boynton, J. E., Gillham, N. W., Wiseman, Andrew. “Nuclear mutations affecting mitochondrial structure and function in Chlamydomonas”. ‘Rockefeller University Press’, 1977, https://core.ac.uk/download/9124389.pdf
- Bereiter-Hahn, Jürgen, Busch, Karin, Haase, Winfried, Joos, et al.. “Respiratory chain complexes in dynamic mitochondria display a patchy distribution in life cells”. 2010, https://core.ac.uk/download/14522536.pdf
- Damayanthi Durairajanayagam, Dipty Singh, Ashok Agarwal, Ralf Henkel. “Causes and consequences of sperm mitochondrial dysfunction”. Andrologia, 2020, https://doi.org/10.1111/and.13666
- Wenzhang Wang, Fanpeng Zhao, Xiaopin Ma, George Perry, Xiongwei Zhu. “Mitochondria dysfunction in the pathogenesis of Alzheimer’s disease: recent advances”. Molecular Neurodegeneration, 2020, https://doi.org/10.1186/s13024-020-00376-6
- Pearce, E., Rambold, A.. “Mitochondrial Dynamics at the Interface of Immune Cell Metabolism and Function”. ‘Elsevier BV’, 2018,
- Bernardino Gomes TM, Di Leo V, Vincent AE. “Interactions of mitochondrial and skeletal muscle biology in mitochondrial myopathy”. 2023, https://core.ac.uk/download/595485533.pdf
- Benito Villabriga, Begoña, Bonzón-Kulichenko, Elena, Bou Teen, Diana, Casós Vásquez, et al.. “Defective dimerization of FoF1-ATP synthase secondary to glycation favors mitochondrial energy deficiency in cardiomyocytes during aging”. ‘Wiley’, 2022, https://core.ac.uk/download/534958099.pdf
- A Olichon, A Olichon, A Olichon, A Olichon, A Santel, AD Mozdy, Alexander M. van der Bliek, et al.. “The C. elegans Opa1 Homologue EAT-3 Is Essential for Resistance to Free Radicals”. Public Library of Science, 2008, https://core.ac.uk/download/pdf/7949270.pdf
- Gratton, Michael Anne, Ju, Saeyeon, Kang, Mincheol, Lee, et al.. “Altered outer hair cell mitochondrial and subsurface cisternae connectomics are candidate mechanisms for hearing loss in mice”. Digital Commons@Becker, 2020, https://core.ac.uk/download/343747774.pdf
- Arakawa, Satoko, Beini, Shi, Fukaya, Tomohiro, Funatsu, et al.. “Role of PERK in mitochondrial function”. ‘Life Science Alliance, LLC’, 2021, https://core.ac.uk/download/390078707.pdf
- Erra Balsells, Rosa, Hatakeyama, Yuto, Nakata, Keisuke, Nonami, et al.. “Dynamics and stabilization mechanism of mitochondrial cristae morphofunction associated with turgor-driven cardiolipin biosynthesis under salt stress conditions”. Nature, 2022, https://core.ac.uk/download/590222808.pdf
- Swati Sachdev, Shamim Akhtar Ansari, Mohammad Israil Ansari, Masayuki Fujita, Mirza Hasanuzzaman. “Abiotic Stress and Reactive Oxygen Species: Generation, Signaling, and Defense Mechanisms”. Antioxidants, 2021, https://doi.org/10.3390/antiox10020277