What Are Bacteria? Structure, Types, and Functions Explained
Table of Contents
I. Introduction to Bacteria
Bacteria are the most abundant and diverse microorganisms on Earth, playing crucial roles in various ecological and biological processes. These unicellular organisms, characterized by their simple structure and lack of a true nucleus, exist in myriad environments, from soil and water to the human body. Their versatile nature allows them to adapt and thrive in different conditions, contributing to vital processes such as nutrient cycling, bioremediation, and fermentation. Notably, the human microbiota showcases a complex community of bacteria that significantly influences overall health, as depicted in , which illustrates the microbial composition across various body regions. This image underscores the significance of understanding bacterial diversity and function, emphasizing their roles not only in health but also in diseases when microbial imbalances occur. Thus, exploring the structure, types, and functions of bacteria is essential for comprehending their integral contributions to both the environment and human health.
Type | Characteristics | Examples | Habitat |
Eubacteria | True bacteria; peptidoglycan cell wall; diverse metabolic processes. | Escherichia coli, Streptococcus pneumoniae | Soil, water, surface of organisms |
Archaebacteria | Ancient bacteria; lack peptidoglycan; often extremophiles. | Methanogens, Halophiles | Hot springs, salt lakes, deep sea vents |
Cyanobacteria | Photosynthetic bacteria; produce oxygen; have chlorophyll. | Anabaena, Microcystis | Freshwater, marine environments |
Bacteria Classification and Characteristics
A. Definition of Bacteria
Bacteria are unicellular microorganisms characterized by their prokaryotic cell structure, meaning they lack a defined nucleus and membrane-bound organelles. These organisms can be found in diverse environments, ranging from deep-sea vents to the human gut, reflecting their remarkable adaptability and ecological significance. Bacteria can be classified based on various criteria, including shape (such as cocci, bacilli, and spirilla), Gram staining properties, and metabolic functions, leading to a complex taxonomy that facilitates their study. In the context of human health, certain bacterial types perform essential roles, such as aiding in digestion or producing necessary vitamins, exemplifying their functional importance. Moreover, considering their presence and variation in different body regions, as depicted in , underscores the intricate relationships bacteria maintain within their environments, including symbiotic associations with hosts. Thus, bacteria not only embody a fundamental aspect of life on Earth, but also serve crucial roles in health and disease dynamics.
Characteristic | Description |
Definition | Bacteria are single-celled microorganisms that can exist either as free-living organisms or as parasites. |
Cell Type | Prokaryotic, meaning they do not have a nucleus or other membrane-bound organelles. |
Size | Typically range from 0.5 to 5 micrometers in length. |
Reproduction | Primarily reproduce asexually through binary fission. |
Habitat | Found in diverse environments including soil, water, and inside living organisms. |
Role in Ecosystem | Play crucial roles in nutrient cycling and decomposing organic matter. |
Bacteria Characteristics
B. Where Bacteria Are Found
Bacteria are ubiquitous organisms that inhabit a diverse array of environments, both in and on the human body as well as in the external world. Within the human body, specific bacterial populations are tailored to reside in various regions, such as the gut, oral cavity, skin, and even the respiratory tract. This distribution is critical for maintaining homeostasis and promoting overall health, reflecting a complex interplay between human physiology and microbial ecosystems. For instance, the balanced microbiota of the gut plays a significant role in digestion and immune function, while the skin hosts unique bacterial communities that can protect against pathogens. The visualization provided in underscores the distinct microbial compositions across these anatomical sites, highlighting how specific bacteria contribute to bodily functions. Understanding where bacteria are found not only enhances our comprehension of microbial ecology but also emphasizes their potential implications for human health and disease prevention.
Habitat | Bacteria Species | Estimated Population (per gram) |
Soil | Nitrosomonas, Pseudomonas | 10 million – 1 billion |
Water | Aquifex, Cyanobacteria | 1 million – 30 million |
Human Gut | Bacteroides, Lactobacillus | 100 billion – 1 trillion |
Extreme Environments (Hot Springs, Deep Sea) | Thermophiles, Halophiles | 1 million – several billion |
On Skin | Staphylococcus, Propionibacterium | 1,000 – 10 million |
Bacterial Habitats
II. Structure of Bacteria
Understanding the structure of bacteria is crucial for comprehending their functional complexities and diverse roles in various environments, including the human body. Bacteria are typically unicellular organisms characterized by a prokaryotic cell organization, which lacks a membrane-bound nucleus. Instead, their genetic material is contained within a nucleoid region, presenting a significant difference from eukaryotic cells. The bacterial cell wall, primarily composed of peptidoglycan, not only provides structural support but also differentiates between gram-positive and gram-negative bacteria—features that are pivotal in medical microbiology and antibiotic treatment. The presence of structures such as pili, flagella, and capsules further reflects the adaptability and survival mechanisms of bacteria in varying conditions. Incorporating knowledge about the structural differences among bacterial species can unveil their functional capabilities, as illustrated by the complex interactions of microbiota within human microbiomes, effectively contextualized in . Understanding this structural basis ultimately aids in the exploration of bacterias roles in health and disease.
Component | Function | Example | Occurrence |
Cell Wall | Provides shape and protection | Peptidoglycan in Gram-positive bacteria | Present in most bacteria |
Cell Membrane | Regulates the movement of substances in and out of the cell | Phospholipid bilayer | Present in all bacteria |
Cytoplasm | Houses cellular components and metabolic processes | Aqueous solution containing enzymes and nutrients | Present in all bacteria |
Nucleoid | Contains genetic material (DNA) | Circular DNA strand | Present in all bacteria |
Ribosomes | Synthesizes proteins | 70S ribosomes | Present in all bacteria |
Capsule | Provides additional protection and helps in adherence | Polysaccharide layer in some bacteria | Not present in all bacteria |
Flagella | Facilitates movement | Long, whip-like structures | Present in some bacteria |
Structure of Bacteria
A. Cell Wall and Membrane
The cell wall and membrane of bacteria serve critical roles in maintaining cellular integrity and function, acting as barriers that regulate the transport of substances in and out of the cell. The bacterial cell wall, primarily composed of peptidoglycan, provides structural support and protects against osmotic pressure, which is crucial for survival in varying environments. Bacteria are categorized into two main types based on their cell wall structure: Gram-positive, which have a thick peptidoglycan layer, and Gram-negative, characterized by a thinner layer and an outer membrane that can impede antibiotic penetration. This distinction is key to understanding how bacteria interact with their surroundings and respond to antimicrobial agents. The importance of this structural feature is underscored by its variability among different bacterial species, illustrated effectively in , which outlines the microbiota composition across various regions of the human body and the role of specific bacterial types relevant to health and disease. Thus, the cell wall and membrane are not only pivotal for bacterial protection but also influence their symbiotic and pathogenic relationships within diverse ecosystems.
Bacteria Type | Cell Wall Composition | Cell Membrane | Common Examples |
Gram-Positive | Thick Peptidoglycan Layer | Single Membrane | Staphylococcus, Streptococcus |
Gram-Negative | Thin Peptidoglycan Layer, Outer Membrane | Double Membrane | Escherichia coli, Salmonella |
Mycoplasma | No Cell Wall | Cell Membrane Present | Mycoplasma pneumoniae |
Archaea | Pseudopeptidoglycan or Other Polymers | Unique Lipids | Methanogens, Halophiles |
Bacterial Cell Wall and Membrane Characteristics
B. Cytoplasm and Organelles
In the complex structure of bacteria, the cytoplasm and organelles play crucial roles in maintaining cellular functions and facilitating survival in diverse environments. The cytoplasm serves as a gel-like matrix that suspends various organelles, enzymes, and molecules essential for metabolic processes. Within this environment, ribosomes are particularly important as they synthesize proteins required for bacterial growth and response to environmental stimuli. Additionally, while bacteria lack membrane-bound organelles found in eukaryotic cells, they do possess specialized structures such as inclusions and nucleoid regions, which store genetic material and nutrients. This adaptation highlights the efficiency of bacterial organization, allowing them to thrive despite limited structural complexity. To better understand the implications of these components, the image depicting microbiota composition across different regions of the human body, , effectively illustrates the diverse roles bacteria play in various environments, reinforcing the importance of cytoplasm and organelles in the broader context of bacterial function and identity.
C. DNA and Plasmids
A fundamental aspect of bacterial biology is the structure and function of their genetic material, specifically DNA and plasmids. Unlike eukaryotic cells, bacteria possess a singular circular DNA molecule housed in the nucleoid region, which includes essential genes necessary for survival and replication. Additionally, many bacteria contain plasmids—small, circular, double-stranded DNA molecules that can replicate independently of chromosomal DNA. These plasmids often carry genes that confer advantageous traits, such as antibiotic resistance or the ability to metabolize unusual substrates, thus allowing bacteria to adapt to varying environments. The implications of plasmids extend beyond individual survival; they play a significant role in horizontal gene transfer, enabling genetic exchanges between bacteria, thereby promoting diversity and evolution. This dynamic is crucial for understanding bacterial adaptability and the emergence of pathogenic strains. For a visual representation of the diversity of bacterial communities, the microbiota composition in various regions of the human body is effectively illustrated in .
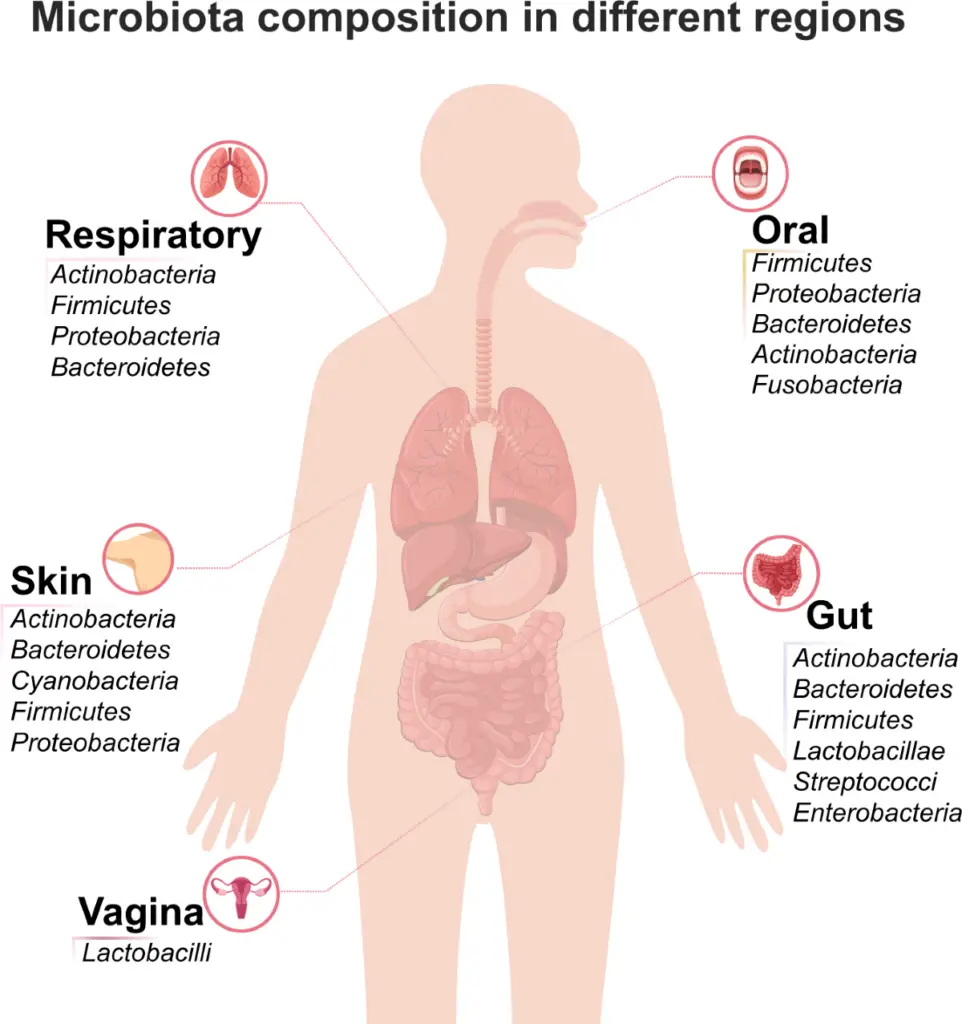
Image : Microbiota Composition in Human Body Regions (The image illustrates the composition of microbiota in various regions of the human body, focusing on five distinct areas: the respiratory system, oral cavity, skin, gut, and vagina. Each section is visually marked with an anatomical reference and lists the predominant bacterial phyla and genera found in that region, including Actinobacteria, Firmicutes, Proteobacteria, Bacteroidetes, Fusobacteria, Lactobacilli, and Streptococci. This representation aids in understanding the diversity and distribution of microbiota, which can have implications for health and disease.)
III. Types of Bacteria
The classification of bacteria is crucial in understanding their diverse roles in ecosystems and human health. Broadly, bacteria can be categorized into several types based on their shapes, gram-staining properties, and metabolic processes. Common shapes include cocci (spherical), bacilli (rod-shaped), and spiral forms, each associated with different ecological niches and functions. Furthermore, bacteria are classified as either gram-positive or gram-negative, which reflects their cell wall structure and influences their reaction to antibiotics, thereby impacting treatment strategies for bacterial infections. A specific illustration of this diversity can be seen in the composition of microbiota across various regions of the human body, as depicted in . Understanding these types is essential for both clinical applications and ecological studies, as the interactions between different bacterial types can influence health outcomes and contribute to the stability of microbiomes within different environments. Such classifications facilitate targeted research and interventions in fields ranging from medicine to environmental science.
A. Gram-Positive vs. Gram-Negative
The distinction between Gram-positive and Gram-negative bacteria primarily hinges on their cell wall composition, which significantly influences their biochemical behavior and pathogenic potential. Gram-positive bacteria possess a thick peptidoglycan layer that retains the crystal violet stain used in Gram staining, resulting in a purple coloration under a microscope. This thick layer offers structural support and protection, making them more resilient to environmental stresses. In contrast, Gram-negative bacteria have a thin peptidoglycan layer and are surrounded by an outer membrane containing lipopolysaccharides, which contribute to their weaker staining characteristics, rendering them pink. This outer membrane not only acts as a barrier to certain antibiotics but also plays a crucial role in the bacterias ability to evade the host immune system. Understanding these differences is essential for developing targeted antimicrobial therapies. The complexity of bacterial interactions and their implications for human health is vividly illustrated in , which provides insight into the diverse roles of different bacterial types in various body regions.
Characteristic | Gram Positive | Gram Negative |
Cell Wall Structure | Thick peptidoglycan layer | Thin peptidoglycan layer and an outer membrane |
Response to Gram Stain | Stains purple | Stains pink |
Toxins Produced | Usually produce exotoxins | Usually produce endotoxins |
Examples | Staphylococcus aureus, Streptococcus pneumoniae | Escherichia coli, Salmonella enterica |
Sensitivity to Antibiotics | Generally more susceptible to penicillin | More resistant to antibiotics due to outer membrane |
Comparison of Gram-Positive and Gram-Negative Bacteria
B. Aerobic vs. Anaerobic
Understanding the distinction between aerobic and anaerobic bacteria is crucial for comprehending bacterial physiology and its implications for various biological processes. Aerobic bacteria thrive in environments rich in oxygen, utilizing it for cellular respiration to generate energy, a process that is significantly more efficient than anaerobic mechanisms. Conversely, anaerobic bacteria function optimally in oxygen-poor environments where they resort to fermentation or anaerobic respiration to metabolize organic matter. This divergence in metabolic pathways has profound effects on both the environment and human health, as aerobic bacteria play critical roles in decomposition and nutrient cycling, while anaerobic bacteria contribute to processes such as fermentation in the gut. The image , which illustrates the diverse microbiota across various regions of the human body, reinforces the significance of these bacterial types. It highlights how both aerobic and anaerobic bacteria coexist and interact within different niches, offering insights into their respective functions and the balance essential for maintaining health.
Type | Definition | Examples | Oxygen Requirement | Function | Common Habitats |
Aerobic | Bacteria that require oxygen for metabolism. | Mycobacterium tuberculosis, Pseudomonas aeruginosa | Obligate Aerobes and Facultative Anaerobes | Break down organic material with oxygen to produce energy. | Soil, water, and aerobic regions of the human body. |
Anaerobic | Bacteria that do not require oxygen and may even be harmed by it. | Clostridium botulinum, Bacteroides fragilis | Obligate Anaerobes and Facultative Anaerobes | Ferment organic compounds to produce energy without oxygen. | Deep sediment, gastrointestinal tracts, and anaerobic environments. |
Aerobic vs Anaerobic Bacteria Comparison
C. Pathogenic vs. Beneficial Bacteria
The distinction between pathogenic and beneficial bacteria is crucial in understanding their roles within ecosystems and human health. Pathogenic bacteria, such as Streptococcus pneumoniae, can cause diseases ranging from mild infections to severe illnesses, emphasizing the need for effective medical interventions. In contrast, beneficial bacteria, particularly those found in our microbiota, contribute positively to our physiological functions, including digestion and immune system modulation. For instance, various Lactobacillus species help maintain gut health by fermenting dietary fibers, thus producing short-chain fatty acids that nourish colon cells and suppress harmful bacteria. The interconnectedness of these bacterial types highlights the delicate balance within the human microbiome, where the presence of beneficial bacteria can often outcompete potentially harmful pathogens. Such complexity is visually represented in , which showcases the microbiota composition across different regions of the body, illustrating the diverse roles bacteria play in sustaining health and combating disease.
Type | Examples | Diseases Caused | Estimated Annual Deaths (US) | Sources |
Pathogenic Bacteria | Escherichia coli (E. coli), Salmonella, Streptococcus pneumoniae | Food poisoning, Pneumonia, Urinary tract infections | 10 | CDC, WHO |
Beneficial Bacteria | Lactobacillus, Bifidobacterium, Streptococcus thermophilus | Aid in digestion, Produce vitamins, Boost immune system | 1,000 species, trillions of cells | Harvard Health, NIH |
Antibiotic Resistance | Methicillin-resistant Staphylococcus aureus (MRSA), Vancomycin-resistant Enterococcus (VRE) | Increased healthcare costs, Extended hospital stays | 2 | CDC, WHO |
Pathogenic vs. Beneficial Bacteria
IV. Functions of Bacteria in Ecosystems
Bacteria are indispensable to the functioning of ecosystems, fulfilling various vital roles that maintain ecological balance. One of their primary functions involves nutrient cycling, particularly in the decomposition of organic matter. As decomposers, bacteria break down dead plant and animal materials, releasing essential nutrients back into the soil, which promotes the growth of new life. Additionally, certain bacteria engage in nitrogen fixation, a process that converts atmospheric nitrogen into a form usable by plants. This capability not only enriches soil fertility but also enhances plant diversity and productivity. Moreover, bacteria participate in biogeochemical cycles, influencing carbon, sulfur, and phosphorus cycles, thus playing a crucial part in energy flow within ecosystems. Their presence can also facilitate symbiotic relationships with other organisms, exemplified in the human gut, where they assist digestion and bolster immune function. Thus, the diverse functions of bacteria underscore their critical role in sustaining and regulating ecosystem health.
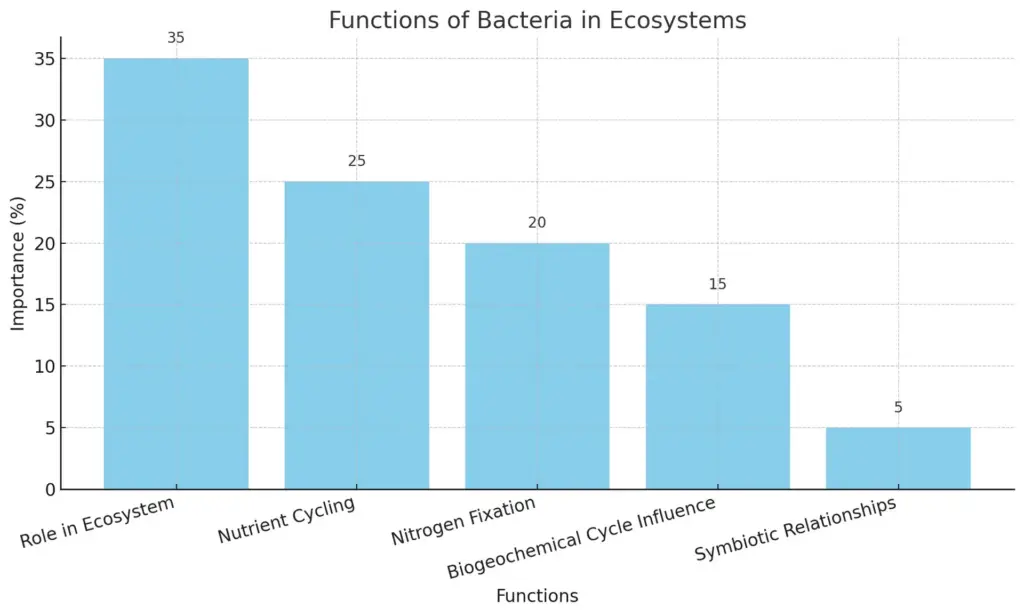
This bar chart represents the various functions of bacteria in ecosystems, highlighting their vital roles such as nutrient cycling, nitrogen fixation, influence on biogeochemical cycles, and symbiotic relationships, which collectively contribute to ecological balance and health.
A. Decomposers and Nutrient Cycling
Bacteria play a pivotal role in the process of decomposition and nutrient cycling, acting as essential decomposers in various ecosystems. As heterotrophic organisms, they break down organic matter, such as dead plants and animals, thereby recycling vital nutrients back into the soil. This biological activity not only contributes to soil fertility but also ensures the continuous availability of nutrients for plant growth, which is fundamental for maintaining ecological balance. The enzymatic capabilities of various bacterial species enable them to metabolize complex organic compounds and convert them into simpler substances, facilitating nutrient availability for autotrophs. Furthermore, this decomposition process is intricately linked to broader nutrient cycles, such as the nitrogen and carbon cycles, where bacteria mediate key transformations. The importance of this relationship is underscored by visual representations of microbial diversity in different ecological niches, as illustrated in . Thus, understanding these processes enhances our appreciation of the intricate roles bacteria occupy within various ecosystems.
B. Symbiotic Relationships
Symbiotic relationships involving bacteria are crucial for maintaining ecological balance and promoting health within larger biological systems. These relationships can be classified into mutualism, commensalism, and parasitism, each reflecting different degrees of interaction between bacteria and their hosts. In mutualistic relationships, both organisms benefit; for instance, gut bacteria assist in digesting food while obtaining nutrients in return. Commensalism, on the other hand, benefits one organism without harming the other, seen in skin bacteria that derive nutrients from sweat while not interfering with human health. Parasitism, conversely, involves one organism benefiting at the expense of the other, potentially leading to disease. The diverse microbiota composition across various regions of the human body exemplifies these intricate interactions, as highlighted in . Understanding these symbiotic relationships is vital, as they play a fundamental role in human health and disease prevention, emphasizing the importance of bacteria beyond traditional views as mere pathogens.
C. Industrial and Medical Uses
Bacteria play an indispensable role in various industrial and medical applications, showcasing their versatility and importance in contemporary society. In industry, bacteria are employed in bioremediation, where they decompose pollutants in contaminated environments, thus demonstrating their potential in environmental sustainability. Additionally, their ability to ferment carbohydrates is harnessed in the production of biofuels, beverages, and dairy products, underscoring their significant contributions to the food and energy sectors. In the medical field, certain bacteria are utilized in the development of probiotics, which enhance gut health and bolster the immune system, as illustrated in . Furthermore, advancements in genetic engineering have allowed for the exploitation of bacteria in the production of insulin, vaccines, and antibiotics, highlighting their critical role in pharmaceuticals. Overall, the diverse functions of bacteria in industrial and medical contexts not only reflect their biological importance but also emphasize their potential to address pressing global challenges.
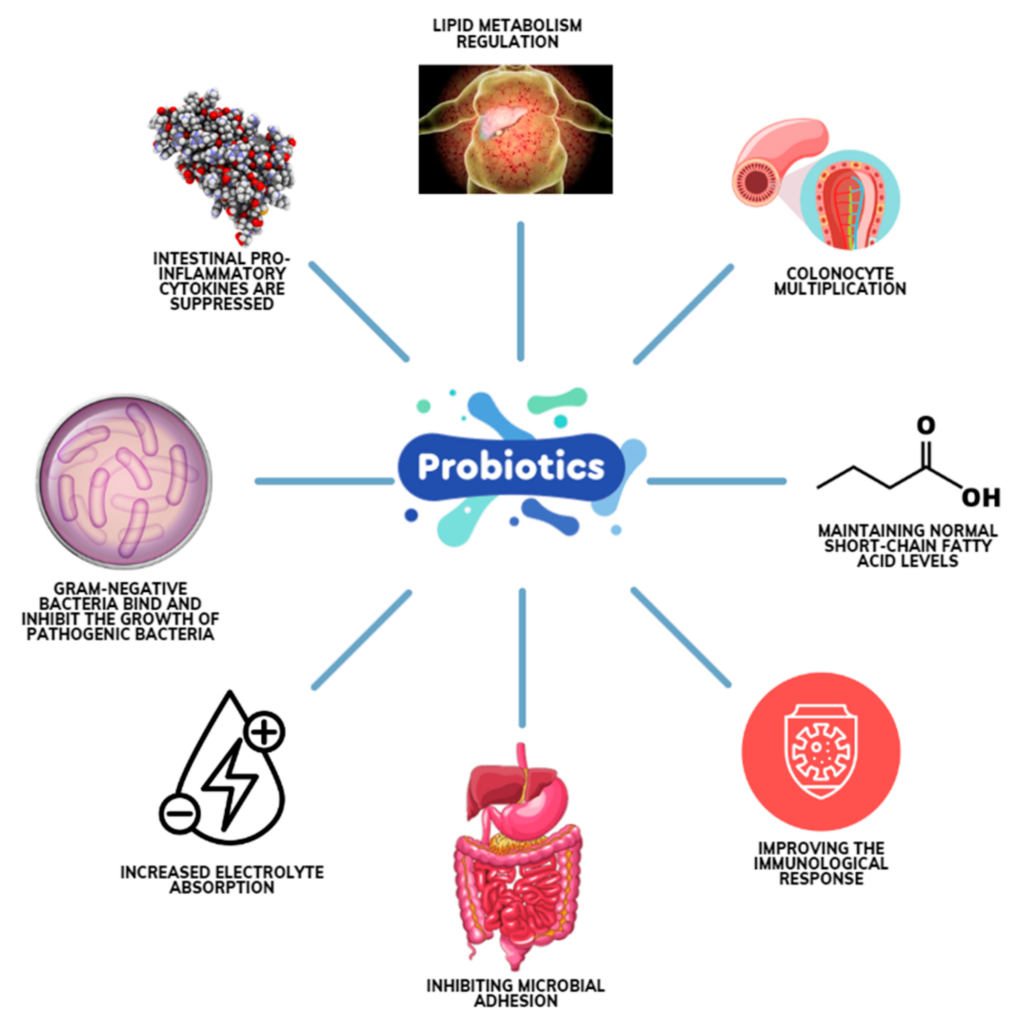
Image : Health Benefits of Probiotics (The image illustrates the multifaceted benefits of probiotics in human health. Central to the image is the term ‘Probiotics,’ surrounded by various effects that probiotics have on the body. These include suppression of intestinal pro-inflammatory cytokines, regulation of lipid metabolism, enhancement of colonocyte multiplication, binding of gram-negative bacteria to inhibit pathogenic bacteria, increased electrolyte absorption, maintenance of normal short-chain fatty acid levels, improvement of the immunological response, and inhibition of microbial adhesion. The visual elements combine biological illustrations with labels, making it a concise reference for understanding the impact of probiotics on gastrointestinal health and overall well-being.)
V. Conclusion
In summation, the intricate world of bacteria underscores their fundamental roles in various ecosystems, human health, and disease processes. As diverse microorganisms, bacteria not only contribute to essential biochemical cycles but also play pivotal roles within the human microbiome, influencing digestion, immunity, and even mental health. The varied structures and types of bacteria, including their adaptive features, emphasize their evolutionary significance and functional versatility. Additionally, understanding the specific distributions of bacterial populations, as illustrated in the microbiota composition image , enhances our comprehension of their localized functions and interrelations within the human body. This exploration reveals how these microscopic entities serve as both allies and adversaries in health, highlighting the critical balance required for maintaining homeostasis. Ultimately, recognizing the multifaceted nature of bacteria prompts further investigation into their applications in medicine, agriculture, and environmental science, thus paving the way for innovative solutions to contemporary challenges.
A. Summary of Key Points
In summary, understanding bacteria is essential due to their vast diversity, structural characteristics, and multifaceted roles in various ecosystems, including human health. The intricate relationships among different bacterial species and their environments underscore the importance of knowing how these microorganisms interact with one another and with their hosts. For example, the microbiota composition across different regions of the human body, as shown in , highlights the specialized functions of distinct bacterial communities, revealing their contributions to immunity and metabolic processes. Furthermore, the health benefits attributed to probiotics elucidate the therapeutic potentials of certain bacterial strains, reinforcing the significant influence of bacteria on overall well-being. By exploring the structure, types, and functions of bacteria, we gain insights into their critical roles in maintaining ecological balance and supporting human health, which underscores the necessity of ongoing research in microbiology and related fields.
B. Future Research in Bacteriology
As bacteriology continues to evolve, future research is poised to unlock new dimensions in our understanding of these microorganisms and their multifaceted roles in health and disease. In particular, investigations into the human microbiome and its interaction with various bacterial communities will shed light on how specific bacteria contribute to gastrointestinal health, immune function, and overall well-being. This research is becoming increasingly vital, as targeting the microbiome offers promising therapeutic avenues, such as probiotic interventions, that could enhance patient outcomes in conditions ranging from autoimmune disorders to mental health issues. Furthermore, the exploration of antibiotic resistance mechanisms in bacteria demands urgent attention; understanding these pathways may lead to the development of novel antimicrobial strategies. Visual representations, such as , illustrating the intricate composition of human microbiota across different body regions, will facilitate clearer communication of these complex interactions, thereby supporting scholarly discourse aimed at advancing bacteriology.
REFERENCES
- National Academy of Engineering. ‘Microbiomes of the Built Environment.’ A Research Agenda for Indoor Microbiology, Human Health, and Buildings, National Academies of Sciences, Engineering, and Medicine, National Academies Press, 10/6/2017
- R. Hakenbeck. ‘Bacterial Cell Wall.’ J.-M. Ghuysen, Elsevier, 2/9/1994
- Arthur L. Koch. ‘The Bacteria: Their Origin, Structure, Function and Antibiosis.’ Springer Science & Business Media, 9/20/2007
- Board on Global Health. ‘Microbial Evolution and Co-Adaptation.’ A Tribute to the Life and Scientific Legacies of Joshua Lederberg: Workshop Summary, Institute of Medicine, National Academies Press, 5/10/2009
- Alistair McCleery. ‘An Introduction to Book History.’ David Finkelstein, Routledge, 3/13/2006
Image References:
- Image: Microbiota Composition in Human Body Regions, Accessed: 2025.https://media.springernature.com/lw1200/springer-static/image/art%3A10.1038%2Fs41392-022-00974-4/MediaObjects/41392_2022_974_Fig1_HTML.png
- Image: Health Benefits of Probiotics, Accessed: 2025.https://www.mdpi.com/microorganisms/microorganisms-12-00234/article_deploy/html/images/microorganisms-12-00234-g001.png