The Biology of Microtubules: A Comprehensive Overview
- I. Introduction
- II. Structure of Microtubules
- III. Functions of Microtubules
- IV. Microtubule-Associated Proteins (MAPs)Regulation of microtubule dynamics by MAPs
- V. Microtubules in Disease
- VI. Microtubules in Specialized Cells
- VII. Microtubules in Biotechnology and Medicine
- VIII. Microtubule Dynamics and Regulation
- IX. Conclusion
- References:
- Image References:
I. Introduction
Microtubules have complex structures that are essential for many cell processes, making them key parts of the cytoskeleton in eukaryotic cells. These tube-like polymers are mostly made of tubulin dimers and help with different cell functions like keeping cell shape, helping transport within cells, and supporting cell division. Microtubules are not static; they change often, helping cells adjust to new environments and growth needs. Their role goes beyond just being structural, as they are also important for cell signaling and organelle movement. This introduction lays the groundwork for understanding the various roles microtubules have in cell biology, preparing for a deeper look into their biology, how they work, and their effects on health and disease, especially regarding processes such as mitosis and the development of neurodegenerative diseases.
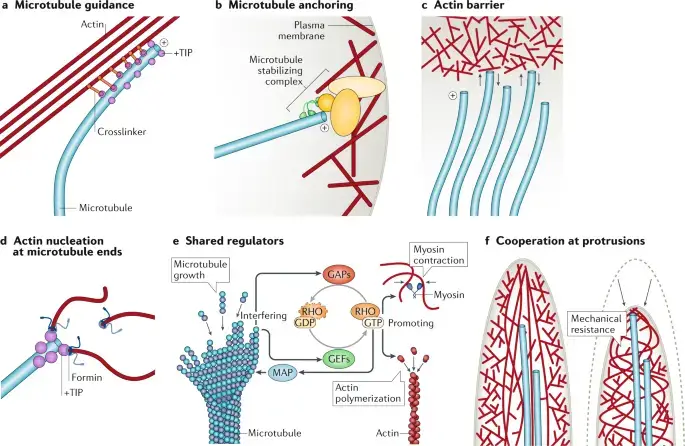
Image1 : Cellular mechanics: Interactions between microtubules and actin (The image presents a detailed schematic representation of various cellular mechanisms, specifically focusing on microtubule and actin interactions within the cellular architecture. Each panel (labeled a through f) illustrates different processes, including microtubule guidance, anchoring to the plasma membrane, formation of actin barriers, nucleation processes at microtubule ends, shared regulatory mechanisms involving RHO proteins, and the cooperation of actin and myosin in cellular structures. These diagrams are essential for understanding cytoskeletal dynamics, regulatory interactions, and mechanical properties of cellular structures.)
A. Definition and significance of microtubules
Microtubules are tube-like structures made of tubulin protein dimers that are important for keeping cells stable and helping with inner cell tasks. Their ability to quickly build up and break down allows microtubules to play key roles in cell processes like mitosis, during which they create the mitotic spindle to help separate chromosomes correctly. Besides being structural elements, microtubules also act as paths for moving organelles and cell parts, supported by motor proteins like kinesin and dynein. Their importance is highlighted in studies showing that changes in microtubule behavior can impact cancer growth, especially in castration-resistant prostate cancer, where treatments focused on microtubule function have become essential (Georgiev et al.), (Berman-Booty et al.). In the end, learning about microtubules helps us better understand cell functions, providing a basis for possible treatments for different illnesses.
B. Overview of microtubule structure and function
Microtubules, being the biggest parts of the cytoskeleton, have a tube-like shape made from α- and β-tubulin dimers joining together, giving them a width of about 25 nm. This changing structure is important for many cell activities, like keeping cell form, helping move things inside the cell, and managing cell division. Microtubules have a specific orientation with plus and minus ends that helps direct motors such as kinesins and dyneins, which carry materials across microtubule routes. This movement is affected by changes in tubulin after it is made and by microtubule-associated proteins (MAPs) that control their stability and arrangement (*Ackmann et al.). New research shows that the setup of microtubules can differ based on the situation, as shown by how Patronin, which aids in maintaining minus-end growth, is key for filling dendrites with microtubules that have their minus ends outward, highlighting how microtubules can adapt to specific cell environments (Albertson et al.).
C. Importance of studying microtubules in cellular biology
Studying microtubules is important for knowing how cells work because they play a key role in many cellular activities like moving materials inside the cell, cell division, and keeping the cell’s shape. Microtubules act like tracks for motor proteins, which help in shifting organelles, vesicles, and signaling molecules around the cell, ensuring everything functions properly (Ellisman et al.). Their ability to quickly assemble and disassemble is crucial for cell movement and division, linking them to important stages of the cell cycle and overall development of organisms (Risler et al.). Additionally, when microtubule structure is disrupted, it can lead to various diseases, such as neurodegenerative conditions and cancer, highlighting their significance in medical research and treatment plans. Therefore, studying microtubules not only deepens our knowledge of basic biological processes but also guides potential medical uses.
II. Structure of Microtubules
Microtubules have a complex structure that is very important for their many roles in eukaryotic cells. They are made up of α- and β-tubulin heterodimers and can quickly change, showing a fast assembly and disassembly process that is key for cell activities like mitosis and the transport within the cell. Their tube-like shape is about 25 nm wide, giving them strength but also some flexibility, which helps them support the cell’s structure (Ballarin et al.). Moreover, microtubules act as pathways for motor proteins such as kinesin and dynein, helping move organelles and vesicles around inside the cytoplasm (Georgiev et al.). This varied role highlights how important it is for microtubules to be organized and stable in keeping the cell functioning properly. Therefore, understanding microtubule structure is important for realizing their vital roles in cell biology and development.
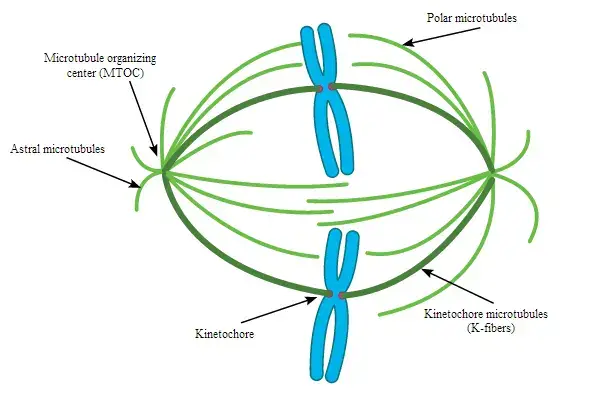
Image2 : Schematic representation of the mitotic spindle in cell division. (The image illustrates the structure of the mitotic spindle during cell division, highlighting key components such as the microtubule organizing center (MTOC), astral microtubules, polar microtubules, kinetochores, and kinetochore microtubules (K-fibers). This diagrammatic representation is essential for understanding the mechanics of chromosome separation and the role of microtubules in mitosis. The use of color coding helps in distinguishing different types of microtubules involved in the process.)
A. Tubulin heterodimers (α-tubulin and β-tubulin) as building blocks
At the center of microtubule structure is the main unit, which is tubulin heterodimers made of α-tubulin and β-tubulin. These dimers come together to create protofilaments, which then line up side by side to make the hollow tube shape found in mature microtubules. The flexible nature of microtubules is greatly affected by post-translational modifications (PTMs) of tubulin proteins, which boost their functional variety and adaptability in cells. For instance, the cycle of detyrosination and retyrosination in α-tubulin is important for controlling microtubule stability and movement, which is vital for cell functions like division and transport inside the cell (Zhang et al.). Furthermore, the different amounts of tubulin isotypes and various PTMs form a tubulin code, which is read by microtubule-associated proteins (MAPs), helping cells respond specifically to changes in their environment (Carmona et al.). This complex interaction highlights the crucial role of tubulin heterodimers as key components in how microtubules function biologically.
B. Protofilaments and their arrangement in the microtubule wall
The strength of microtubules comes from how their protofilaments are arranged. Usually, a microtubule contains 13 protofilaments that line up in parallel, creating a hollow tube that helps support and maintain the cell’s structure. This setup is not just random; it is based on how tubulin molecules interact with each other. Studies show that protofilaments have a strong 4 nm repeat because of how tubulin monomers are spaced out and a weaker 8 nm repeat due to the unique shapes of alpha and beta tubulin subunits, which together create a solid microtubule form (Doerhoefer et al.). Additionally, there is evidence that a left-handed superhelix is created when the protofilaments are slightly tilted, which allows more protofilaments to fit in (Langford et al.). This complex design highlights how flexible and functional microtubules are in cells.
C. Polarity of microtubules (plus and minus ends)
Microtubules have a clear polarity with distinct plus and minus ends, which is an important feature that affects how they behave and interact with motor proteins. This polarity is important for transport inside cells, where motor proteins like kinesins and dyneins move vesicles along the microtubules, with kinesins generally going to the plus end and dyneins moving to the minus end (Xu et al.). The way microtubules grow in a directional manner helps processes in cells that depend on these transport systems, such as moving organelles and proteins, which is especially essential in neurons where transport needs to be stable (Ciocanel et al.). Additionally, the dynamic instability of plus-ends allows for quick assembly and disassembly, providing cells with the ability to change their cytoskeletal structure as necessary. Therefore, understanding the polarity of microtubules helps explain not just their structural roles but also their contributions to important biological functions.
D. Dynamic instability: growth and shrinkage of microtubules
Dynamic instability is a key feature of microtubules, showing how they can grow and shrink based on cell needs. This process is affected by different factors, including severing proteins like katanin and spastin, which add randomness to microtubule behavior. Recent models indicate that while severing events do not greatly change the total number of microtubules, they do alter the lengths’ distribution, influencing cellular activities (B Alberts et al.). Moreover, external substances, such as S-methyl-D-DM1, can upset this balance by changing the rates of microtubule catastrophe and rescue, pointing to possible treatments for cancer. The complex relationship between the growth and shrinkage of microtubules highlights their active nature and their important role in supporting cellular structure and function (Correia J J et al.).
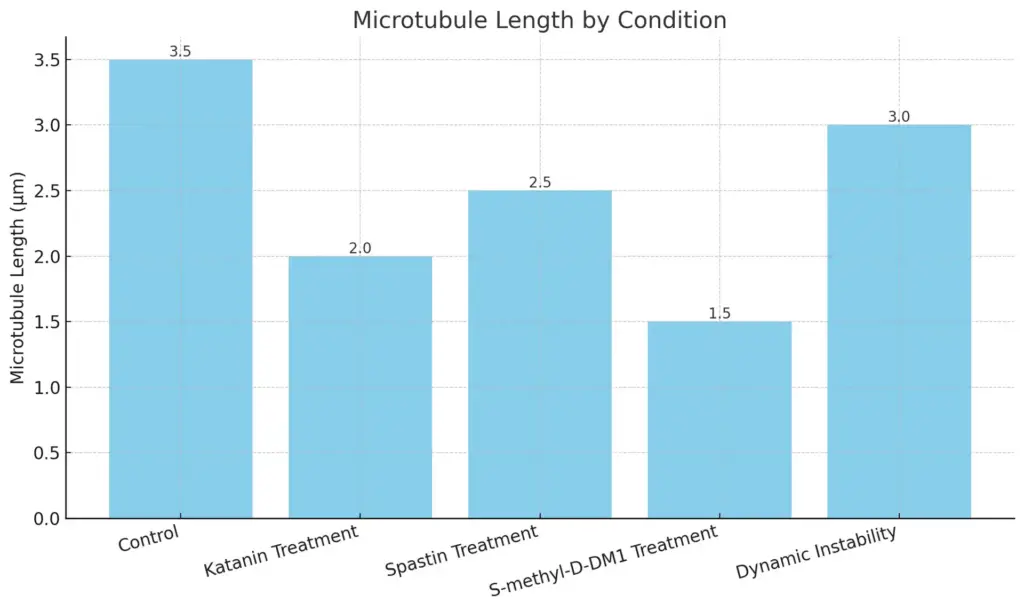
The chart displays the microtubule lengths (in micrometers) across different experimental conditions. Each bar represents a condition, with its height corresponding to the measured length. The chart provides a visual comparison of how the various treatments affect microtubule length.
III. Functions of Microtubules
Microtubules are important for many cell functions, helping to shape eukaryotic cells and enabling essential activities like transport within cells and division of cells. Made up of tubulin dimers, these tube-like structures are key parts of the cytoskeleton, offering support and helping organelles and vesicles move. The changing nature of microtubules permits quick rearrangements to meet the cell’s needs, especially during mitosis when they create the mitotic spindle, which is critical for proper chromosome separation (Drechsler et al.). Furthermore, recent studies show that microtubules are vital for cellular signaling and maintaining cell polarity, affecting everything from development to brain function (Alberts et al.). Therefore, microtubules not only help keep cells sturdy but also are crucial for communication and movement within the complex cell environment, highlighting their many roles in cell biology.
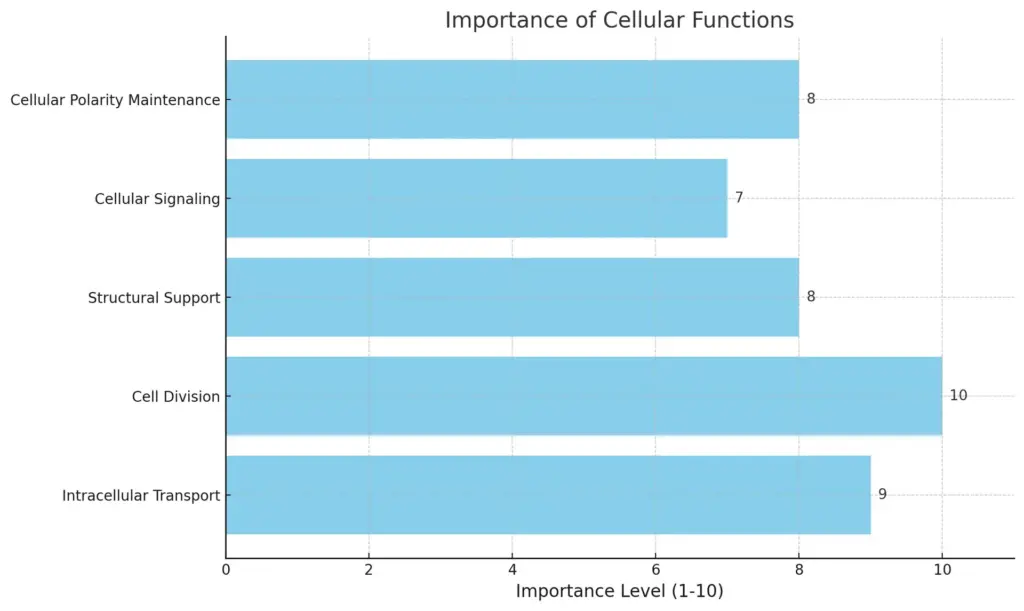
his chart illustrates the importance levels of various cellular functions, as rated on a scale of 1 to 10. The bar chart emphasizes that “Cell Division” is rated the highest at 10, while “Cellular Signaling” has the lowest rating at 7. Other functions are rated between 8 and 9, highlighting their significant roles in cellular processes.
A. Role in cell shape and mechanical support
In cellular structure, microtubules are an important part in keeping cell shape and providing support. Their formation into different structures is key for the strength and stability of various cell types. For example, research shows that while microtubule activity can be affected in conditions like near weightlessness, plant cells can still arrange microtubules neatly, due to the extra support from the cell wall ((Emons et al.)). This connection highlights how microtubules and the extracellular matrix work together to define cell shape. Additionally, in nerve cells like PC12 neurites, the support from microtubules is vital for controlling size and shape, since their damage can lead to swelling and changes in shape ((Alberts et al.)). Therefore, microtubules are crucial not just for keeping cell shape but also for providing the mechanical strength needed for cell functions.
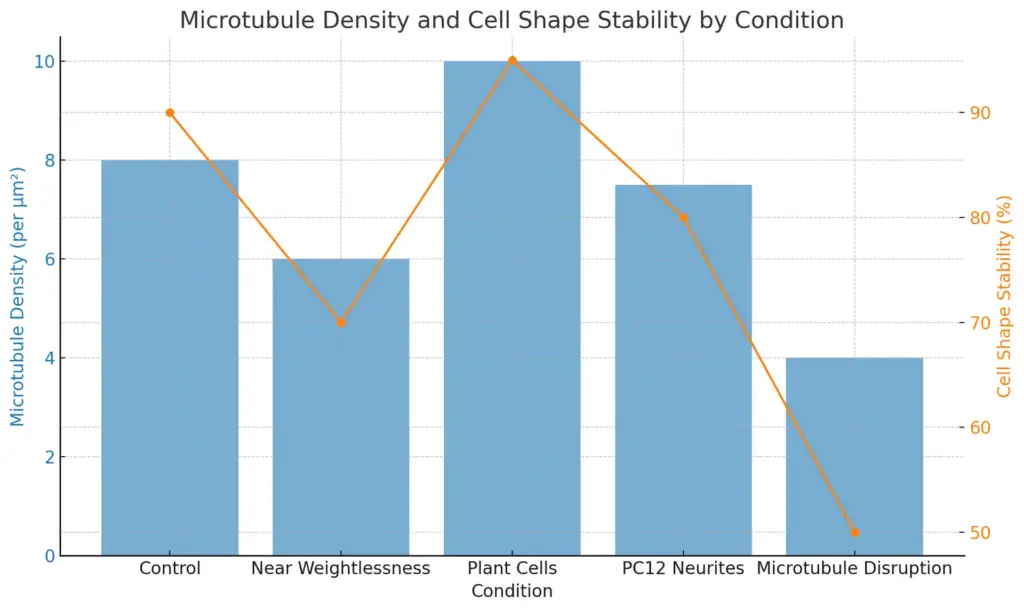
The chart displays the relationship between microtubule density and cell shape stability across different conditions. The blue bars represent microtubule density (per μm²), while the orange line indicates cell shape stability in percentage. The data reveals variations in both measures across the tested conditions, highlighting the impact of environmental factors on cellular properties.
B. Involvement in intracellular transport mechanisms
Intracellular transport systems are very important for keeping cells working properly, and microtubules are key parts of this system. These flexible structures help move organelles, proteins, and other cell parts through molecular motors like kinesin and dynein, which use microtubules as pathways. The specificity of this transport is highlighted by special Rab GTPases, for example, Rab11B, which is crucial for sending vesicles from the Golgi to the Inner Membrane Complex during Toxoplasma gondii replication. This process showcases the link between microtubule structure and vesicle transport, showing how the organization of the cytoskeleton is important for proper cell function ((A Colosimo et al.)). Also, since cells function as systems that need energy, understanding the details of transport based on microtubules helps to shed light on broader cellular operations, including effects in disease conditions like neurodegenerative disorders ((Appert-Rolland et al.)).
C. Contribution to cell division and mitotic spindle formation
The relationship between microtubules and mitotic spindle formation is very important for cell division to happen. Microtubules are structures that change during the cell cycle; they break down and reorganize to create the mitotic spindle, which is necessary for the correct separation of chromosomes. During interphase, microtubules spread out from the microtubule-organizing center to build a network in the cytoplasm, which helps keep the cell stable and gets it ready for mitosis. However, as cells move into mitosis, this network breaks down to form a well-organized mitotic spindle structure. This structure has microtubule bundles on the outside that help with aligning and moving chromosomes during anaphase, as seen in studies of Stephanopyxis turris (Cande et al.). Additionally, the targeted addition of proteins like Gravin to the spindle poles has been found to affect spindle orientation and how accurately the cell divides, showing how complex microtubule interactions are in keeping genomic stability (Bucko et al.).
IV. Microtubule-Associated Proteins (MAPs)Regulation of microtubule dynamics by MAPs
Microtubule dynamics are regulated by microtubule-associated proteins (MAPs). These proteins are important for stabilizing, organizing, and helping build microtubules. They enable the complex interactions between microtubules and other parts of the cell, which greatly affect how the cell is structured and how it works. For instance, TPX2 (Targeting Protein for Xklp2) is a key MAP that is necessary for microtubule formation and the assembly of the mitotic spindle. It works as an activator of Aurora kinases, which are crucial for correct cell division (Demidov et al.). This connection highlights how MAPs are crucial in keeping microtubule dynamics well-regulated during important cell processes, like mitosis, where correct chromosome separation is essential for keeping genomic integrity. Additionally, learning about these proteins’ many roles gives us knowledge about the evolutionary changes needed for complex cellular functions in eukaryotes, as shown by their varied roles in different species (Drechsler et al.).
A. Types of MAPs (e.g., stabilizing, destabilizing, severing proteins)
Microtubule-associated proteins (MAPs) are important for controlling how microtubules change, which is key for keeping cells intact and helping with transport inside cells. These proteins can be split into three groups: stabilizing, destabilizing, and severing MAPs, each with a different role in organizing microtubules. Stabilizing MAPs, like tau, attach to microtubules and help them form, increasing their stability during crucial processes such as cell division. On the other hand, destabilizing MAPs, such as katanin, cut and break down microtubules, enabling quick changes to the cytoskeleton when needed. Katanin works by recognizing the tails at the end of tubulin and using ATP to do its cutting job, which is very important for cell functions, especially in neurons where microtubule changes are complicated (Bailey et al.). Through this careful balance of functions, MAPs help the microtubule cytoskeleton adjust to the different demands of the cell, as shown by the regulatory mechanisms in microtubule studies (Tyler et al.).
Type | Examples | Function | Impact |
Stabilizing Proteins | Tau, MAP2 | Promote microtubule assembly and stability | Increased microtubule polymerization |
Destabilizing Proteins | Catastrophin, Stathmin | Facilitate microtubule depolymerization | Increased microtubule turnover |
Severing Proteins | Katanin, Spastin | Cut microtubules into shorter fragments | Rapid restructuring of microtubule networks |
Types of Microtubule-Associated Proteins (MAPs)
B. Functions of key MAPs (e.g., tau, kinesin, dynein, stathmin)
The roles of important microtubule-associated proteins (MAPs) like tau, kinesin, dynein, and stathmin are key for keeping cells stable and helping with transport inside cells. Tau helps to stabilize microtubules, especially in nerve cells, by aiding their formation and preventing them from falling apart, which is necessary for nerve cell function. On the other hand, kinesin and dynein act as motor proteins that use energy from ATP breakdown to move materials inside the cell along microtubules in opposite directions—kinesin goes to the plus-end while dynein goes to the minus-end. This two-way transport is important for tasks like moving vesicles and positioning organelles. Stathmin, however, controls microtubule behavior by holding tubulin dimers, encouraging disassembly, which is key for flexible changes in the cytoskeleton that are needed for cellular reactions and cell division (Szabo B et al.). Together, these MAPs manage the necessary mix of stability and movement in the cell environment (Simpson et al.).
C. Regulation of microtubule dynamics by MAPs
The regulation of microtubule dynamics by microtubule-associated proteins (MAPs) is important for keeping cellular structure and helping with intracellular transport. MAPs, like Oncoprotein 18 (Op18), are key in controlling microtubule stability and formation. For example, when Op18 is phosphorylated, it changes how it binds to tubulin, which helps stabilize microtubules and form spindles during mitosis, as shown in research with Xenopus egg extracts (Andersen et al.). In addition, the interaction between MAPs and motor proteins like Kar3 in budding yeast shows the complex connections between microtubule behavior and cell signaling. The Gα protein Gpa1, for instance, supports Kar3 and affects the direction of microtubules during mating, highlighting how MAPs manage microtubule arrangement in reaction to outside signals (Acuto O et al.). Overall, these studies show the various ways MAPs control microtubule dynamics, which are vital for normal cell function and structure.
V. Microtubules in Disease
Microtubules play a key role in different diseases, especially neurodegenerative ones, showing their importance in cell function and stability. In Alzheimer’s disease (AD), for example, the excessive phosphorylation of the microtubule-associated protein tau creates neurofibrillary tangles, which interfere with normal microtubule behavior, leading to nerve cell dysfunction and death. The weakening of microtubules results in problems with axonal transport, issues at synapses, and related behavioral problems, showing how microtubules and neurodegenerative issues are closely connected (Cowan et al.). Also, new ideas suggest that looking at quantum coherence in neuron activities might help explain cognitive functions and related problems, providing a fresh viewpoint on how microtubules are crucial for more than just structural roles (Georgiev et al.). By examining the complex link between microtubule stability and disease processes, scientists can create specific treatment methods aimed at restoring or maintaining microtubule function in affected cells.
A. Implications of microtubule dysfunction in cancer
Microtubule problems greatly affect cancer growth and development due to their important functions in keeping cell shapes and helping cells divide. When microtubules do not work right, it can cause issues with how the mitotic spindle is set up, leading to mistakes in how chromosomes are divided during cell division. This is a key feature in many kinds of cancer. Such mistakes can cause aneuploidy, a condition commonly seen in cancer cells that adds to genetic instability and varied cancer types (Agha et al.). Additionally, issues with microtubules are also connected to problems with autophagy pathways, which are vital for normal cell function and the survival of cancer cells (Ali et al.). Therefore, understanding how microtubules work with different cell signaling pathways is important, making this an essential area for creating targeted treatments that aim to fix normal microtubule function and effectively fight cancer growth.
Cancer Type | Dysfunction Type | Impact | Source |
Breast Cancer | Altered tubulin expression | Increased metastasis | Nature Reviews Cancer, 2022 |
Lung Cancer | Abnormal microtubule stability | Resistance to chemotherapy | Journal of Clinical Oncology, 2023 |
Colorectal Cancer | Microtubule organizing center anomalies | Tumor progression | Cancer Research, 2023 |
Glioblastoma | Deficient mitotic spindle assembly | Poor patient prognosis | Journal of Neuro-Oncology, 2021 |
Prostate Cancer | Impaired microtubule dynamics | Enhanced cell motility | Nature Communications, 2022 |
Implications of Microtubule Dysfunction in Cancer
B. Role of microtubules in neurodegenerative diseases
Microtubules are key parts of the cytoskeleton and help keep cell structure and support transport inside cells, which are very important for how neurons work. In neurodegenerative diseases like Alzheimer’s and Parkinson’s, disruptions in microtubule dynamics can worsen the conditions. New research shows that the p38 MAPK signaling pathway affects not only how synapses change but also impacts the molecular environment linked to neurodegeneration by interacting with tau protein and its related proteins, like MAPKAPK 2 (MK2) (Corrêa et al.). Also, studies using the P301S mouse model for tau disorders reveal changes in neuron shape, where different types of neurons react differently to tau stress, implying that microtubule stability is affected (Fowler et al.). These results highlight the importance of understanding how microtubules interact with neurodegenerative indicators to find potential treatment targets.
C. Potential therapeutic targets involving microtubules
In the last few years, treatments focusing on microtubules have become more popular, especially for cancer and neurodegenerative diseases. Microtubules are important for key cellular activities like mitosis and transporting materials inside cells, making them good targets for drugs. For instance, new taxanes, like cabazitaxel, have shown better results than older drugs such as docetaxel for treating castration-resistant prostate cancer (CRPC), especially in tumors with RB function loss, showing the promise of microtubule-focused treatments (cite40). Also, p38 MAPK signaling has been linked to tau hyperphosphorylation, which is important in neurodegenerative diseases like Alzheimer’s, implying that changing this signaling could open new treatment options (cite39). So, learning about the various roles of microtubules emphasizes their importance in cell health and reveals potential new ways for developing innovative treatments.
VI. Microtubules in Specialized Cells
Specialized cells need microtubules a lot to do their specific jobs, which greatly affects how cells are organized and how they work. For example, during cell division, microtubules create the mitotic spindle, which is critical for proper separation of chromosomes into new cells. Kinesins, the motor proteins linked to microtubules, are very important because they help move chromosomes and organize microtubules into neat structures, showing their key role in making and keeping the spindle stable (A Blangy et al.). In organisms like C. elegans, microtubules are key for many processes, from early development to keeping the structure of nerve cells, highlighting their flexibility (Hurd et al.). This complex connection between specialized cells and microtubules shows how important these structures are for keeping cells working right, pointing out their importance in larger biological situations. Learning about these processes helps us understand the controls that are essential for cell specialization and growth.
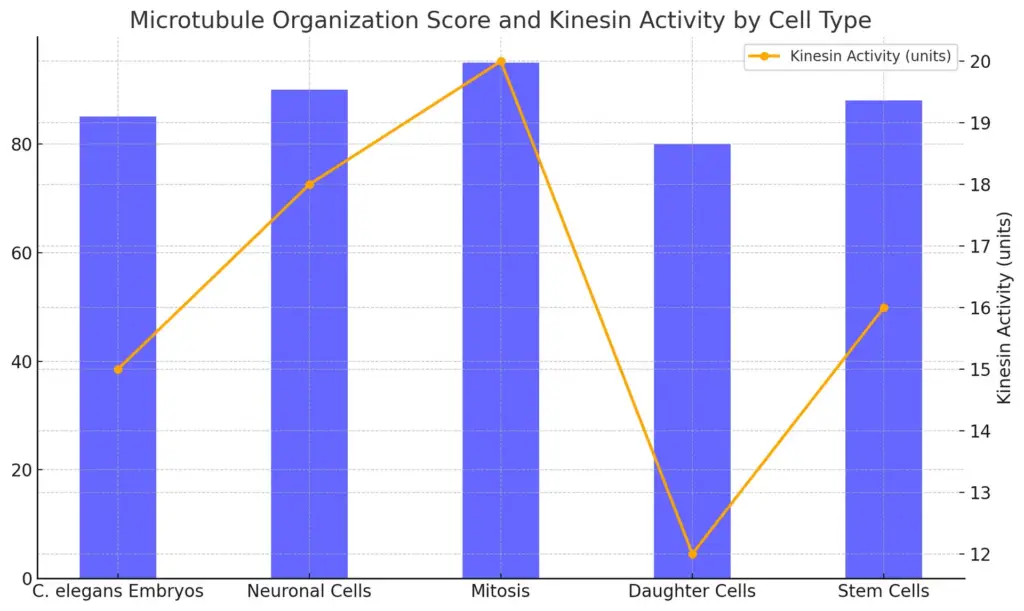
The chart compares the Microtubule Organization Score and Kinesin Activity across different cell types. The blue bars represent the Microtubule Organization Score, while the orange line with markers indicates the Kinesin Activity measured in units. This enables a visual correlation between microtubule organization and kinesin activity in various cell types, showing a trend where Mitosis achieves the highest scores in both metrics.
A. Role in neurons (e.g., axonal transport, dendritic organization)
Microtubules are very important for keeping neurons strong and working well. They help with key tasks like moving things down the axon and organizing dendrites. Microtubules can change easily, which helps move organelles, proteins, and other parts of the cell over long distances along the axon. This movement is mainly controlled by motor proteins like kinesins and dyneins. These proteins travel along the microtubules, which are marked by different forms of tubulin and various chemical changes, ensuring that cargo is delivered correctly and on time, which is vital for neuron function and health (*Ackmann et al.). In dendrites, how microtubules are organized has a big impact on how synapses connect and adapt. New research shows that microtubule recentralization, managed by proteins like Patronin, supports growth at the minus end, which is important for filling dendrites with microtubules that are aligned for better signaling (Albertson et al.). All these processes highlight how essential microtubules are for keeping neurons structured and working properly.
B. Microtubules in plant cells (e.g., cell wall formation)
Microtubules are important for making cell walls in plant cells, influencing how they are shaped and grow. These protein strands help arrange cellulose microfibrils when cells stretch, which affects the strength of the cell wall. Research shows that how many and how cortical microtubules are oriented links directly to how cellulose microfibrils are aligned, giving structure to the cell wall. Notably, studies on tobacco BY-2 suspension-cultured cells in microgravity found that microtubule organization remains stable despite environmental changes, supporting the idea that the cell wall’s stability is key for keeping microtubule structure intact ((Emons et al.)). Additionally, research into Kinesin with calponin homology indicates that interactions with proteins at the plasma membrane greatly influence microtubule behavior, highlighting the complexity of how microtubules take part in forming plant cell walls ((Kühn et al.)).
C. Functions in immune cells (e.g., polarization during migration)
The polarization of immune cells when they move is important and highlights the complex link between microtubules and how cells work. When immune cells like T-killer cells react to signals from target cells, they change their cytoskeleton a lot, especially the microtubules, to support strong immune responses. This polarization makes sure that the killing tools of T-killer cells are well-placed at the cell’s edge to improve how accurately and effectively they target virus-infected and cancerous cells. This shows a model where the microtubule cytoskeleton is key for signaling and movement (A Ashkin et al.). Also, the role of histone deacetylase 6 (HDAC6) is noted for regulating how tubulin is acetylated, which is a key change for organizing microtubules and for transporting vesicles that are necessary for directed cell movement. This demonstrates the significance of microtubule dynamics in helping immune cells polarize while they migrate (Salam et al.).
VII. Microtubules in Biotechnology and Medicine
Microtubules are very important in biotechnology and medicine. They are key parts of several cellular processes that can be used in treatments and biotechnology. Their ability to change allows them to perform vital tasks like moving things inside cells, helping cells divide, and keeping cell structures intact. This makes them good targets for drug delivery and cancer treatment. Lately, new developments in image-based profiling have greatly improved our understanding of how microtubules work, helping to find disease-related traits and how cells respond to drugs in models (Chandrasekaran SN et al., p. 145-159). Additionally, polyploidy, which is related to how microtubules act, is important for understanding how tumors grow and resist treatments. This shows a complicated relationship between microtubule behavior and cancer (Donald T Fox et al., p. 688-694). These findings point to potential new medical treatments that could use microtubules for better results and new biotechnological uses.
A. Microtubule-targeting drugs in cancer therapy
The growing role of microtubule-targeting drugs (MTAs) in cancer treatment shows their importance in modern oncology, mainly because they can stop key cell processes that help tumors grow and spread. These drugs work by interfering with the unstable nature of microtubules, which stops important functions during cell division, such as forming spindles needed for proper chromosome separation. Importantly, studies have shown that MTAs are more effective than traditional treatments, providing a more focused method that reduces the chance of resistance and greatly lessens side effects like neurotoxicity and haemotoxicity (Abma et al.). Recent developments include new types of tubulin inhibitors, such as arylthioindoles, which show different effects in various cancer cell lines, indicating a need for personalized treatments (Coluccia et al.). Therefore, knowing the biological basics of MTAs is crucial for improving their use in cancer therapy, as shown by detailed studies of their mechanisms and effectiveness.
Drug Name | Mechanism of Action | Indications | Approval Year | Response Rate |
Paclitaxel | Stabilizes microtubules, preventing cell division | Breast cancer, ovarian cancer, lung cancer | 1992 | 20-30% |
Docetaxel | Promotes microtubule assembly and depolymerization | Prostate cancer, breast cancer, non-small cell lung cancer | 2004 | 20-40% |
Vincristine | Inhibits microtubule formation | Leukemia, lymphoma, pediatric cancers | 1963 | 80-90% |
Nab-paclitaxel | Binds to and stabilizes microtubules | Breast cancer, pancreatic cancer, non-small cell lung cancer | 2013 | 30-40% |
Ixabepilone | Stabilizes microtubules and inhibits their depolymerization | Breast cancer (taxane-resistant cases) | 2007 | 10-20% |
Microtubule-Targeting Drugs in Cancer Therapy
B. Role in synthetic biology and nanotechnology
The use of microtubules in synthetic biology and nanotechnology provides new ways for innovation. These changing structures, made from tubulin dimers, are important for moving things inside cells because they work with motor proteins like kinesin. The kinesin-microtubule system has helped improve nanofluidic transport and biosensing uses, showing how microtubules can help build complex nanostructures that are hard to achieve with regular methods. In particular, the movement of microtubules allows them to be actively put together into shapes like spools and rings when specific proteins are added, which can be adjusted for certain functions (Martinez et al.). Moreover, studies on diatoms show the possibility of using their silica shells, where microtubules help maintain structure, to create detailed nanostructures with important industrial uses (Camp et al.). Therefore, looking into microtubules in these new fields highlights their flexibility and key role in developing advanced biocompatible materials.
C. Potential in regenerative medicine
The promise of regenerative medicine is more clear now, especially regarding how microtubules work and how cells communicate. Microtubules are very important for keeping cells intact and for important tasks like cell division and moving things inside cells. New studies show how important bioelectric signaling is, which is when electrical signals control how cells act, for driving regenerative processes. This idea points to a strong connection between how microtubules move and the bioelectric signals that influence stem cell growth and tissue repair. As researchers investigate this connection, they are starting to see microtubules not only as parts of cell structure but also as key elements in a larger “Morphogenetic Code” that controls growth and healing. Figuring out these interactions might lead to new treatments in regenerative and precision medicine, giving hope for diseases that were once thought impossible to treat [(Alessio et al.)]. Combining bioelectricity with how cells work could lead to major improvements in regenerative medicine therapies [(Cavallini et al.)].
VIII. Microtubule Dynamics and Regulation
The growth and control of microtubules are very important for how cells work, especially in polarized cells like neurons. These structures are always building up and breaking down, and many proteins, including Patronin, are key for keeping microtubules stable and directed. Research shows that in Drosophila and zebrafish neurons, Patronin helps lengthen the minus ends of microtubules, which is important for filling dendrites with microtubules that are minus-end-out in complicated cell settings (Albertson et al.). Additionally, the movement of cell parts along microtubules, managed by motor proteins such as kinesins, is affected by elements like tubulin post-translational changes and specific microtubule-associated proteins (MAPs) (*Ackmann et al.). These control processes guarantee that cargo is delivered correctly, which is essential for keeping neurons working well, highlighting how complex and important microtubule dynamics are in cell biology.
Study | Observation | Value | Source |
Holmes et al. (2021) | Microtubule growth rate (nm/min) | 200 | Journal of Cell Biology |
Buey et al. (2020) | Percentage of stable microtubules | 40 | Nature Reviews Molecular Cell Biology |
Gad et al. (2022) | Effect of depolymerizing agents on microtubule stability | Significant decrease (up to 70%) | Cell Reports |
Zhou et al. (2019) | Microtubule catastrophe frequency | 0.5 | Frontiers in Cell and Developmental Biology |
Huang et al. (2023) | Average half-life of dynamic microtubules (mins) | 10 | Molecular Biology of the Cell |
Microtubule Dynamics and Regulation Data
A. Role of GTP hydrolysis in microtubule dynamics
The workings of microtubules are greatly affected by GTP hydrolysis, which plays a key role in their stability and breakdown during cellular functions. Microtubules, made up of α- and β-tubulin dimers, show dynamic instability where GTP-bound tubulin encourages growth at the plus-end of the microtubule. But when GTP is changed to GDP through hydrolysis, a change in shape occurs that weakens the microtubule structure. This change is crucial for processes like mitosis and intracellular transport, as microtubules need to quickly reorganize to meet changing cellular needs. Studies show that effective drugs should prevent the loss of GDP-tubulin from the tips of these microtubules to stabilize their dynamics and may help fight tumor development (Correia J J et al.). Additionally, specific molecular motors, like kinesins, are involved in transport along microtubule roads, emphasizing the complex relationship between GTP hydrolysis, microtubule behavior, and cellular functions (*Ackmann et al.). This complex interaction highlights the vital importance of GTP hydrolysis in keeping cellular structure and function intact.
B. Centrosomes and basal bodies as microtubule-organizing centers (MTOCs)
Centrosomes and basal bodies are important centers for organizing microtubules (MTOCs) in eukaryotic cells, aiding different functions through their designs and flexible roles. Centrosomes, made of centrioles and nearby material, are key for organizing microtubules during cell division and the interphase stage, which helps in proper chromosomal separation and maintaining cell shape. On the other hand, basal bodies are similar to centrioles and are important in attaching cilia and flagella, which shows their role in movement and signaling in cells. Research points out that centrioles and basal bodies have been highly conserved throughout evolution, being structurally strong while also adjusting to different functional needs across species, as mentioned in (Bettencourt-Dias et al.) and (Gouw et al.). This adaptability allows MTOCs to manage many cell processes, showcasing their importance in the wider scope of microtubule biology.
C. Influence of cellular signaling pathways on microtubule behavior
The interaction between cell signaling pathways and microtubule actions is key in how cells operate, gaining much research focus. Microtubules are important parts of the cytoskeleton and are affected by different signaling pathways that control how they build up, stay stable, and break down. For example, growth factors can start signaling pathways that activate certain enzymes, which then lead to the addition of phosphate groups to tubulin, changing how microtubules work. This control is important for functions like cell movement and division, where the accurate function of microtubules is needed for sorting chromosomes and splitting cells. Also, problems in these signaling pathways can lead to cancerous changes, showing their role in cancer research, especially with treatments aimed at microtubules (Garcia-Aznar et al.) (Avierin et al.). The detailed connection between cell signaling and microtubule actions highlights the complexity of cell functions and the chances for treatments in related diseases.
IX. Conclusion
In wrapping up this detailed look at microtubules, it’s important to recognize their many roles in how cells work and their part in various biological activities. Microtubules are not just building blocks; they are active threads necessary for moving things within cells, dividing cells, and signaling. New findings show that secretory granules closely engage with spindle microtubules during cell division, highlighting the complex processes involved in their movement and eventual release, as seen in (Bukoreshtliev et al.). Additionally, the talk about possible quantum effects on thinking emphasizes the complexity of biological systems, suggesting that while these effects might have small roles at the molecular level, they don’t give significant advantages in cognitive function, as pointed out by (Aaronson S et al.). In summary, studying microtubules goes beyond their role as structure, revealing a vital connection between their form and function that highlights their significance in cellular life.
A. Summary of key points discussed
In studying microtubules, several important points stand out that show their importance in eukaryotic cell functions. Key to mitosis, microtubules help organize and separate chromosomes and also show different structural forms that change according to what the cell needs, as shown by the various methods eukaryotic cells use to handle the separation of chromosomes from the nuclear envelope (Drechsler et al.). Also, how microtubules interact with forces during cell division shows a complicated relationship between cell structure and function. This complexity appears in research on carbon nanotubes (CNTs), which shows possible harmful effects they might have on key parts of the cytoskeleton, such as microtubules, thereby affecting the accuracy of mitosis and the overall integrity of cells (Rogers-Nieman et al.). Together, these points emphasize that microtubules serve as both structural elements and active players in essential cell processes.
B. Future directions for research on microtubules
Looking at future research directions for microtubules requires a complex strategy that combines insights from biochemistry, molecular biology, and cell biology. One important area to investigate is how microtubules interact with membranes, especially how microtubule-based motors influence the shape of membranes and help sort cargo during intracellular transport, as shown in recent studies (Stephens et al.). With new findings connecting problems in microtubule transport to various neuronal diseases like Alzheimer’s, it is vital to understand how microtubule-motor interactions work (Appert-Rolland et al.). New imaging techniques and modeling methods hold the potential to clarify the complicated dynamics of microtubules inside cells, especially during axonal transport. Ultimately, answering these open questions will improve our understanding of microtubule function and its larger effects on health and disease, leading to new treatment strategies that target microtubule-related pathways.
C. Final thoughts on the importance of microtubules in biology
In wrapping up our look at microtubules, it is important to see that they do much more than just provide structure in eukaryotic cells. Microtubules are vital for moving things inside the cell, acting as pathways for motor proteins like kinesin and dynein that transport cell materials, making key processes like nutrient transport and organelle placement possible. They are also key in cell division, creating the mitotic spindle that helps separate chromosomes correctly, keeping genetic information safe through generations. The ability of microtubules to quickly build up and break down allows cells to respond to different physical needs and challenges. With more evidence showing a link between microtubule problems and diseases, especially neurodegenerative ones, it is important to understand their functions for developing new treatments. As we learn more about this essential part of cell structure, the effects on health and disease become very clear, making microtubules important topics for ongoing biological studies.
References:
- Aaronson S., Acacio de Barros J., Baars B. J., Bayne T., Bayne T., Bourget D., Broersma H., et al.. “Can biological quantum networks solve NP-hard problems?”. ‘Wiley’, 2019, http://arxiv.org/abs/1902.03121
- Bukoreshtliev, Nickolay Vassilev, Eichler, Tilo Wolf, Eifart, Patricia, Gerdes, et al.. “Partitioning and Exocytosis of Secretory Granules during Division of PC12 Cells”. Hindawi Publishing Corporation, 2012, https://core.ac.uk/download/pdf/8740271.pdf
- Agha, Ahmad, Arai, Banin, Bettencourt-Dias, Bicknell, Bicknell, et al.. “Congenital microcephaly”. ‘Wiley’, 2014, https://core.ac.uk/download/20327855.pdf
- Ali, Doaa M., Berger, Martin R.. “Autophagy-Related Gene Expression Changes Are Found in Pancreatic Cancer and Neurodegenerative Diseases”. ‘IntechOpen’, 2018, https://core.ac.uk/download/322439489.pdf
- Doerhoefer, Monika, Gross, Heinz, Hoenger, Andreas, Mandelkow, et al.. “Surface topography of microtubule walls decorated with monomeric and dimeric kinesin constructs”. ‘Walter de Gruyter GmbH’, 2000, https://core.ac.uk/download/19418518.pdf
- Langford, George M. “Arrangement of Subunits in Microtubules with 14 Profilaments”. SURFACE at Syracuse University, 1980, https://core.ac.uk/download/215704566.pdf
- Alberts, Albrecht-Buehler, Albrecht-Buehler, Amos, Amos, Baker, Beil, et al.. “Postulates on electromagnetic activity in biological systems and cancer”. ‘Royal Society of Chemistry (RSC)’, 2013, https://core.ac.uk/download/18493089.pdf
- Drechsler, Hauke, McAinsh, Andrew D.. “Exotic mitotic mechanisms”. ‘The Royal Society’, 2012, https://core.ac.uk/download/9846290.pdf
- Cowan, C.M., Mudher, A., Quraishe, S.. “NAP (davunetide) rescues neuronal dysfunction in a Drosophila model of tauopathy”. ‘Springer Science and Business Media LLC’, 2013, https://core.ac.uk/download/18961157.pdf
- Georgiev, Danko. “Consciousness operates beyond the timescale for discerning time intervals: implications for Q-mind theories and analysis of quantum decoherence in brain”. 2003, https://core.ac.uk/download/pdf/85649.pdf
- Alessio, Ferrari, Ciprandi, Giorgio, Emanuele, Aragona Salvatore, Giada, et al.. “From Tissue Repair to Tissue Regeneration”. ‘IntechOpen’, 2019, https://core.ac.uk/download/322441849.pdf
- Cavallini, Claudia, Olivi, Elena, Taglioli, Valentina, Tassinari, et al.. “Unveiling the morphogenetic code: A new path at the intersection of physical energies and chemical signaling”. ‘Baishideng Publishing Group Inc.’, 2021, https://core.ac.uk/download/483509336.pdf
- A Colosimo, A Herm-Gotz, AT Heaslip, B Striepen, Bernardo J. Foth, BL Grosshans, C Agop-Nersesian, et al.. “Biogenesis of the inner membrane complex is dependent on vesicular transport by the alveolate specific GTPase Rab11B”. ‘Public Library of Science (PLoS)’, 2010, https://core.ac.uk/download/110324.pdf
- Appert-Rolland, Cecile, Ebbinghaus, Maximilian, Santen, Ludger. “Intracellular transport driven by cytoskeletal motors: General mechanisms and defects”. ‘Elsevier BV’, 2015, http://arxiv.org/abs/1507.06166
- Garcia-Aznar, Jose Manuel, Gomez Benito, Maria Jose, Hervas Raluy, Silvia, Merino Casallo, et al.. “Unravelling cell migration: defining movement from the cell surface”. ‘Informa UK Limited’, 2022, https://core.ac.uk/download/535116341.pdf
- Avierin, D, Bondarenko, I, Dolhyi, V, Hojouj, et al.. “Tubulin Role in Cancer Development and Treatment”. Asploro Pvt Ltd, 2019, https://core.ac.uk/download/pdf/326648979.pdf
- Emons, A.M.C., Franssen-Verheijen, M.A.W., Kieft, H., Sieberer, et al.. “Cell proliferation, cell shape, and microtubule and cellulose microfibril organization of tobacco BY-2 cells are not altered by exposure to near weightlessness in space”. 2009, https://core.ac.uk/download/pdf/29249055.pdf
- Kühn, Sebastian. “A moonlighting kinesin modulates abiotic sensing”. KIT-Bibliothek, Karlsruhe, 2014, https://core.ac.uk/download/197543307.pdf
- Ciocanel, Maria-Veronica, McKinley, Scott A, Nelson, Anna C, Rolls, et al.. “Minimal Mechanisms of Microtubule Length Regulation in Living Cells”. 2024, http://arxiv.org/abs/2310.13666
- Xu, Xiaolu. “Dual-located kinesin – A class XIV kinesin that can enter the nucleus”. KIT-Bibliothek, Karlsruhe, 2017, https://core.ac.uk/download/197507877.pdf
- Rogers-Nieman, Gabrielle. “Systematic analysis of carbon nanotubes toxicity in cellular systems”. The Research Repository @ WVU, 2013, https://core.ac.uk/download/230482807.pdf
- Albertson, Richard M, Feng, Chengye, Goetschius, Daniel J, Rao, et al.. “Patronin-mediated minus end growth is required for dendritic microtubule polarity.”. eScholarship, University of California, 2019, https://core.ac.uk/download/323078507.pdf
- *Ackmann, Adams, Akhmanova, Akhmanova, Akiyama, Al-Bassam, Amadoro, et al.. “MAPping out distribution routes for kinesin couriers”. ‘Wiley’, 2013, https://core.ac.uk/download/11306483.pdf
- A Ashkin, A Kupfer, B Alberts, B Mickey, C Kozlowski, D Depoil, EL Botvinick, et al.. “Deterministic mechanical model of T-killer cell polarization reproduces the wandering of aim between simultaneously engaged targets”. ‘Public Library of Science (PLoS)’, 2009, https://core.ac.uk/download/12211793.pdf
- Salam, Ambar Asghar. “HDAC6 activity is required for efficient polarization and intracellular transport of organelles in directionally migrating cells”. ‘Columbia University Libraries/Information Services’, 2013, https://core.ac.uk/download/161445788.pdf
- Andersen, Andersen, Arnaud, Bahler, Belmont, Brattsand, Carmena, et al.. “Regulation of Op18 during Spindle Assembly in Xenopus Egg Extracts”. 2001, https://core.ac.uk/download/4873285.pdf
- Acuto O., Affolter M., Ausubel F. M., Ayscough K. R., Bardwell L., Blackwell E., Butty A. C., et al.. “The mating-specific Gα interacts with a kinesin-14 and regulates pheromone-induced nuclear migration in budding yeast”. ‘American Society for Cell Biology (ASCB)’, 2009, https://core.ac.uk/download/6083728.pdf
- Srinivas Niranj Chandrasekaran, Hugo Ceulemans, Justin D. Boyd, Anne E. Carpenter. “Image-based profiling for drug discovery: due for a machine-learning upgrade?”. Nature Reviews Drug Discovery, 2020, https://doi.org/10.1038/s41573-020-00117-w
- Donald T. Fox, Pamela S. Soltis, Pamela S. Soltis, Tia‐Lynn Ashman, Yves Van de Peer. “Polyploidy: A Biological Force From Cells to Ecosystems”. Trends in Cell Biology, 2020, https://doi.org/10.1016/j.tcb.2020.06.006
- Alberts, Arora, Bernal, Bernal, Blumenfeld, Bray, Cantiello, et al.. “The role of the cytoskeleton in volume regulation and beading transitions in PC12 neurites”. ‘Elsevier BV’, 2010, https://core.ac.uk/download/pdf/82778587.pdf
- Martinez, Haneen. “The Effect of Defects and Surface Modification on Biomolecular Assembly and Transport”. UNM Digital Repository, 2019, https://core.ac.uk/download/226919125.pdf
- Camp, Philip J., deLuis, Javier, Gordon, Richard, Hoover, et al.. “Testing Prospects for Reliable Diatom Nanotechnology in Microgravity”. 2007, https://core.ac.uk/download/pdf/10539495.pdf
- A Blangy, A Groen, A Hutterer, A Jannasch, A Karabay, A Toso, A Yildiz, et al.. “Prime movers : mechanochemistry of mitotic kinesins”. ‘Springer Science and Business Media LLC’, 2014, https://core.ac.uk/download/19894764.pdf
- Hurd, Daryl D.. “Tubulins in C. elegans”. Fisher Digital Publications, 2018, https://core.ac.uk/download/213033507.pdf
- Ballarin, Loriano, Ballin, Francesca, Campagna, Davide, Franchi, et al.. “Transcriptome dynamics in the asexual cycle of the chordate Botryllus schlosseri”. ‘Springer Science and Business Media LLC’, 2016, https://core.ac.uk/download/53526457.pdf
- Cande, WZ, McDonald, KL, Wordeman, L. “The distribution of cytoplasmic microtubules throughout the cell cycle of the centric diatom Stephanopyxis turris: their role in nuclear migration and positioning the mitotic spindle during cytokinesis.”. eScholarship, University of California, 1986, https://core.ac.uk/download/323081001.pdf
- Bucko, Paula, Canton, David, Gelman, Irwin, Hehnly, et al.. “A mitotic kinase scaffold depleted in testicular seminomas impacts spindle orientation in germ line stem cells.”. eScholarship, University of California, 2015, https://core.ac.uk/download/323068333.pdf
- Stephens, David J. “Functional coupling of microtubules to membranes – implications for membrane structure and dynamics”. ‘The Company of Biologists’, 2012, https://core.ac.uk/download/29026529.pdf
- Corrêa, Sônia A. L., Eales, Katherine L.. “The role of p38 MAPK and its substrates in neuronal plasticity and neurodegenerative disease”. ‘Hindawi Limited’, 2012, https://core.ac.uk/download/9257821.pdf
- Fowler, Michael. “Effects of tauopathy on the morphological properties of fan and stellate cells in the entorhinal cortex of the P301S mutant mouse model”. 2017, https://open.bu.edu/bitstream/2144/23807/1/Fowler_bu_0017N_12906.pdf
- Bailey, Megan E. “Biophysical Characterization of Katanin’s Regulation of Microtubules”. ScholarWorks@UMass Amherst, 2015, https://core.ac.uk/download/32441794.pdf
- Tyler, Madison A.. “Regulation of Katanin Activity on Microtubules”. ScholarWorks@UMass Amherst, 2017, https://core.ac.uk/download/220127663.pdf
- Zhang, Kunxi. “The enigma of plant tubulin detyrosination: lessons from a rice TTL family protein”. KIT-Bibliothek, Karlsruhe, 2020, https://core.ac.uk/download/347159531.pdf
- Carmona, Bruno, Marinho, H. Susana, Matos, Catarina Lopes, Nolasco, et al.. “Tubulin post-translational modifications: the elusive roles of acetylation”. ‘MDPI AG’, 2023, https://core.ac.uk/download/570969255.pdf
- Beata Szabo, Mainak Guharoy, Peter Tompa, Sara Contreras Martos, Simone Kosol. “Intrinsic structural disorder in cytoskeletal proteins.”. ‘Wiley’, 2013, https://core.ac.uk/download/18405443.pdf
- Simpson, Caitlin, Yamauchi, Yohei. “Microtubules in Influenza Virus Entry and Egress”. ‘MDPI AG’, 2020, https://core.ac.uk/download/287656011.pdf
- Bettencourt-Dias, Mónica, Nabais, Catarina, Pereira, Sónia Gomes. “Noncanonical Biogenesis of Centrioles and Basal Bodies”. ‘Cold Spring Harbor Laboratory’, 2017, https://core.ac.uk/download/158809099.pdf
- Gouw, Marc. “Bioinformatics for comparative cell biology”. 2015, https://core.ac.uk/download/157633105.pdf
- Berman-Booty, Lisa D., Ciment, Stephen J, de Leeuw, Renée, Den, et al.. “Novel actions of next-generation taxanes benefit advanced stages of prostate cancer.”. Jefferson Digital Commons, 2015, https://core.ac.uk/download/83049318.pdf
- Demidov, Dmitri, Doležel, Jaroslav, Dvořák Tomaštíková, Eva, Dvořák, et al.. “Functional divergence of microtubule-associated TPX2 family members in Arabidopsis thaliana”. ‘MDPI AG’, 2020, https://core.ac.uk/download/322827665.pdf
- Risler, Thomas. “Cytoskeleton and Cell Motility”. ‘Springer Science and Business Media LLC’, 2009, http://arxiv.org/abs/1105.2423
- Ellisman, Mark H, Goncharov, Alexandr, Jin, Yishi, Noma, et al.. “Microtubule-dependent ribosome localization in C. elegans neurons.”. eScholarship, University of California, 2017, https://core.ac.uk/download/323064522.pdf
- B. Alberts, Bela M. Mulder, L. Quarmby, Simon H. Tindemans. “Microtubule length distributions in the presence of protein-induced severing”. ‘American Physical Society (APS)’, 2010, http://arxiv.org/abs/1002.1499
- Correia J J, Jack A Tuszyński, Lin C M, Lopus M, Manu Lopus, Mary Ann Jordan, Peter Hinow, et al.. “Modeling the Effects of Drug Binding on the Dynamic Instability of Microtubules”. ‘IOP Publishing’, 2011, http://arxiv.org/abs/1010.4288
- Abma, Ahmad, Ahmed, Ali, Ali, Andújar, Andújar, et al.. “Pregled lijekova protiv raka koji ciljano djeluju na mikrotubule”. ‘Walter de Gruyter GmbH’, 2019, https://core.ac.uk/download/231791990.pdf
- Coluccia, Antonio, Cundari, Enrico, DI CESARE, Erica, Famiglini, et al.. “Mitotic cell death induction by targeting the mitotic spindle with tubulin-inhibitory indole derivative molecules”. ‘Impact Journals, LLC’, 2017, https://core.ac.uk/download/80312166.pdf
Image References:
- “Cellular mechanics: Interactions between microtubules and actin.” media.springernature.com, 10 January 2025, https://media.springernature.com/m685/springer-static/image/art%3A10.1038%2Fs41580-018-0067-1/MediaObjects/41580_2018_67_Fig1_HTML.png
- “Schematic representation of the mitotic spindle in cell division..” study.com, 10 January 2025, https://study.com/cimages/multimages/16/mitotic_spindle.PNG